Physicists clock the fastest possible speed of sound
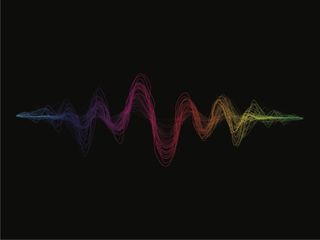
Scientists have discovered the fastest possible speed of sound, a zippy 22 miles (36 kilometers) per second.
Sound waves move at different speeds in solids , liquids and gases , and within those states of matter — for instance, they travel faster in warmer liquids compared with colder ones. Physicist Kostya Trachenko of Queen Mary University of London and his colleagues wanted to figure out the upper limits of how fast sound could travel.
This exercise was largely theoretical: The researchers found that the answer, which is about twice as fast as sound moves through solid diamond, depends on some fundamental numbers in the universe. The first is the fine structure constant, which is a number that describes the electromagnetic force that holds together elementary particles such as electrons and protons. (It happens to be approximately 1/137.) The second is the proton-to-electron mass ratio of a material, which, as it sounds, is the ratio of mass from protons and mass from electrons within the atomic structure of the material.
Related: In photos: Large numbers that define the universe
It's not possible to test this theoretical top speed in the real world, because the math predicts that sound moves at its top speed in the lowest-mass atoms . The lowest-mass atom is hydrogen, but hydrogen isn't solid —— unless it's under super-duper pressure that's a million times stronger than that of Earth's atmosphere. That might happen at the core of a gas giant like Jupiter, but it doesn't happen anywhere nearby where scientific testing is possible.
So instead, Trachenko and his colleagues turned to quantum mechanics and math to calculate what would happen to sound zipping through a solid atom of hydrogen . They found that sound could travel close to the theoretical limit of 79,200 mph (127,460 km/h), confirming their initial calculations. In contrast, the speed of sound in air is roughly 767 mph (1,235 km/h).
The movement of sound in such extreme and specific environments may seem unimportant, but because sound waves are traveling vibrations of molecules, the speed of sound is related to many other properties of materials, such as the ability to resist stress, study co-author Chris Pickard, a materials scientist at the University of Cambridge, said in a statement . Thus, understanding the fundamentals of sound could help illuminate other fundamental properties of materials in extreme circumstances, Trachenko added in the statement.
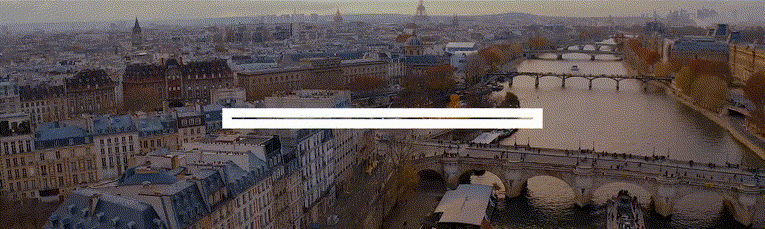
Sign up for the Live Science daily newsletter now
Get the world’s most fascinating discoveries delivered straight to your inbox.
— The 18 biggest unsolved mysteries in physics
— From Big Bang to present: Snapshots of our universe through time
— The mysterious physics of 7 everyday things
For instance, previous research has suggested that solid atomic hydrogen could be a superconductor. So knowing its fundamental properties could be important for future superconductivity research. Sound could also reveal more about the hot mix of quarks and gluons that made up the universe an instant after the Big Bang, and could be applied to the strange physics around the gravity wells that are black holes. (Other researchers have studied " sonic black holes " to gather insight into these cosmic objects.)
"We believe the findings of this study could have further scientific applications by helping us to find and understand limits of different properties, such as viscosity and thermal conductivity, relevant for high-temperature superconductivity, quark-gluon plasma, and even black hole physics," Trachenko said.
The researchers reported their findings Oct. 9 in the journal Science Advances .
Originally published on Live Science.
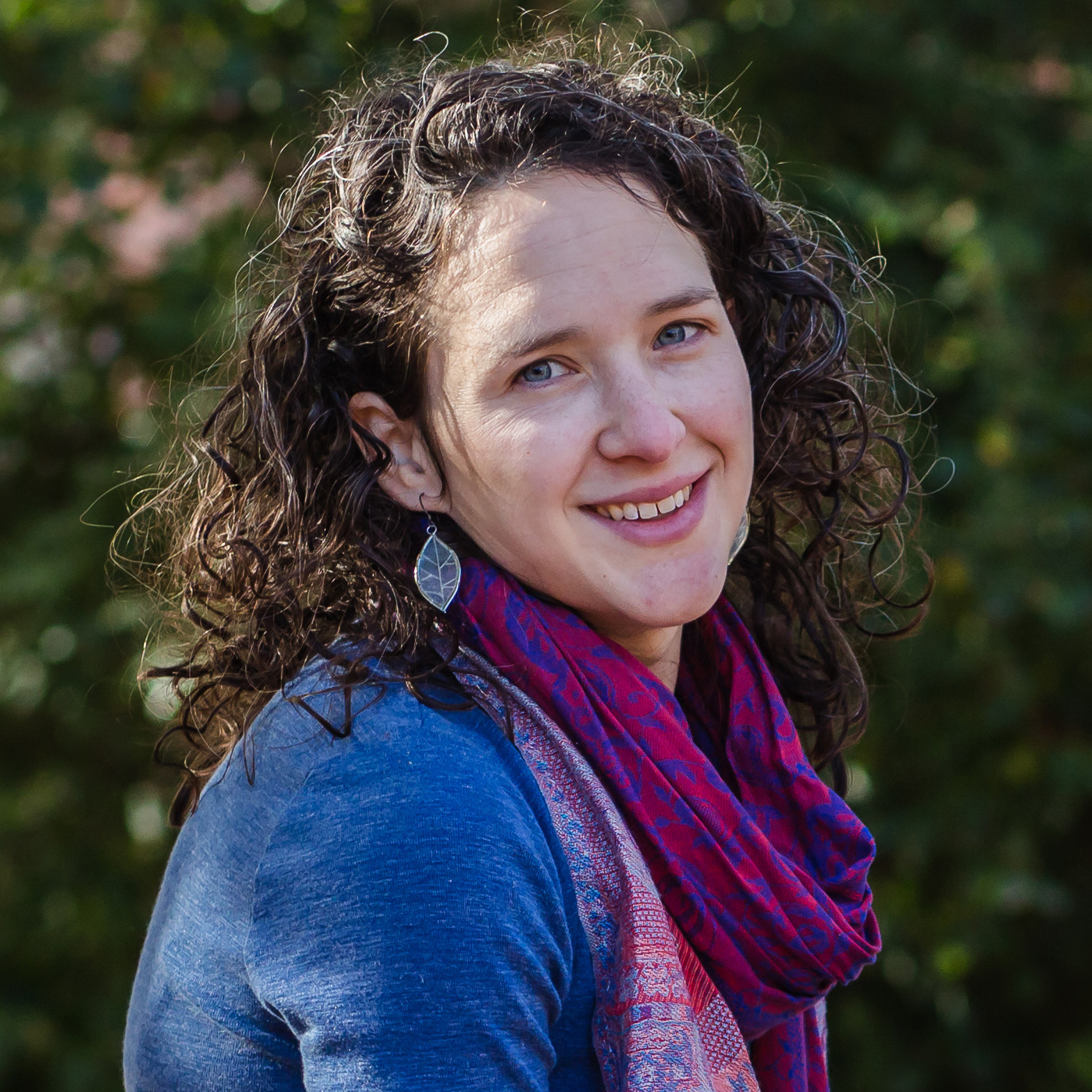
Stephanie Pappas is a contributing writer for Live Science, covering topics ranging from geoscience to archaeology to the human brain and behavior. She was previously a senior writer for Live Science but is now a freelancer based in Denver, Colorado, and regularly contributes to Scientific American and The Monitor, the monthly magazine of the American Psychological Association. Stephanie received a bachelor's degree in psychology from the University of South Carolina and a graduate certificate in science communication from the University of California, Santa Cruz.
Scientists are one step closer to knowing the mass of ghostly neutrinos — possibly paving the way to new physics
The universe may be dominated by particles that break causality and move faster than light, new paper suggests
Tweak to Schrödinger's cat equation could unite Einstein's relativity and quantum mechanics, study hints
Most Popular
- 2 Haunting photo of Earth and moon snapped by China's experimental lunar satellites
- 3 Dying SpaceX rocket tears blood-red 'hole' in the sky over Texas — again
- 4 Global 'time signals' subtly shifted as the total solar eclipse reshaped Earth's upper atmosphere, new data shows
- 5 Rare 'porcelain gallbladder' found in 100-year-old unmarked grave at Mississippi mental asylum cemetery
- 2 China green-lights mass production of autonomous flying taxis — with commercial flights set for 2025
- 3 George Washington's stash of centuries-old cherries found hidden under Mount Vernon floor
- 4 Haunting photo of Earth and moon snapped by China's experimental lunar satellites
- 5 5 catastrophic megathrust earthquakes led to the demise of the pre-Aztec city of Teotihuacan, new study suggests
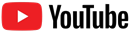
- TPC and eLearning
- Read Watch Interact
- What's NEW at TPC?
- Practice Review Test
- Teacher-Tools
- Subscription Selection
- Seat Calculator
- Ad Free Account
- Edit Profile Settings
- Classes (Version 2)
- Student Progress Edit
- Task Properties
- Export Student Progress
- Task, Activities, and Scores
- Metric Conversions Questions
- Metric System Questions
- Metric Estimation Questions
- Significant Digits Questions
- Proportional Reasoning
- Acceleration
- Distance-Displacement
- Dots and Graphs
- Graph That Motion
- Match That Graph
- Name That Motion
- Motion Diagrams
- Pos'n Time Graphs Numerical
- Pos'n Time Graphs Conceptual
- Up And Down - Questions
- Balanced vs. Unbalanced Forces
- Change of State
- Force and Motion
- Mass and Weight
- Match That Free-Body Diagram
- Net Force (and Acceleration) Ranking Tasks
- Newton's Second Law
- Normal Force Card Sort
- Recognizing Forces
- Air Resistance and Skydiving
- Solve It! with Newton's Second Law
- Which One Doesn't Belong?
- Component Addition Questions
- Head-to-Tail Vector Addition
- Projectile Mathematics
- Trajectory - Angle Launched Projectiles
- Trajectory - Horizontally Launched Projectiles
- Vector Addition
- Vector Direction
- Which One Doesn't Belong? Projectile Motion
- Forces in 2-Dimensions
- Being Impulsive About Momentum
- Explosions - Law Breakers
- Hit and Stick Collisions - Law Breakers
- Case Studies: Impulse and Force
- Impulse-Momentum Change Table
- Keeping Track of Momentum - Hit and Stick
- Keeping Track of Momentum - Hit and Bounce
- What's Up (and Down) with KE and PE?
- Energy Conservation Questions
- Energy Dissipation Questions
- Energy Ranking Tasks
- LOL Charts (a.k.a., Energy Bar Charts)
- Match That Bar Chart
- Words and Charts Questions
- Name That Energy
- Stepping Up with PE and KE Questions
- Case Studies - Circular Motion
- Circular Logic
- Forces and Free-Body Diagrams in Circular Motion
- Gravitational Field Strength
- Universal Gravitation
- Angular Position and Displacement
- Linear and Angular Velocity
- Angular Acceleration
- Rotational Inertia
- Balanced vs. Unbalanced Torques
- Getting a Handle on Torque
- Torque-ing About Rotation
- Properties of Matter
- Fluid Pressure
- Buoyant Force
- Sinking, Floating, and Hanging
- Pascal's Principle
- Flow Velocity
- Bernoulli's Principle
- Balloon Interactions
- Charge and Charging
- Charge Interactions
- Charging by Induction
- Conductors and Insulators
- Coulombs Law
- Electric Field
- Electric Field Intensity
- Polarization
- Case Studies: Electric Power
- Know Your Potential
- Light Bulb Anatomy
- I = ∆V/R Equations as a Guide to Thinking
- Parallel Circuits - ∆V = I•R Calculations
- Resistance Ranking Tasks
- Series Circuits - ∆V = I•R Calculations
- Series vs. Parallel Circuits
- Equivalent Resistance
- Period and Frequency of a Pendulum
- Pendulum Motion: Velocity and Force
- Energy of a Pendulum
- Period and Frequency of a Mass on a Spring
- Horizontal Springs: Velocity and Force
- Vertical Springs: Velocity and Force
- Energy of a Mass on a Spring
- Decibel Scale
- Frequency and Period
- Closed-End Air Columns
- Name That Harmonic: Strings
- Rocking the Boat
- Wave Basics
- Matching Pairs: Wave Characteristics
- Wave Interference
- Waves - Case Studies
- Color Addition and Subtraction
- Color Filters
- If This, Then That: Color Subtraction
- Light Intensity
- Color Pigments
- Converging Lenses
- Curved Mirror Images
- Law of Reflection
- Refraction and Lenses
- Total Internal Reflection
- Who Can See Who?
- Formulas and Atom Counting
- Atomic Models
- Bond Polarity
- Entropy Questions
- Cell Voltage Questions
- Heat of Formation Questions
- Reduction Potential Questions
- Oxidation States Questions
- Measuring the Quantity of Heat
- Hess's Law
- Oxidation-Reduction Questions
- Galvanic Cells Questions
- Thermal Stoichiometry
- Molecular Polarity
- Quantum Mechanics
- Balancing Chemical Equations
- Bronsted-Lowry Model of Acids and Bases
- Classification of Matter
- Collision Model of Reaction Rates
- Density Ranking Tasks
- Dissociation Reactions
- Complete Electron Configurations
- Elemental Measures
- Enthalpy Change Questions
- Equilibrium Concept
- Equilibrium Constant Expression
- Equilibrium Calculations - Questions
- Equilibrium ICE Table
- Intermolecular Forces Questions
- Ionic Bonding
- Lewis Electron Dot Structures
- Limiting Reactants
- Line Spectra Questions
- Mass Stoichiometry
- Measurement and Numbers
- Metals, Nonmetals, and Metalloids
- Metric Estimations
- Metric System
- Molarity Ranking Tasks
- Mole Conversions
- Name That Element
- Names to Formulas
- Names to Formulas 2
- Nuclear Decay
- Particles, Words, and Formulas
- Periodic Trends
- Precipitation Reactions and Net Ionic Equations
- Pressure Concepts
- Pressure-Temperature Gas Law
- Pressure-Volume Gas Law
- Chemical Reaction Types
- Significant Digits and Measurement
- States Of Matter Exercise
- Stoichiometry Law Breakers
- Stoichiometry - Math Relationships
- Subatomic Particles
- Spontaneity and Driving Forces
- Gibbs Free Energy
- Volume-Temperature Gas Law
- Acid-Base Properties
- Energy and Chemical Reactions
- Chemical and Physical Properties
- Valence Shell Electron Pair Repulsion Theory
- Writing Balanced Chemical Equations
- Mission CG1
- Mission CG10
- Mission CG2
- Mission CG3
- Mission CG4
- Mission CG5
- Mission CG6
- Mission CG7
- Mission CG8
- Mission CG9
- Mission EC1
- Mission EC10
- Mission EC11
- Mission EC12
- Mission EC2
- Mission EC3
- Mission EC4
- Mission EC5
- Mission EC6
- Mission EC7
- Mission EC8
- Mission EC9
- Mission RL1
- Mission RL2
- Mission RL3
- Mission RL4
- Mission RL5
- Mission RL6
- Mission KG7
- Mission RL8
- Mission KG9
- Mission RL10
- Mission RL11
- Mission RM1
- Mission RM2
- Mission RM3
- Mission RM4
- Mission RM5
- Mission RM6
- Mission RM8
- Mission RM10
- Mission LC1
- Mission RM11
- Mission LC2
- Mission LC3
- Mission LC4
- Mission LC5
- Mission LC6
- Mission LC8
- Mission SM1
- Mission SM2
- Mission SM3
- Mission SM4
- Mission SM5
- Mission SM6
- Mission SM8
- Mission SM10
- Mission KG10
- Mission SM11
- Mission KG2
- Mission KG3
- Mission KG4
- Mission KG5
- Mission KG6
- Mission KG8
- Mission KG11
- Mission F2D1
- Mission F2D2
- Mission F2D3
- Mission F2D4
- Mission F2D5
- Mission F2D6
- Mission KC1
- Mission KC2
- Mission KC3
- Mission KC4
- Mission KC5
- Mission KC6
- Mission KC7
- Mission KC8
- Mission AAA
- Mission SM9
- Mission LC7
- Mission LC9
- Mission NL1
- Mission NL2
- Mission NL3
- Mission NL4
- Mission NL5
- Mission NL6
- Mission NL7
- Mission NL8
- Mission NL9
- Mission NL10
- Mission NL11
- Mission NL12
- Mission MC1
- Mission MC10
- Mission MC2
- Mission MC3
- Mission MC4
- Mission MC5
- Mission MC6
- Mission MC7
- Mission MC8
- Mission MC9
- Mission RM7
- Mission RM9
- Mission RL7
- Mission RL9
- Mission SM7
- Mission SE1
- Mission SE10
- Mission SE11
- Mission SE12
- Mission SE2
- Mission SE3
- Mission SE4
- Mission SE5
- Mission SE6
- Mission SE7
- Mission SE8
- Mission SE9
- Mission VP1
- Mission VP10
- Mission VP2
- Mission VP3
- Mission VP4
- Mission VP5
- Mission VP6
- Mission VP7
- Mission VP8
- Mission VP9
- Mission WM1
- Mission WM2
- Mission WM3
- Mission WM4
- Mission WM5
- Mission WM6
- Mission WM7
- Mission WM8
- Mission WE1
- Mission WE10
- Mission WE2
- Mission WE3
- Mission WE4
- Mission WE5
- Mission WE6
- Mission WE7
- Mission WE8
- Mission WE9
- Vector Walk Interactive
- Name That Motion Interactive
- Kinematic Graphing 1 Concept Checker
- Kinematic Graphing 2 Concept Checker
- Graph That Motion Interactive
- Two Stage Rocket Interactive
- Rocket Sled Concept Checker
- Force Concept Checker
- Free-Body Diagrams Concept Checker
- Free-Body Diagrams The Sequel Concept Checker
- Skydiving Concept Checker
- Elevator Ride Concept Checker
- Vector Addition Concept Checker
- Vector Walk in Two Dimensions Interactive
- Name That Vector Interactive
- River Boat Simulator Concept Checker
- Projectile Simulator 2 Concept Checker
- Projectile Simulator 3 Concept Checker
- Hit the Target Interactive
- Turd the Target 1 Interactive
- Turd the Target 2 Interactive
- Balance It Interactive
- Go For The Gold Interactive
- Egg Drop Concept Checker
- Fish Catch Concept Checker
- Exploding Carts Concept Checker
- Collision Carts - Inelastic Collisions Concept Checker
- Its All Uphill Concept Checker
- Stopping Distance Concept Checker
- Chart That Motion Interactive
- Roller Coaster Model Concept Checker
- Uniform Circular Motion Concept Checker
- Horizontal Circle Simulation Concept Checker
- Vertical Circle Simulation Concept Checker
- Race Track Concept Checker
- Gravitational Fields Concept Checker
- Orbital Motion Concept Checker
- Angular Acceleration Concept Checker
- Balance Beam Concept Checker
- Torque Balancer Concept Checker
- Aluminum Can Polarization Concept Checker
- Charging Concept Checker
- Name That Charge Simulation
- Coulomb's Law Concept Checker
- Electric Field Lines Concept Checker
- Put the Charge in the Goal Concept Checker
- Circuit Builder Concept Checker (Series Circuits)
- Circuit Builder Concept Checker (Parallel Circuits)
- Circuit Builder Concept Checker (∆V-I-R)
- Circuit Builder Concept Checker (Voltage Drop)
- Equivalent Resistance Interactive
- Pendulum Motion Simulation Concept Checker
- Mass on a Spring Simulation Concept Checker
- Particle Wave Simulation Concept Checker
- Boundary Behavior Simulation Concept Checker
- Slinky Wave Simulator Concept Checker
- Simple Wave Simulator Concept Checker
- Wave Addition Simulation Concept Checker
- Standing Wave Maker Simulation Concept Checker
- Color Addition Concept Checker
- Painting With CMY Concept Checker
- Stage Lighting Concept Checker
- Filtering Away Concept Checker
- InterferencePatterns Concept Checker
- Young's Experiment Interactive
- Plane Mirror Images Interactive
- Who Can See Who Concept Checker
- Optics Bench (Mirrors) Concept Checker
- Name That Image (Mirrors) Interactive
- Refraction Concept Checker
- Total Internal Reflection Concept Checker
- Optics Bench (Lenses) Concept Checker
- Kinematics Preview
- Velocity Time Graphs Preview
- Moving Cart on an Inclined Plane Preview
- Stopping Distance Preview
- Cart, Bricks, and Bands Preview
- Fan Cart Study Preview
- Friction Preview
- Coffee Filter Lab Preview
- Friction, Speed, and Stopping Distance Preview
- Up and Down Preview
- Projectile Range Preview
- Ballistics Preview
- Juggling Preview
- Marshmallow Launcher Preview
- Air Bag Safety Preview
- Colliding Carts Preview
- Collisions Preview
- Engineering Safer Helmets Preview
- Push the Plow Preview
- Its All Uphill Preview
- Energy on an Incline Preview
- Modeling Roller Coasters Preview
- Hot Wheels Stopping Distance Preview
- Ball Bat Collision Preview
- Energy in Fields Preview
- Weightlessness Training Preview
- Roller Coaster Loops Preview
- Universal Gravitation Preview
- Keplers Laws Preview
- Kepler's Third Law Preview
- Charge Interactions Preview
- Sticky Tape Experiments Preview
- Wire Gauge Preview
- Voltage, Current, and Resistance Preview
- Light Bulb Resistance Preview
- Series and Parallel Circuits Preview
- Thermal Equilibrium Preview
- Linear Expansion Preview
- Heating Curves Preview
- Electricity and Magnetism - Part 1 Preview
- Electricity and Magnetism - Part 2 Preview
- Vibrating Mass on a Spring Preview
- Period of a Pendulum Preview
- Wave Speed Preview
- Slinky-Experiments Preview
- Standing Waves in a Rope Preview
- Sound as a Pressure Wave Preview
- DeciBel Scale Preview
- DeciBels, Phons, and Sones Preview
- Sound of Music Preview
- Shedding Light on Light Bulbs Preview
- Models of Light Preview
- Electromagnetic Radiation Preview
- Electromagnetic Spectrum Preview
- EM Wave Communication Preview
- Digitized Data Preview
- Light Intensity Preview
- Concave Mirrors Preview
- Object Image Relations Preview
- Snells Law Preview
- Reflection vs. Transmission Preview
- Magnification Lab Preview
- Reactivity Preview
- Ions and the Periodic Table Preview
- Periodic Trends Preview
- Intermolecular Forces Preview
- Melting Points and Boiling Points Preview
- Reaction Rates Preview
- Ammonia Factory Preview
- Stoichiometry Preview
- Nuclear Chemistry Preview
- Gaining Teacher Access
- Tasks and Classes
- Tasks - Classic
- Subscription
- Subscription Locator
- 1-D Kinematics
- Newton's Laws
- Vectors - Motion and Forces in Two Dimensions
- Momentum and Its Conservation
- Work and Energy
- Circular Motion and Satellite Motion
- Thermal Physics
- Static Electricity
- Electric Circuits
- Vibrations and Waves
- Sound Waves and Music
- Light and Color
- Reflection and Mirrors
- About the Physics Interactives
- Task Tracker
- Usage Policy
- Newtons Laws
- Vectors and Projectiles
- Forces in 2D
- Momentum and Collisions
- Circular and Satellite Motion
- Balance and Rotation
- Electromagnetism
- Waves and Sound
- Atomic Physics
- Forces in Two Dimensions
- Work, Energy, and Power
- Circular Motion and Gravitation
- Sound Waves
- 1-Dimensional Kinematics
- Circular, Satellite, and Rotational Motion
- Einstein's Theory of Special Relativity
- Waves, Sound and Light
- QuickTime Movies
- About the Concept Builders
- Pricing For Schools
- Directions for Version 2
- Measurement and Units
- Relationships and Graphs
- Rotation and Balance
- Vibrational Motion
- Reflection and Refraction
- Teacher Accounts
- Task Tracker Directions
- Kinematic Concepts
- Kinematic Graphing
- Wave Motion
- Sound and Music
- About CalcPad
- 1D Kinematics
- Vectors and Forces in 2D
- Simple Harmonic Motion
- Rotational Kinematics
- Rotation and Torque
- Rotational Dynamics
- Electric Fields, Potential, and Capacitance
- Transient RC Circuits
- Light Waves
- Units and Measurement
- Stoichiometry
- Molarity and Solutions
- Thermal Chemistry
- Acids and Bases
- Kinetics and Equilibrium
- Solution Equilibria
- Oxidation-Reduction
- Nuclear Chemistry
- NGSS Alignments
- 1D-Kinematics
- Projectiles
- Circular Motion
- Magnetism and Electromagnetism
- Graphing Practice
- About the ACT
- ACT Preparation
- For Teachers
- Other Resources
- Newton's Laws of Motion
- Work and Energy Packet
- Static Electricity Review
- Solutions Guide
- Solutions Guide Digital Download
- Motion in One Dimension
- Work, Energy and Power
- Frequently Asked Questions
- Purchasing the Download
- Purchasing the CD
- Purchasing the Digital Download
- About the NGSS Corner
- NGSS Search
- Force and Motion DCIs - High School
- Energy DCIs - High School
- Wave Applications DCIs - High School
- Force and Motion PEs - High School
- Energy PEs - High School
- Wave Applications PEs - High School
- Crosscutting Concepts
- The Practices
- Physics Topics
- NGSS Corner: Activity List
- NGSS Corner: Infographics
- About the Toolkits
- Position-Velocity-Acceleration
- Position-Time Graphs
- Velocity-Time Graphs
- Newton's First Law
- Newton's Second Law
- Newton's Third Law
- Terminal Velocity
- Projectile Motion
- Forces in 2 Dimensions
- Impulse and Momentum Change
- Momentum Conservation
- Work-Energy Fundamentals
- Work-Energy Relationship
- Roller Coaster Physics
- Satellite Motion
- Electric Fields
- Circuit Concepts
- Series Circuits
- Parallel Circuits
- Describing-Waves
- Wave Behavior Toolkit
- Standing Wave Patterns
- Resonating Air Columns
- Wave Model of Light
- Plane Mirrors
- Curved Mirrors
- Teacher Guide
- Using Lab Notebooks
- Current Electricity
- Light Waves and Color
- Reflection and Ray Model of Light
- Refraction and Ray Model of Light
- Classes (Legacy Version)
- Teacher Resources
- Subscriptions

- Newton's Laws
- Einstein's Theory of Special Relativity
- About Concept Checkers
- School Pricing
- Newton's Laws of Motion
- Newton's First Law
- Newton's Third Law
- The Speed of Sound
- Pitch and Frequency
- Intensity and the Decibel Scale
- The Human Ear
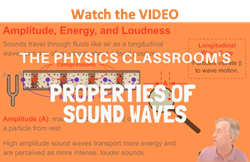
Since the speed of a wave is defined as the distance that a point on a wave (such as a compression or a rarefaction) travels per unit of time, it is often expressed in units of meters/second (abbreviated m/s). In equation form, this is
The faster a sound wave travels, the more distance it will cover in the same period of time. If a sound wave were observed to travel a distance of 700 meters in 2 seconds, then the speed of the wave would be 350 m/s. A slower wave would cover less distance - perhaps 660 meters - in the same time period of 2 seconds and thus have a speed of 330 m/s. Faster waves cover more distance in the same period of time.
Factors Affecting Wave Speed
The speed of any wave depends upon the properties of the medium through which the wave is traveling. Typically there are two essential types of properties that affect wave speed - inertial properties and elastic properties. Elastic properties are those properties related to the tendency of a material to maintain its shape and not deform whenever a force or stress is applied to it. A material such as steel will experience a very small deformation of shape (and dimension) when a stress is applied to it. Steel is a rigid material with a high elasticity. On the other hand, a material such as a rubber band is highly flexible; when a force is applied to stretch the rubber band, it deforms or changes its shape readily. A small stress on the rubber band causes a large deformation. Steel is considered to be a stiff or rigid material, whereas a rubber band is considered a flexible material. At the particle level, a stiff or rigid material is characterized by atoms and/or molecules with strong attractions for each other. When a force is applied in an attempt to stretch or deform the material, its strong particle interactions prevent this deformation and help the material maintain its shape. Rigid materials such as steel are considered to have a high elasticity. (Elastic modulus is the technical term). The phase of matter has a tremendous impact upon the elastic properties of the medium. In general, solids have the strongest interactions between particles, followed by liquids and then gases. For this reason, longitudinal sound waves travel faster in solids than they do in liquids than they do in gases. Even though the inertial factor may favor gases, the elastic factor has a greater influence on the speed ( v ) of a wave, thus yielding this general pattern:
Inertial properties are those properties related to the material's tendency to be sluggish to changes in its state of motion. The density of a medium is an example of an inertial property . The greater the inertia (i.e., mass density) of individual particles of the medium, the less responsive they will be to the interactions between neighboring particles and the slower that the wave will be. As stated above, sound waves travel faster in solids than they do in liquids than they do in gases. However, within a single phase of matter, the inertial property of density tends to be the property that has a greatest impact upon the speed of sound. A sound wave will travel faster in a less dense material than a more dense material. Thus, a sound wave will travel nearly three times faster in Helium than it will in air. This is mostly due to the lower mass of Helium particles as compared to air particles.
The Speed of Sound in Air
The speed of a sound wave in air depends upon the properties of the air, mostly the temperature, and to a lesser degree, the humidity. Humidity is the result of water vapor being present in air. Like any liquid, water has a tendency to evaporate. As it does, particles of gaseous water become mixed in the air. This additional matter will affect the mass density of the air (an inertial property). The temperature will affect the strength of the particle interactions (an elastic property). At normal atmospheric pressure, the temperature dependence of the speed of a sound wave through dry air is approximated by the following equation:
where T is the temperature of the air in degrees Celsius. Using this equation to determine the speed of a sound wave in air at a temperature of 20 degrees Celsius yields the following solution.
v = 331 m/s + (0.6 m/s/C)•(20 C)
v = 331 m/s + 12 m/s
v = 343 m/s
(The above equation relating the speed of a sound wave in air to the temperature provides reasonably accurate speed values for temperatures between 0 and 100 Celsius. The equation itself does not have any theoretical basis; it is simply the result of inspecting temperature-speed data for this temperature range. Other equations do exist that are based upon theoretical reasoning and provide accurate data for all temperatures. Nonetheless, the equation above will be sufficient for our use as introductory Physics students.)
Look It Up!
Using wave speed to determine distances.
At normal atmospheric pressure and a temperature of 20 degrees Celsius, a sound wave will travel at approximately 343 m/s; this is approximately equal to 750 miles/hour. While this speed may seem fast by human standards (the fastest humans can sprint at approximately 11 m/s and highway speeds are approximately 30 m/s), the speed of a sound wave is slow in comparison to the speed of a light wave. Light travels through air at a speed of approximately 300 000 000 m/s; this is nearly 900 000 times the speed of sound. For this reason, humans can observe a detectable time delay between the thunder and the lightning during a storm. The arrival of the light wave from the location of the lightning strike occurs in so little time that it is essentially negligible. Yet the arrival of the sound wave from the location of the lightning strike occurs much later. The time delay between the arrival of the light wave (lightning) and the arrival of the sound wave (thunder) allows a person to approximate his/her distance from the storm location. For instance if the thunder is heard 3 seconds after the lightning is seen, then sound (whose speed is approximated as 345 m/s) has traveled a distance of
If this value is converted to miles (divide by 1600 m/1 mi), then the storm is a distance of 0.65 miles away.
Another phenomenon related to the perception of time delays between two events is an echo . A person can often perceive a time delay between the production of a sound and the arrival of a reflection of that sound off a distant barrier. If you have ever made a holler within a canyon, perhaps you have heard an echo of your holler off a distant canyon wall. The time delay between the holler and the echo corresponds to the time for the holler to travel the round-trip distance to the canyon wall and back. A measurement of this time would allow a person to estimate the one-way distance to the canyon wall. For instance if an echo is heard 1.40 seconds after making the holler , then the distance to the canyon wall can be found as follows:
The canyon wall is 242 meters away. You might have noticed that the time of 0.70 seconds is used in the equation. Since the time delay corresponds to the time for the holler to travel the round-trip distance to the canyon wall and back, the one-way distance to the canyon wall corresponds to one-half the time delay.
While an echo is of relatively minimal importance to humans, echolocation is an essential trick of the trade for bats. Being a nocturnal creature, bats must use sound waves to navigate and hunt. They produce short bursts of ultrasonic sound waves that reflect off objects in their surroundings and return. Their detection of the time delay between the sending and receiving of the pulses allows a bat to approximate the distance to surrounding objects. Some bats, known as Doppler bats, are capable of detecting the speed and direction of any moving objects by monitoring the changes in frequency of the reflected pulses. These bats are utilizing the physics of the Doppler effect discussed in an earlier unit (and also to be discussed later in Lesson 3 ). This method of echolocation enables a bat to navigate and to hunt.
The Wave Equation Revisited
Like any wave, a sound wave has a speed that is mathematically related to the frequency and the wavelength of the wave. As discussed in a previous unit , the mathematical relationship between speed, frequency and wavelength is given by the following equation.
Using the symbols v , λ , and f , the equation can be rewritten as
Check Your Understanding
1. An automatic focus camera is able to focus on objects by use of an ultrasonic sound wave. The camera sends out sound waves that reflect off distant objects and return to the camera. A sensor detects the time it takes for the waves to return and then determines the distance an object is from the camera. If a sound wave (speed = 340 m/s) returns to the camera 0.150 seconds after leaving the camera, how far away is the object?
Answer = 25.5 m
The speed of the sound wave is 340 m/s. The distance can be found using d = v • t resulting in an answer of 25.5 m. Use 0.075 seconds for the time since 0.150 seconds refers to the round-trip distance.
2. On a hot summer day, a pesky little mosquito produced its warning sound near your ear. The sound is produced by the beating of its wings at a rate of about 600 wing beats per second.
a. What is the frequency in Hertz of the sound wave? b. Assuming the sound wave moves with a velocity of 350 m/s, what is the wavelength of the wave?
Part a Answer: 600 Hz (given)
Part b Answer: 0.583 meters
3. Doubling the frequency of a wave source doubles the speed of the waves.
a. True b. False
Doubling the frequency will halve the wavelength; speed is unaffected by the alteration in the frequency. The speed of a wave depends upon the properties of the medium.
4. Playing middle C on the piano keyboard produces a sound with a frequency of 256 Hz. Assuming the speed of sound in air is 345 m/s, determine the wavelength of the sound corresponding to the note of middle C.
Answer: 1.35 meters (rounded)
Let λ = wavelength. Use v = f • λ where v = 345 m/s and f = 256 Hz. Rearrange the equation to the form of λ = v / f. Substitute and solve.
5. Most people can detect frequencies as high as 20 000 Hz. Assuming the speed of sound in air is 345 m/s, determine the wavelength of the sound corresponding to this upper range of audible hearing.
Answer: 0.0173 meters (rounded)
Let λ = wavelength. Use v = f • λ where v = 345 m/s and f = 20 000 Hz. Rearrange the equation to the form of λ = v / f. Substitute and solve.
6. An elephant produces a 10 Hz sound wave. Assuming the speed of sound in air is 345 m/s, determine the wavelength of this infrasonic sound wave.
Answer: 34.5 meters
Let λ = wavelength. Use v = f • λ where v = 345 m/s and f = 10 Hz. Rearrange the equation to the form of λ = v / f. Substitute and solve.
7. Determine the speed of sound on a cold winter day (T=3 degrees C).
Answer: 332.8 m/s
The speed of sound in air is dependent upon the temperature of air. The dependence is expressed by the equation:
v = 331 m/s + (0.6 m/s/C) • T
where T is the temperature in Celsius. Substitute and solve.
v = 331 m/s + (0.6 m/s/C) • 3 C v = 331 m/s + 1.8 m/s v = 332.8 m/s
8. Miles Tugo is camping in Glacier National Park. In the midst of a glacier canyon, he makes a loud holler. He hears an echo 1.22 seconds later. The air temperature is 20 degrees C. How far away are the canyon walls?
Answer = 209 m
The speed of the sound wave at this temperature is 343 m/s (using the equation described in the Tutorial). The distance can be found using d = v • t resulting in an answer of 343 m. Use 0.61 second for the time since 1.22 seconds refers to the round-trip distance.
9. Two sound waves are traveling through a container of unknown gas. Wave A has a wavelength of 1.2 m. Wave B has a wavelength of 3.6 m. The velocity of wave B must be __________ the velocity of wave A.
a. one-ninth b. one-third c. the same as d. three times larger than
The speed of a wave does not depend upon its wavelength, but rather upon the properties of the medium. The medium has not changed, so neither has the speed.
10. Two sound waves are traveling through a container of unknown gas. Wave A has a wavelength of 1.2 m. Wave B has a wavelength of 3.6 m. The frequency of wave B must be __________ the frequency of wave A.
Since Wave B has three times the wavelength of Wave A, it must have one-third the frequency. Frequency and wavelength are inversely related.
- Interference and Beats

- Science Notes Posts
- Contact Science Notes
- Todd Helmenstine Biography
- Anne Helmenstine Biography
- Free Printable Periodic Tables (PDF and PNG)
- Periodic Table Wallpapers
- Interactive Periodic Table
- Periodic Table Posters
- How to Grow Crystals
- Chemistry Projects
- Fire and Flames Projects
- Holiday Science
- Chemistry Problems With Answers
- Physics Problems
- Unit Conversion Example Problems
- Chemistry Worksheets
- Biology Worksheets
- Periodic Table Worksheets
- Physical Science Worksheets
- Science Lab Worksheets
- My Amazon Books
Speed of Sound in Physics

In physics, the speed of sound is the distance traveled per unit of time by a sound wave through a medium. It is highest for stiff solids and lowest for gases. There is no sound or speed of sound in a vacuum because sound (unlike light ) requires a medium in order to propogate.
What Is the Speed of Sound?
Usually, conversations about the speed of sound refer to the speed of sound of dry air (humidity changes the value). The value depends on temperature.
- at 20 ° C or 68 ° F: 343 m/s or 1234.8 kph or 1125ft/s or 767 mph
- at 0 ° C or 32 ° F: 331 m/s or 1191.6 kph or 1086 ft/s or 740 mph
Mach Numher
The Mach number is the ratio of air speed to the speed of sound. So, an object at Mach 1 is traveling at the speed of sound. Exceeding Mach 1 is breaking the sound barrier or is supersonic . At Mach 2, the object travels twice the speed of sound. Mach 3 is three times the speed of sound, and so on.
Remember that the speed of sound depends on temperature, so you break sound barrier at a lower speed when the temperature is colder. To put it another way, it gets colder as you get higher in the atmosphere, so an aircraft might break the sound barrier at a higher altitude even if it does not increase its speed.
Solids, Liquids, and Gases
The speed of sound is greatest for solids, intermediate for liquids, and lowest for gases:
v solid > v liquid >v gas
Particles in a gas undergo elastic collisions and the particles are widely separated. In contrast, particles in a solid are locked into place (rigid or stiff), so a vibration readily transmits through chemical bonds.
Here are examples of the difference between the speed of sound in different materials:
- Diamond (solid): 12000 m/s
- Copper (solid): 6420 m/s
- Iron (solid): 5120 m/s
- Water (liquid) 1481 m/s
- Helium (gas): 965 m/s
- Dry air (gas): 343 m/s
Sounds waves transfer energy to matter via a compression wave (in all phases) and also shear wave (in solids). The pressure disturbs a particle, which then impacts its neighbor, and continues traveling through the medium. The speed is how quickly the wave moves, while the frequency is the number of vibrations the particle makes per unit of time.
The Hot Chocolate Effect
The hot chocolate effect describes the phenomenon where the pitch you hear from tapping a cup of hot liquid rises after adding a soluble powder (like cocoa powder into hot water). Stirring in the powder introduces gas bubbles that reduce the speed of sound of the liquid and lower the frequency (pitch) of the waves. Once the bubbles clear, the speed of sound and the frequency increase again.
Speed of Sound Formulas
There are several formulas for calculating the speed of sound. Here are a few of the most common ones:
For gases these approximations work in most situations:
For this formula, use the Celsius temperature of the gas.
v = 331 m/s + (0.6 m/s/C)•T
Here is another common formula:
v = (γRT) 1/2
- γ is the ratio of specific heat values or adiabatic index (1.4 for air at STP )
- R is a gas constant (282 m 2 /s 2 /K for air)
- T is the absolute temperature (Kelvin)
The Newton-Laplace formula works for both gases and liquids (fluids):
v = (K s /ρ) 1/2
- K s is the coefficient of stiffness or bulk modulus of elasticity for gases
- ρ is the density of the material
So solids, the situation is more complicated because shear waves play into the formula. There can be sound waves with different velocities, depending on the mode of deformation. The simplest formula is for one-dimensional solids, like a long rod of a material:
v = (E/ρ) 1/2
- E is Young’s modulus
Note that the speed of sound decreases with density! It increases according to the stiffness of a medium. This is not intuitively obvious, since often a dense material is also stiff. But, consider that the speed of sound in a diamond is much faster than the speed in iron. Diamond is less dense than iron and also stiffer.
Factors That Affect the Speed of Sound
The primary factors affecting the speed of sound of a fluid (gas or liquid) are its temperature and its chemical composition. There is a weak dependence on frequency and atmospheric pressure that is omitted from the simplest equations.
While sound travels only as compression waves in a fluid, it also travels as shear waves in a solid. So, a solid’s stiffness, density, and compressibility also factor into the speed of sound.
Speed of Sound on Mars
Thanks to the Perseverance rover, scientists know the speed of sound on Mars. The Martian atmosphere is much colder than Earth’s, its thin atmosphere has a much lower pressure, and it consists mainly of carbon dioxide rather than nitrogen. As expected, the speed of sound on Mars is slower than on Earth. It travels at around 240 m/s or about 30% slower than on Earth.
What scientists did not expect is that the speed of sound varies for different frequencies. A high pitched sound, like from the rover’s laser, travels faster at around 250 m/s. So, for example, if you listened to a symphony recording from a distance on Mars you’d hear the various instruments at different times. The explanation has to do with the vibrational modes of carbon dioxide, the primary component of the Martian atmosphere. Also, it’s worth noting that the atmospheric pressure is so low that there really isn’t any much sound at all from a source more than a few meters away.
Speed of Sound Example Problems
Find the speed of sound on a cold day when the temperature is 2 ° C.
The simplest formula for finding the answer is the approximation:
v = 331 m/s + (0.6 m/s/C) • T
Since the given temperature is already in Celsius, just plug in the value:
v = 331 m/s + (0.6 m/s/C) • 2 C = 331 m/s + 1.2 m/s = 332.2 m/s
You’re hiking in a canyon, yell “hello”, and hear an echo after 1.22 seconds. The air temperature is 20 ° C. How far away is the canyon wall?
The first step is finding the speed of sound at the temperature:
v = 331 m/s + (0.6 m/s/C) • T v = 331 m/s + (0.6 m/s/C) • 20 C = 343 m/s (which you might have memorized as the usual speed of sound)
Next, find the distance using the formula:
d = v• T d = 343 m/s • 1.22 s = 418.46 m
But, this is the round-trip distance! The distance to the canyon wall is half of this or 209 meters.
If you double the frequency of sound, it double the speed of its waves. True or false?
This is (mostly) false. Doubling the frequency halves the wavelength, but the speed depends on the properties of the medium and not its frequency or wavelength. Frequency only affects the speed of sound for certain media (like the carbon dioxide atmosphere of Mars).
- Everest, F. (2001). The Master Handbook of Acoustics . New York: McGraw-Hill. ISBN 978-0-07-136097-5.
- Kinsler, L.E.; Frey, A.R.; Coppens, A.B.; Sanders, J.V. (2000). Fundamentals of Acoustics (4th ed.). New York: John Wiley & Sons. ISBN 0-471-84789-5.
- Maurice, S.; et al. (2022). “In situ recording of Mars soundscape:. Nature. 605: 653-658. doi: 10.1038/s41586-022-04679-0
- Wong, George S. K.; Zhu, Shi-ming (1995). “Speed of sound in seawater as a function of salinity, temperature, and pressure”. The Journal of the Acoustical Society of America . 97 (3): 1732. doi: 10.1121/1.413048
Related Posts

- April 24, 2024 | Study Finds Artificial Sweetener Can Cause Healthy Gut Bacteria To Become Diseased
- April 24, 2024 | Ash Shrouded Skies: Powerful Volcanic Eruption at Mount Ruang
- April 24, 2024 | Startling Discovery: Cancer Can Arise Without Genetic Mutations
- April 24, 2024 | Liftoff! NASA’s Next-Generation Solar Sail Boom Technology Launched
- April 24, 2024 | 3.7 Billion Years Old: Oldest Undisputed Evidence of Earth’s Magnetic Field Uncovered in Greenland
Fundamental Physics: Scientists Discover the Fastest Possible Speed of Sound
By Queen Mary University of London October 11, 2020
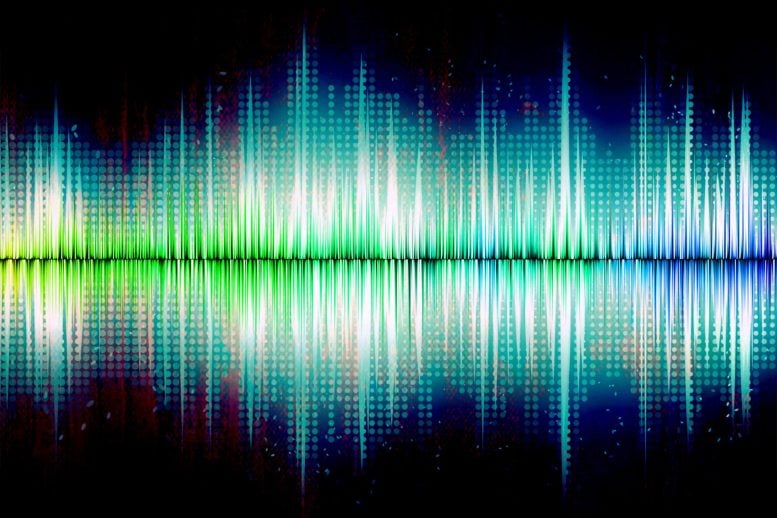
Scientists find the ultimate speed of sound: about 36 km per second (80,500 mph), twice as fast as sound in diamond, the hardest known material.
A research collaboration between Queen Mary University of London, the University of Cambridge, and the Institute for High Pressure Physics in Troitsk has discovered the fastest possible speed of sound.
The result — about 36 km per second (80,500 mph) — is around twice as fast as the speed of sound in diamond, the hardest known material in the world.
Waves, such as sound or light waves, are disturbances that move energy from one place to another. Sound waves can travel through different mediums, such as air or water, and move at different speeds depending on what they’re traveling through. For example, they move through solids much faster than they would through liquids or gases, which is why you’re able to hear an approaching train much faster if you listen to the sound propagating in the rail track rather than through the air.
Einstein’s theory of special relativity sets the absolute speed limit at which a wave can travel which is the speed of light, and is equal to about 300,000 km (186,400 mi) per second. However, until now it was not known whether sound waves also have an upper-speed limit when traveling through solids or liquids.
The study, published in the journal Science Advances , shows that predicting the upper limit of the speed of sound is dependent on two dimensionless fundamental constants: the fine structure constant and the proton-to-electron mass ratio.
These two numbers are already known to play an important role in understanding our Universe. Their finely-tuned values govern nuclear reactions such as proton decay and nuclear synthesis in stars and the balance between the two numbers provides a narrow ‘ habitable zone ‘ where stars and planets can form and life-supporting molecular structures can emerge. However, the new findings suggest that these two fundamental constants can also influence other scientific fields, such as materials science and condensed matter physics, by setting limits to specific material properties such as the speed of sound.
The scientists tested their theoretical prediction on a wide range of materials and addressed one specific prediction of their theory that the speed of sound should decrease with the mass of the atom . This prediction implies that sound is the fastest in solid atomic hydrogen. However, hydrogen is an atomic solid at very high pressure above 1 million atmospheres only, pressure comparable to those in the core of gas giants like Jupiter . At those pressures, hydrogen becomes a fascinating metallic solid conducting electricity just like copper and is predicted to be a room-temperature superconductor. Therefore, researchers performed state-of-the-art quantum mechanical calculations to test this prediction and found that the speed of sound in solid atomic hydrogen is close to the theoretical fundamental limit.
Chris Pickard, Professor of Materials Science at the University of Cambridge, said: “Soundwaves in solids are already hugely important across many scientific fields. For example, seismologists use sound waves initiated by earthquakes deep in the Earth interior to understand the nature of seismic events and the properties of Earth composition. They’re also of interest to materials scientists because sound waves are related to important elastic properties including the ability to resist stress.”
Professor Kostya Trachenko, Professor of Physics at Queen Mary, added: “We believe the findings of this study could have further scientific applications by helping us to find and understand limits of different properties such as viscosity and thermal conductivity relevant for high-temperature superconductivity, quark-gluon plasma , and even black hole physics.”
Reference: “Speed of sound from fundamental physical constants” by K. Trachenko, B. Monserrat, C. J. Pickard and V. V. Brazhkin, 8 October 2020, Science Advances . DOI: 10.1126/sciadv.abc8662
More on SciTechDaily
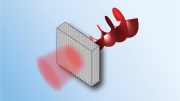
Physicists Discover a Remarkable New Type of Sound Wave
Unusual sound waves induced using laser pulses – 140 years after alexander graham bell reported that light can be converted into sound waves.
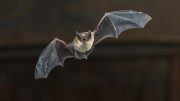
Bats’ Surprising “Supersense” of Time: They Know the Speed of Sound From Birth
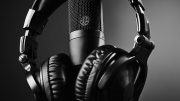
Sound Science: Great Headphones Blend Physics, Anatomy and Psychology
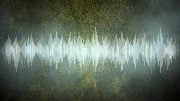
MIT Physicists Created a Perfect Fluid and Captured the Sound – Listen Here
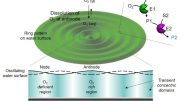
Using Sound To Control Enzymatic Reactions
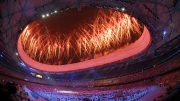
Olympic Sound Design: 3,600 Microphones and Counting
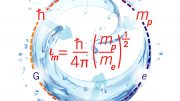
Scientists Discover Fundamental Physical Constants Govern How Runny a Liquid Can Be
Be the first to comment on "fundamental physics: scientists discover the fastest possible speed of sound", leave a comment cancel reply.
Email address is optional. If provided, your email will not be published or shared.
Save my name, email, and website in this browser for the next time I comment.
- Random article
- Teaching guide
- Privacy & cookies
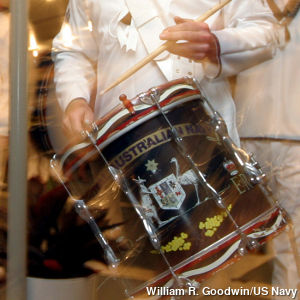
by Chris Woodford . Last updated: July 23, 2023.
Photo: Sound is energy we hear made by things that vibrate. Photo by William R. Goodwin courtesy of US Navy and Wikimedia Commons .
What is sound?
Photo: Sensing with sound: Light doesn't travel well through ocean water: over half the light falling on the sea surface is absorbed within the first meter of water; 100m down and only 1 percent of the surface light remains. That's largely why mighty creatures of the deep rely on sound for communication and navigation. Whales, famously, "talk" to one another across entire ocean basins, while dolphins use sound, like bats, for echolocation. Photo by Bill Thompson courtesy of US Fish and Wildlife Service .
Robert Boyle's classic experiment
Artwork: Robert Boyle's famous experiment with an alarm clock.
How sound travels
Artwork: Sound waves and ocean waves compared. Top: Sound waves are longitudinal waves: the air moves back and forth along the same line as the wave travels, making alternate patterns of compressions and rarefactions. Bottom: Ocean waves are transverse waves: the water moves back and forth at right angles to the line in which the wave travels.
The science of sound waves
Picture: Reflected sound is extremely useful for "seeing" underwater where light doesn't really travel—that's the basic idea behind sonar. Here's a side-scan sonar (reflected sound) image of a World War II boat wrecked on the seabed. Photo courtesy of U.S. National Oceanographic and Atmospheric Administration, US Navy, and Wikimedia Commons .
Whispering galleries and amphitheaters
Photos by Carol M. Highsmith: 1) The Capitol in Washington, DC has a whispering gallery inside its dome. Photo credit: The George F. Landegger Collection of District of Columbia Photographs in Carol M. Highsmith's America, Library of Congress , Prints and Photographs Division. 2) It's easy to hear people talking in the curved memorial amphitheater building at Arlington National Cemetery, Arlington, Virginia. Photo credit: Photographs in the Carol M. Highsmith Archive, Library of Congress , Prints and Photographs Division.
Measuring waves
Understanding amplitude and frequency, why instruments sound different, the speed of sound.
Photo: Breaking through the sound barrier creates a sonic boom. The mist you can see, which is called a condensation cloud, isn't necessarily caused by an aircraft flying supersonic: it can occur at lower speeds too. It happens because moist air condenses due to the shock waves created by the plane. You might expect the plane to compress the air as it slices through. But the shock waves it generates alternately expand and contract the air, producing both compressions and rarefactions. The rarefactions cause very low pressure and it's these that make moisture in the air condense, producing the cloud you see here. Photo by John Gay courtesy of US Navy and Wikimedia Commons .
Why does sound go faster in some things than in others?
Chart: Generally, sound travels faster in solids (right) than in liquids (middle) or gases (left)... but there are exceptions!
How to measure the speed of sound
Sound in practice, if you liked this article..., find out more, on this website.
- Electric guitars
- Speech synthesis
- Synthesizers
On other sites
- Explore Sound : A comprehensive educational site from the Acoustical Society of America, with activities for students of all ages.
- Sound Waves : A great collection of interactive science lessons from the University of Salford, which explains what sound waves are and the different ways in which they behave.
Educational books for younger readers
- Sound (Science in a Flash) by Georgia Amson-Bradshaw. Franklin Watts/Hachette, 2020. Simple facts, experiments, and quizzes fill this book; the visually exciting design will appeal to reluctant readers. Also for ages 7–9.
- Sound by Angela Royston. Raintree, 2017. A basic introduction to sound and musical sounds, including simple activities. Ages 7–9.
- Experimenting with Sound Science Projects by Robert Gardner. Enslow Publishers, 2013. A comprehensive 120-page introduction, running through the science of sound in some detail, with plenty of hands-on projects and activities (including welcome coverage of how to run controlled experiments using the scientific method). Ages 9–12.
- Cool Science: Experiments with Sound and Hearing by Chris Woodford. Gareth Stevens Inc, 2010. One of my own books, this is a short introduction to sound through practical activities, for ages 9–12.
- Adventures in Sound with Max Axiom, Super Scientist by Emily Sohn. Capstone, 2007. The original, graphic novel (comic book) format should appeal to reluctant readers. Ages 8–10.
Popular science
- The Sound Book: The Science of the Sonic Wonders of the World by Trevor Cox. W. W. Norton, 2014. An entertaining tour through everyday sound science.
Academic books
- Master Handbook of Acoustics by F. Alton Everest and Ken Pohlmann. McGraw-Hill Education, 2015. A comprehensive reference for undergraduates and sound-design professionals.
- The Science of Sound by Thomas D. Rossing, Paul A. Wheeler, and F. Richard Moore. Pearson, 2013. One of the most popular general undergraduate texts.
Text copyright © Chris Woodford 2009, 2021. All rights reserved. Full copyright notice and terms of use .
Rate this page
Tell your friends, cite this page, more to explore on our website....
- Get the book
- Send feedback
14.1 Speed of Sound, Frequency, and Wavelength
Section learning objectives.
By the end of this section, you will be able to do the following:
- Relate the characteristics of waves to properties of sound waves
- Describe the speed of sound and how it changes in various media
- Relate the speed of sound to frequency and wavelength of a sound wave
Teacher Support
The learning objectives in this section will help your students master the following standards:
- (A) examine and describe oscillatory motion and wave propagation in various types of media;
- (B) investigate and analyze characteristics of waves, including velocity, frequency, amplitude, and wavelength, and calculate using the relationship between wave speed, frequency, and wavelength;
- (C) compare characteristics and behaviors of transverse waves, including electromagnetic waves and the electromagnetic spectrum, and characteristics and behaviors of longitudinal waves, including sound waves;
- (F) describe the role of wave characteristics and behaviors in medical and industrial applications.
In addition, the High School Physics Laboratory Manual addresses content in this section in the lab titled: Waves, as well as the following standards:
- (B) investigate and analyze characteristics of waves, including velocity, frequency, amplitude, and wavelength, and calculate using the relationship between wave speed, frequency, and wavelength.
Section Key Terms
[BL] [OL] Review waves and types of waves—mechanical and non-mechanical, transverse and longitudinal, pulse and periodic. Review properties of waves—amplitude, period, frequency, velocity and their inter-relations.
Properties of Sound Waves
Sound is a wave. More specifically, sound is defined to be a disturbance of matter that is transmitted from its source outward. A disturbance is anything that is moved from its state of equilibrium. Some sound waves can be characterized as periodic waves, which means that the atoms that make up the matter experience simple harmonic motion .
A vibrating string produces a sound wave as illustrated in Figure 14.2 , Figure 14.3 , and Figure 14.4 . As the string oscillates back and forth, part of the string’s energy goes into compressing and expanding the surrounding air. This creates slightly higher and lower pressures. The higher pressure... regions are compressions, and the low pressure regions are rarefactions . The pressure disturbance moves through the air as longitudinal waves with the same frequency as the string. Some of the energy is lost in the form of thermal energy transferred to the air. You may recall from the chapter on waves that areas of compression and rarefaction in longitudinal waves (such as sound) are analogous to crests and troughs in transverse waves .
The amplitude of a sound wave decreases with distance from its source, because the energy of the wave is spread over a larger and larger area. But some of the energy is also absorbed by objects, such as the eardrum in Figure 14.5 , and some of the energy is converted to thermal energy in the air. Figure 14.4 shows a graph of gauge pressure versus distance from the vibrating string. From this figure, you can see that the compression of a longitudinal wave is analogous to the peak of a transverse wave, and the rarefaction of a longitudinal wave is analogous to the trough of a transverse wave. Just as a transverse wave alternates between peaks and troughs, a longitudinal wave alternates between compression and rarefaction.
The Speed of Sound
[BL] Review the fact that sound is a mechanical wave and requires a medium through which it is transmitted.
[OL] [AL] Ask students if they know the speed of sound and if not, ask them to take a guess. Ask them why the sound of thunder is heard much after the lightning is seen during storms. This phenomenon is also observed during a display of fireworks. Through this discussion, develop the concept that the speed of sound is finite and measurable and is much slower than that of light.
The speed of sound varies greatly depending upon the medium it is traveling through. The speed of sound in a medium is determined by a combination of the medium’s rigidity (or compressibility in gases) and its density. The more rigid (or less compressible) the medium, the faster the speed of sound. The greater the density of a medium, the slower the speed of sound. The speed of sound in air is low, because air is compressible. Because liquids and solids are relatively rigid and very difficult to compress, the speed of sound in such media is generally greater than in gases. Table 14.1 shows the speed of sound in various media. Since temperature affects density, the speed of sound varies with the temperature of the medium through which it’s traveling to some extent, especially for gases.
Misconception Alert
Students might be confused between rigidity and density and how they affect the speed of sound. The speed of sound is slower in denser media. Solids are denser than gases. However, they are also very rigid, and hence sound travels faster in solids. Stress on the fact that the speed of sound always depends on a combination of these two properties of any medium.
[BL] Note that in the table, the speed of sound in very rigid materials such as glass, aluminum, and steel ... is quite high, whereas the speed in rubber, which is considerably less rigid, is quite low.
The Relationship Between the Speed of Sound and the Frequency and Wavelength of a Sound Wave
Sound, like all waves, travels at certain speeds through different media and has the properties of frequency and wavelength . Sound travels much slower than light—you can observe this while watching a fireworks display (see Figure 14.6 ), since the flash of an explosion is seen before its sound is heard.
The relationship between the speed of sound, its frequency, and wavelength is the same as for all waves:
where v is the speed of sound (in units of m/s), f is its frequency (in units of hertz), and λ λ is its wavelength (in units of meters). Recall that wavelength is defined as the distance between adjacent identical parts of a wave. The wavelength of a sound, therefore, is the distance between adjacent identical parts of a sound wave. Just as the distance between adjacent crests in a transverse wave is one wavelength, the distance between adjacent compressions in a sound wave is also one wavelength, as shown in Figure 14.7 . The frequency of a sound wave is the same as that of the source. For example, a tuning fork vibrating at a given frequency would produce sound waves that oscillate at that same frequency. The frequency of a sound is the number of waves that pass a point per unit time.
[BL] [OL] [AL] In musical instruments, shorter strings vibrate faster and hence produce sounds at higher pitches. Fret placements on instruments such as guitars, banjos, and mandolins, are mathematically determined to give the correct interval or change in pitch. When the string is pushed against the fret wire, the string is effectively shortened, changing its pitch. Ask students to experiment with strings of different lengths and observe how the pitch changes in each case.
One of the more important properties of sound is that its speed is nearly independent of frequency. If this were not the case, and high-frequency sounds traveled faster, for example, then the farther you were from a band in a football stadium, the more the sound from the low-pitch instruments would lag behind the high-pitch ones. But the music from all instruments arrives in cadence independent of distance, and so all frequencies must travel at nearly the same speed.
Recall that v = f λ v = f λ , and in a given medium under fixed temperature and humidity, v is constant. Therefore, the relationship between f and λ λ is inverse: The higher the frequency, the shorter the wavelength of a sound wave.
Teacher Demonstration
Hold a meter stick flat on a desktop, with about 80 cm sticking out over the edge of the desk. Make the meter stick vibrate by pulling the tip down and releasing, while holding the meter stick tight to the desktop. While it is vibrating, move the stick back onto the desktop, shortening the part that is sticking out. Students will see the shortening of the vibrating part of the meter stick, and hear the pitch or number of vibrations go up—an increase in frequency.
The speed of sound can change when sound travels from one medium to another. However, the frequency usually remains the same because it is like a driven oscillation and maintains the frequency of the original source. If v changes and f remains the same, then the wavelength λ λ must change. Since v = f λ v = f λ , the higher the speed of a sound, the greater its wavelength for a given frequency.
[AL] Ask students to predict what would happen if the speeds of sound in air varied by frequency.
Virtual Physics
This simulation lets you see sound waves. Adjust the frequency or amplitude (volume) and you can see and hear how the wave changes. Move the listener around and hear what she hears. Switch to the Two Source Interference tab or the Interference by Reflection tab to experiment with interference and reflection.
Tips For Success
Make sure to have audio enabled and set to Listener rather than Speaker, or else the sound will not vary as you move the listener around.
- Because, intensity of the sound wave changes with the frequency.
- Because, the speed of the sound wave changes when the frequency is changed.
- Because, loudness of the sound wave takes time to adjust after a change in frequency.
- Because it takes time for sound to reach the listener, so the listener perceives the new frequency of sound wave after a delay.
- Yes, the speed of propagation depends only on the frequency of the wave.
- Yes, the speed of propagation depends upon the wavelength of the wave, and wavelength changes as the frequency changes.
- No, the speed of propagation depends only on the wavelength of the wave.
- No, the speed of propagation is constant in a given medium; only the wavelength changes as the frequency changes.
Voice as a Sound Wave
In this lab you will observe the effects of blowing and speaking into a piece of paper in order to compare and contrast different sound waves.
- sheet of paper
Instructions
- Suspend a sheet of paper so that the top edge of the paper is fixed and the bottom edge is free to move. You could tape the top edge of the paper to the edge of a table, for example.
- Gently blow air near the edge of the bottom of the sheet and note how the sheet moves.
- Speak softly and then louder such that the sounds hit the edge of the bottom of the paper, and note how the sheet moves.
- Interpret the results.
Grasp Check
Which sound wave property increases when you are speaking more loudly than softly?
- amplitude of the wave
- frequency of the wave
- speed of the wave
- wavelength of the wave
Worked Example
What are the wavelengths of audible sounds.
Calculate the wavelengths of sounds at the extremes of the audible range, 20 and 20,000 Hz, in conditions where sound travels at 348.7 m/s.
To find wavelength from frequency, we can use v = f λ v = f λ .
(1) Identify the knowns. The values for v and f are given.
(2) Solve the relationship between speed, frequency and wavelength for λ λ .
(3) Enter the speed and the minimum frequency to give the maximum wavelength.
(4) Enter the speed and the maximum frequency to give the minimum wavelength.
Because the product of f multiplied by λ λ equals a constant velocity in unchanging conditions, the smaller f is, the larger λ λ must be, and vice versa. Note that you can also easily rearrange the same formula to find frequency or velocity.
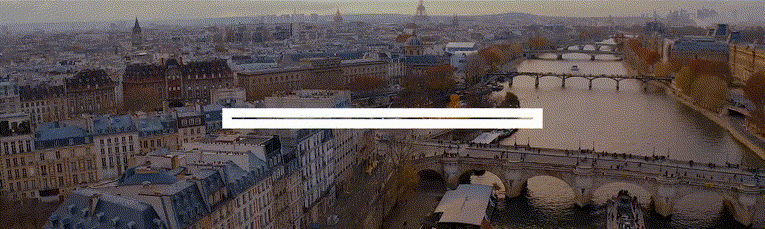
Practice Problems
- 5 × 10 3 m / s
- 3.2 × 10 2 m / s
- 2 × 10 − 4 m/s
- 8 × 10 2 m / s
- 2.0 × 10 7 m
- 1.5 × 10 7 m
- 1.4 × 10 2 m
- 7.4 × 10 − 3 m
Links To Physics
Echolocation.
Echolocation is the use of reflected sound waves to locate and identify objects. It is used by animals such as bats, dolphins and whales, and is also imitated by humans in SONAR—Sound Navigation and Ranging—and echolocation technology.
Bats, dolphins and whales use echolocation to navigate and find food in their environment. They locate an object (or obstacle) by emitting a sound and then sensing the reflected sound waves. Since the speed of sound in air is constant, the time it takes for the sound to travel to the object and back gives the animal a sense of the distance between itself and the object. This is called ranging . Figure 14.8 shows a bat using echolocation to sense distances.
Echolocating animals identify an object by comparing the relative intensity of the sound waves returning to each ear to figure out the angle at which the sound waves were reflected. This gives information about the direction, size and shape of the object. Since there is a slight distance in position between the two ears of an animal, the sound may return to one of the ears with a bit of a delay, which also provides information about the position of the object. For example, if a bear is directly to the right of a bat, the echo will return to the bat’s left ear later than to its right ear. If, however, the bear is directly ahead of the bat, the echo would return to both ears at the same time. For an animal without a sense of sight such as a bat, it is important to know where other animals are as well as what they are; their survival depends on it.
Principles of echolocation have been used to develop a variety of useful sensing technologies. SONAR, is used by submarines to detect objects underwater and measure water depth. Unlike animal echolocation, which relies on only one transmitter (a mouth) and two receivers (ears), manmade SONAR uses many transmitters and beams to get a more accurate reading of the environment. Radar technologies use the echo of radio waves to locate clouds and storm systems in weather forecasting, and to locate aircraft for air traffic control. Some new cars use echolocation technology to sense obstacles around the car, and warn the driver who may be about to hit something (or even to automatically parallel park). Echolocation technologies and training systems are being developed to help visually impaired people navigate their everyday environments.
- The echo would return to the left ear first.
- The echo would return to the right ear first.
Check Your Understanding
Use these questions to assess student achievement of the section’s Learning Objectives. If students are struggling with a specific objective, these questions will help identify which and direct students to the relevant content.
- Rarefaction is the high-pressure region created in a medium when a longitudinal wave passes through it.
- Rarefaction is the low-pressure region created in a medium when a longitudinal wave passes through it.
- Rarefaction is the highest point of amplitude of a sound wave.
- Rarefaction is the lowest point of amplitude of a sound wave.
What sort of motion do the particles of a medium experience when a sound wave passes through it?
- Simple harmonic motion
- Circular motion
- Random motion
- Translational motion
What does the speed of sound depend on?
- The wavelength of the wave
- The size of the medium
- The frequency of the wave
- The properties of the medium
What property of a gas would affect the speed of sound traveling through it?
- The volume of the gas
- The flammability of the gas
- The mass of the gas
- The compressibility of the gas
As an Amazon Associate we earn from qualifying purchases.
This book may not be used in the training of large language models or otherwise be ingested into large language models or generative AI offerings without OpenStax's permission.
Want to cite, share, or modify this book? This book uses the Creative Commons Attribution License and you must attribute Texas Education Agency (TEA). The original material is available at: https://www.texasgateway.org/book/tea-physics . Changes were made to the original material, including updates to art, structure, and other content updates.
Access for free at https://openstax.org/books/physics/pages/1-introduction
- Authors: Paul Peter Urone, Roger Hinrichs
- Publisher/website: OpenStax
- Book title: Physics
- Publication date: Mar 26, 2020
- Location: Houston, Texas
- Book URL: https://openstax.org/books/physics/pages/1-introduction
- Section URL: https://openstax.org/books/physics/pages/14-1-speed-of-sound-frequency-and-wavelength
© Jan 19, 2024 Texas Education Agency (TEA). The OpenStax name, OpenStax logo, OpenStax book covers, OpenStax CNX name, and OpenStax CNX logo are not subject to the Creative Commons license and may not be reproduced without the prior and express written consent of Rice University.
Want to create or adapt books like this? Learn more about how Pressbooks supports open publishing practices.
Traveling waves
17 How sound moves
Speed of sound.
There’s a delay between when a sound is created and when it is heard. In everyday life, the delay is usually too short to notice. However, the delay can be noticeable if the distance between source and detector is large enough. You see lightning before you hear the thunder. If you’ve sat in the outfield seats in a baseball stadium, you’ve experienced the delay between seeing the player hit the ball and the sound of the “whack.” Life experiences tell us that sound travels fast, but not nearly as fast as light does. Careful experiments confirm this idea.
The speed of sound in air is roughly 340 m/s. The actual value depends somewhat on the temperature and humidity. In everyday terms, sound travels about the length of three and a half foot ball fields every second- about 50% faster than a Boeing 747 (roughly 250 m/s). This may seem fast, but it’s tiny compared to light, which travels roughly a million times faster than sound (roughly 300,000,000 m/s).
Sound requires some material in which to propagate (i.e. travel). This material sound travels through is called the medium . You can show that sound requires a medium by putting a cell phone inside a glass jar connected to a vacuum pump. As the air is removed from the jar, the cell phone’s ringer gets quieter and quieter. A youTube video (2:05 min) produced by the UNSW PhysClips project shows the demo with a drumming toy monkey [1] instead of a cell phone.
What affects the speed of sound?
Sound travels at different speeds though different materials. The physical properties of the medium are the only factors that affect the speed of sound- nothing else matters.
The speed of sound in a material is determined mainly by two properties- the stiffness of the material and the density of the material. Sound travels fastest through materials that are stiff and light. In general, sound travels fastest through solids, slower through liquids and slowest through gasses. (See the table on this page). This may seem backwards- after all, metals are quite dense. However, the high density of metals is more than offset by far greater stiffness (compared to liquids and solids).
The speed of sound in air depends mainly on temperature. The speed of sound is 331 m/s in dry air at 0 o Celsius and increases slightly with temperature- about 0.6 m/s for every 1 o Celsius for temperatures commonly found on Earth. Though speed of sound in air also depends on humidity, the effect is tiny- sound travels only about 1 m/s faster in air with 100% humidity air at 20 o C than it does in completely dry air at the same temperature.
Nothing else matters
The properties of the medium are the only factors that affect the speed of sound- nothing else matters.
Frequency of the sound does not matter- high frequency sounds travel at the same speed as low frequency sounds. If you’ve ever listened to music, you’ve witnessed this- the low notes and the high notes that were made simultaneously reach you simultaneously, even if you are far from the stage. If you’ve heard someone shout from across a field, you’ve noticed that the entire shout sound (which contains many different frequencies at once) reaches you at the same time. If different frequencies traveled at different rates, some frequencies would arrive before others.
The amplitude of the sound does not matter- loud sounds and quiet ones travel at the same speed. Whisper or yell- it doesn’t matter. The sound still takes the same amount of time to reach the listener. You’ve probably heard that you can figure out how far away the lightning by counting the seconds between when you see lightning and hear thunder. If the speed of sound depended on loudness, this rule of thumb would have to account for loudness- yet there is nothing in the rule about loud vs. quiet thunder. The rule of thumb works the same for all thunder- regardless of loudness . That’s because the speed of sound doesn’t depend on amplitude.
Stop to thinks
- Which takes longer to cross a football field: the sound of a high pitched chirp emitted by a fruit bat or the (relatively) low pitched sound emitted by a trumpet?
- Which sound takes longer to travel 100 meters: the sound of a snapping twig in the forest or the sound of a gunshot?
- Which takes longer to travel the distance of a football field: the low pitched sound of a whale or the somewhat higher pitched sound of a human being?
Constant speed
Sound travels at a constant speed. Sound does not speed up or slow down as it travels (unless the properties of the material the sound is going through changes). I know what you’re thinking- how is that possible? Sounds die out as they travel, right? True. That means sounds must slow down and come to a stop, right? Wrong. As sound travels, its amplitude decreases- but that’s not the same thing as slowing down. Sound (in air) covers roughly 340 meters each and every second, even as its amplitude shrinks. Eventually, the amplitude gets small enough that the sound is undetectable. A sound’s amplitude shrinks as it travels, but its speed remains constant.
The basic equation for constant speed motion (shown below) applies to sound.
[latex]d=vt[/latex]
In this equation, [latex]d[/latex] represents the distance traveled by the sound, [latex]t[/latex] represents the amount of time it took to go that distance and [latex]v[/latex] represents the speed.
Rule of thumb for lightning example
Example: thunder and lightning.
The rule of thumb for figuring out how far away a lightning strike is from you is this:
Count the number of seconds between when you see the lightning and hear the thunder. Divide the number of seconds by five to find out how many miles away the lightning hit.
According to this rule, what is the speed of sound in air? How does the speed of sound implied by this rule compare to 340 m/s?
Identify important physics concept : This question is about speed of sound.
List known and unknown quantities (with letter names and units):
At first glance, it doesn’t look like there’s enough information to solve the problem. We were asked to find speed, but not given either a time or a distance. However, the problem does allow us to figure out a distance if we know the time- “Divide the number of seconds by five to find out how many miles away the lightning hit.” So, let’s make up a time and see what happens; if the time is 10 seconds, the rule of thumb says that the distance should be 2 miles.
[latex]v= \: ?[/latex]
[latex]d=2 \: miles[/latex]
[latex]t=10 \: seconds[/latex]
You might ask “Is making stuff up OK here?” The answer is YES! If the rule of thumb is right, it should work no matter what time we choose. (To check if the rule is OK, we should probably test it with more than just one distance-time combination, but we’ll assume the rule is OK for now).
Do the algebra: The equation is already solved for speed. Move on to the next step.
Do unit conversions (if needed) then plug in numbers: If you just plug in the numbers, the speed comes out in miles per second:
[latex]v = \frac{2 \: miles} {10 \: seconds}=0.2 \: \frac{miles} {second}[/latex]
We are asked to compare this speed to 340 m/s, so a unit conversion is in order; since there are 1609 meters in a mile:
[latex]v =0.2 \: \frac{miles} {second}*\frac{1609 \: meters} {1 \:mile}=320 \frac{m}{s}[/latex]
Reflect on the answer:
- The answer for speed from the rule of thumb is less than 10% off the actual value of roughly 340 m/s- surprisingly close!
- At the beginning, we assumed a time of 10 seconds. Does the result hold up for other choices? A quick check shows that it does! For instance, if we use a time of 5 seconds, the rule of thumb gives a distance of 1 mile, and the math still gives a speed of 0.2 miles/second. The speed will be the same no matter what time we pick. The reason is this: The more time it takes the thunder to arrive, the farther away the lightning strike is, but the speed remains the same. In the equation for speed, both time and distance change by the same factor and the overall fraction remains unchanged.
Stop to think answers
- Both sounds take the same amount of time. (High and low pitched sounds travel at the same speed).
- Both sounds take the same amount of time. (Quiet sounds and loud sounds travel at the same speed).
- The sound of the whale travels the distance in less time- assuming sound from the whale travels in water and sound from the human travels in air. Sound travels faster in water than in air. (The info about frequency was given just to throw you off- frequency doesn’t matter).
- Wolfe, J. (2014, February 20). Properties of Sound. Retrieved from https://www.youtube.com/watch?v=P8-govgAffY ↵
Understanding Sound Copyright © by dsa2gamba and abbottds is licensed under a Creative Commons Attribution 4.0 International License , except where otherwise noted.
Share This Book
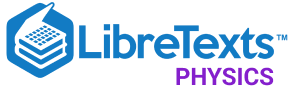
- school Campus Bookshelves
- menu_book Bookshelves
- perm_media Learning Objects
- login Login
- how_to_reg Request Instructor Account
- hub Instructor Commons
- Download Page (PDF)
- Download Full Book (PDF)
- Periodic Table
- Physics Constants
- Scientific Calculator
- Reference & Cite
- Tools expand_more
- Readability
selected template will load here
This action is not available.
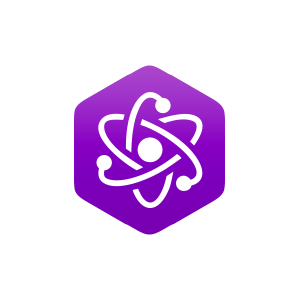
5.1.1: Speeds of Different Types of Waves
- Last updated
- Save as PDF
- Page ID 26167

- Kyle Forinash and Wolfgang Christian
The speed of a wave is fixed by the type of wave and the physical properties of the medium in which it travels. An exception is electromagnetic waves which can travel through a vacuum. For most substances the material will vibrate obeying a Hooke's law force as a wave passes through it and the speed will not depend on frequency. Electromagnetic waves in a vacuum and waves traveling though a linear medium are termed linear waves and have constant speed. Examples:
- For sound waves in a fluid (for example air or water) the speed is determined by \(v=(B/\rho )^{1/2}\) where \(B\) is the bulk modulus or compressibility of the fluid in newtons per meter squared and \(\rho\) is the density in kilograms per cubic meter.
- For sound waves in a solid the speed is determined by \(v= (Y/\rho )^{1/2}\) where \(Y\) is Young's modulus or stiffness in Newtons per meter squared and \(\rho\) is the density in kilograms per meter cubed.
- For waves on a string the speed is determined by \(v=(T/\mu )^{1/2}\) where \(T\) is the tension in the string in Newtons and \(\mu\) is the mass per length in kilograms per meter.
- Although electromagnetic waves do not need a medium to travel (they can travel through a vacuum) their speed in a vacuum, \(c = (1/\mu _{o} ε_{o})^{1/2} = 3.0\times 10^{8}\text{ m/s}\) is governed by two physical constants, the permeability \(\mu_{o}\) and the permittivity, \(ε_{o}\) of free space (vacuum).
Table \(\PageIndex{1}\)
Here is a more comprehensive list of the speed of sound in various materials .
As we saw in the previous chapter, there is a relationship between the period, wavelength and speed of the wave. The period of a cork floating in the water is affected by how fast the wave passes (wave speed) and the distance between peaks (wavelength). The relationship between speed, period and wavelength of a sine wave is given by \(v=\lambda /T\) where wavelength and period for a sine wave were defined previously. This can also be written as \(v=\lambda f\) since frequency is the inverse of period and is true for all linear waves. Notice that, since wave speed is normally a fixed quantity the frequency and wavelength will be inversely proportion; higher frequencies mean shorter wavelengths.
Often it is easier to write \(ω = 2πf\) where \(\omega\) is the angular frequency in radians per second instead of having to write \(2\pi f\) everywhere. Likewise it is easier to write \(k=2\pi /\lambda \) where \(k\) is the wave number in radians per meter rather than having to write \(2\pi /\lambda\) a lot. (Note that \(k\) is not a spring constant here.) Using these new definitions the speed of a wave can also be written as \(v=f\lambda =\omega /k\).
If the medium is uniform the speed of a wave is fixed and does not change. There are circumstances where the speed of a particular wave does change, however. Notice that the speed of sound in air depends on the density of the air (mass per volume). But the density of air changes with temperature and humidity. So the speed of sound can be different on different days and in different locations. The temperature dependence of the speed of sound in air is given by \(v = 344 + 0.6 (T - 20)\) in meters per second where \(T\) is the temperature in Celsius (\(T\) here is temperature, not period). Notice that at room temperature (\(20^{\circ}\text{C}\)) sound travels at \(344\text{ m/s}\).
The speed of sound can also be affected by the movement of the medium in which it travels. For example, wind can carry sound waves further (i.e. faster) if the sound is traveling in the same direction or it can slow the sound down if the sound is traveling in a direction opposite to the wind direction.
Electromagnetic waves travel at \(\text{c} = 3.0\times 10^{8}\text{ m/s}\) in a vacuum but slow down when they pass through a medium (for example light passing from air to glass). This occurs because the material has a different value for the permittivity and/or permeability due to the interaction of the wave with the atoms of the material. The amount the speed changes is given by the index of refraction \(n=c/v\) where \(c\) is the speed of light in a vacuum and \(v\) is the speed in the medium. The frequency of the wave does not change when it slows down so, since \(v=\lambda f\), the wavelength of electromagnetic waves in a medium must be slightly smaller.
Video/audio examples:
- What is the speed of sound in a vacuum? Buzzer in a bell jar . Why is there no sound when the air is removed from the jar?
- Demonstration of speed of sound in different gasses . Why is there no sound when the air is removed from the jar?
- These two videos demonstrate the Allasonic effect. The speed of sound is different in a liquid with air bubbles because the density is different. As the bubbles burst, the speed of sound changes, causing the frequency of sound waves in the liquid column to change, thus changing the pitch. Example: one , two . What do you hear in each case?
- The Zube Tube is a toy that has a spring inside attached to two plastic cups on either end. Vibrations in the spring travel at different speeds so a sound starting at one end (for example a click when you shake the tube and the spring hits the cup) ends up changing pitch at the other end as the various frequencies arrive. In other words this is a nonlinear system. See if you can figure out from the video which frequencies travel faster, high frequencies or low.
Mini-lab on measuring the speed of sound .
Questions on Wave Speed:
\(f=1/T,\quad v=f\lambda ,\quad v=\omega /k,\quad k=2\pi /\lambda,\quad \omega =2\pi f,\quad y(x,t)=A\cos (kx-\omega t+\phi ),\quad v=\sqrt{B/Q}\)
- Light travels at \(3.0\times 10^{8}\text{ m/s}\) but sound waves travel at about \(344\text{ m/s}\). What is the time delay for light and sound to arrive from a source that is \(10,000\text{ m}\) away (this can be used to get an approximate distance to a thunderstorm)?
- What two mistakes are made in science fiction movies where you see and hear an explosion in space at the same time?
- Consult the table for the speed of sound in various substances. If you have one ear in the water and one ear out while swimming in a lake and a bell is rung that is half way in the water some distance away, which ear hears the sound first?
- At \(20\text{C}\) the speed of sound is \(344\text{ m/s}\). How far does sound travel in \(1\text{ s}\)? How far does sound travel in \(60\text{ s}\)?
- Compare the last two answers with the distance traveled by light which has a speed of \(3.0\times 10^{8}\text{ m/s}\). Why do you see something happen before you hear it?
- The speed of sound in water is \(1482\text{ m/s}\). How far does sound travel under water in \(1\text{ s}\)? How far does sound travel under water in \(60\text{ s}\)?
- What happens to the speed of sound in air as temperature increases?
- Using the equation for the speed of sound at different temperatures, what is the speed of sound on a hot day when the temperature is \(30^{\circ}\text{C}\)? Hint: \(v = 344\text{ m/s} + 0.6 (T - 20)\) where \(T\) is the temperature in Celsius.
- Using the speed of sound at \(30^{\circ}\text{C}\) from the last question, recalculate the distance traveled for the cases in question four.
- Suppose on a cold day the temperature is \(-10^{\circ}\text{C}\: (14^{\circ}\text{F}\)). You are playing in the marching band outside. How long does it take the sound from the band to reach the spectators if they are \(100\text{ m}\) away?
- What is the difference in the speed of sound in air on a hot day (\(40^{\circ}\text{C}\)) and a cold day (\(0^{\circ}\text{C}\))?
- What would an orchestra sound like if different instruments produced sounds that traveled at different speeds?
- The speed of a wave is fixed by the medium it travels in so, for a given situation, is usually constant. What happens to the frequency of a wave if the wavelength is doubled?
- What happens to the wavelength of a wave if the frequency is doubled and has the same speed?
- Suppose a sound wave has a frequency \(200\text{ Hz}\). If the speed of sound is \(343\text{ m/s}\), what wavelength is this wave?
- What factors determine the speed of sound in air?
- Why do sound waves travel faster through liquids than air?
- Why do sound waves travel faster through solids than liquids?
- The speed of sound in a fluid is given by \(v=\sqrt{B/Q}\) where \(B\) is the Bulk Modulus (compressibility) and \(Q\) is the density. What happens to the speed if the density of the fluid increases?
- What must be true about the compressibility, \(B\), of water versus air, given that sound travels faster in water and water is denser than air?
- The speed of sound in a fluid is given by \(v=\sqrt{B/Q}\) where \(B\) is the Bulk Modulus (compressibility) and \(Q\) is the density. Can you think of a clever way to measure the Bulk Modulus of a fluid if you had an easy way to measure the speed of sound in a fluid? Explain.
- The speed of sound on a string is given by \(v=\sqrt{T/\mu}\) where \(T\) is the tension in Newtons and \(\mu\) is the linear density (thickness) in \(\text{kg/m}\). You also know that \(v=f\lambda\). Give two ways of changing the frequency of vibration of a guitar string based on the knowledge of these two equations.
- For the previous question, increasing the tension does what to the frequency? What does using a denser string do to the frequency?
- The following graph is of a wave, frozen in time at \(t = 0\). The equation describing the wave is \(y(x,t)=A\cos (kx-\omega t+\phi )\). Sketch the effect of doubling the amplitude, \(A\).
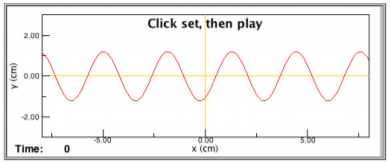
Figure \(\PageIndex{1}\)
- For the following graph of a wave, sketch the effect of doubling the wavelength.
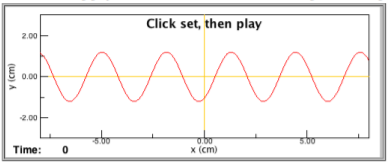
Figure \(\PageIndex{2}\)
- The mathematical description of a sine wave is given by \(y(x,t)=A\cos (kx-\omega t+\phi )\). Explain what each of the terms \((A, k, \omega, \phi )\) represent.
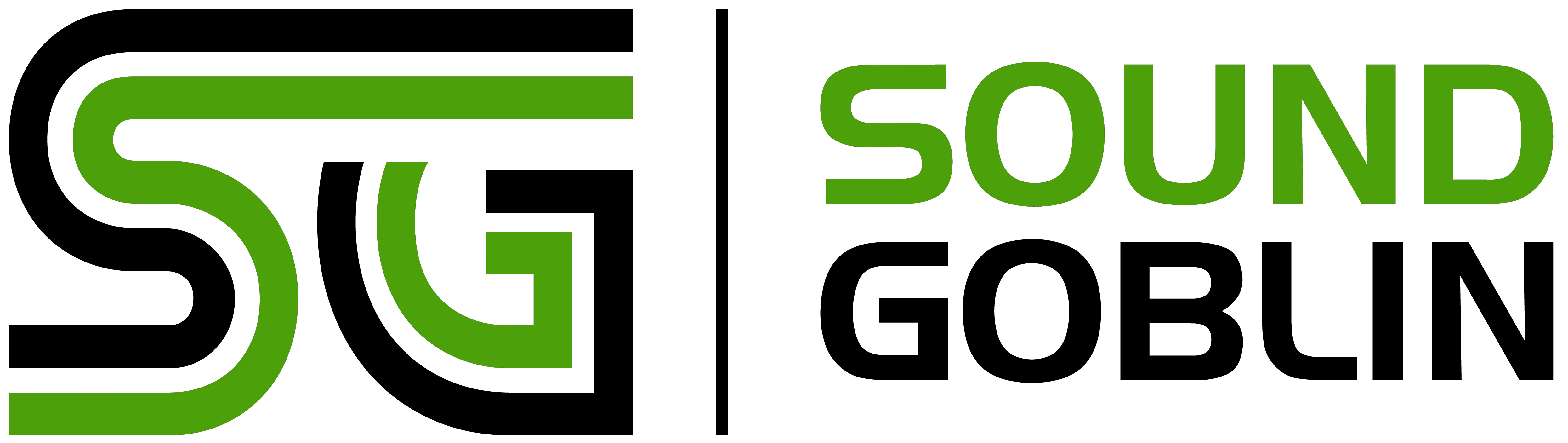
How Sound Travels Through Solids, Liquids and Gases
- August 13, 2022
We love to work with sound. Many of us record our own music, podcast, or other forms of sound. Knowing how sound travels through different mediums will allow you to have better control over the sound that you generate. That is what we will be looking at today. How does sound travel through solids, liquids, and gases?
Can the way that you produce sound and the medium that it moves in make a difference in the volume that you will hear? How does this change when it comes to the different mediums? Will the furniture in a room have any impact on the acoustics of the room? How can you change it to create the perfect recording environment?
These are just some of the things that we will discuss today. Knowing how sound travels through solids, liquids and gases are not only interesting, but it can have an impact on the way we record sounds and how we change things up.
Why Is the Way That Sound Travels Through Mediums Important?
One of the main reasons why it is important to understand how sound acts, is that when you understand something better, you can control it. As a youngster, I loved swimming. I still do. But one of my main attractions was that underneath the water it was the one place where everything went quiet. It always felt like the world stopped and it was just me and complete calm.
I loved my family but there was always so much going on that it was just a great place to just be with my own imagination and thoughts. I could make up imaginary worlds and people, and have millions of stories running through my mind. All because there was a lack of sound under the water.
But in reality, when you look at how sound behaves in a liquid, scientifically, this should not be the case. In fact, there should be more sound under the water than there is in the fresh air. Why? And why doesn’t it work that way? I wanted to find out.
For many of us who record sound, it is important to be connected to it. If you understand what makes something sound fuller, what makes a noise loud, and how things act, you can have better control and your recording will end up being closer to what you intended in the first place.
You might be recording a podcast, but for some reason, your voice keeps sounding muffled, understanding sound can help you identify what the cause could be and how you can fix it.
What Is Sound?
To understand why sound acts differently in different mediums we first have to understand what sound is.
First, you need to know that sound can not exist in a void. This is different from light that does travel through nothingness. That is why we can see light shining from space where there is a void and no atmosphere. But those that have been to space say it is completely quiet. It must be an almost eerie feeling.
Sound happens when something creates a vibration. This is done through musical instruments, our voices, speakers, and many other things. This then causes the medium around it, like the water or the air, to also vibrate and carry this sound with them. Without a medium, sound would not exist. That is because the molecules of the medium react and bump into those next to it and this allows the sound to travel on.
At the same time, the medium that is used will determine just how loud the sound will be, it will also determine how far can travel and how the sound will generally react. This is because different solutions will have molecules that are more or less densely packed.
Your surroundings will have a big impact. People who create a sound studio try to make the acoustics of the room as powerful as possible. This should help you do that.
Let us now look at the three mediums that sound can travel through, solids, liquids, and gases, and how they change the reaction of the sound.
First up we can look at gasses. You might wonder why gasses are mentioned when speaking of mediums that sound can move in. You may be visualizing a bunch of fog at a concert that makes the lights look incredible and makes the crowd go wild.
And that is one possibility of gasses that can be used as a medium for sound to travel through. But most of the time, our air is the only gas that sound needs to continue its vibration.
What Is the Air Made Of?
We have already mentioned that sound can not exist in a void. But we can hear each other when we speak out in the open. We can hear music when it is being played under the starry sky and we can even hear kids shouting in a park a block away .
That is because most of the air in our atmosphere is made up of gasses. Our atmosphere is not just a void, or we wouldn’t be able to live here anyway, but is made up of lots of gasses we can’t see. The atmosphere is made up of 78% Nitrogen, and 21% Oxygen, and the rest is a mixture of carbon dioxide, neon, and hydrogen.
This gives us all the ability to breathe without needing a space suit, but it also gives sound the ability to travel in our atmosphere. We make a vibration and the molecules of the gases that we can’t even see start to bump into each other and takes that vibration further, making it possible for us to hear sounds. It is pretty amazing when you think about it.
How Does Sound Travel Through Gases?
Gas is the medium that will have the slowest speed of sound of all of them. This is because the molecules of the gases surrounding us are expanded and far away from each other. The vibrations do get passed over to each other but it takes longer to do.
This is also why we often need things that can amplify our sounds like a microphone when we are speaking to a bigger group of people. These help us to make the vibrations bigger and to allow them to travel further than we would have been able to achieve with only our voice.
Some Things That Can Influence Sound in Gases
Have you ever felt that things are so much quieter after a big snowstorm? How the world seems almost different then? Turns out it might not just be your imagination. This is because the volume and speed of sound can be impacted by the temperature of the air and in turn the gas that is surrounding us.
At lower temperatures, the molecules move around quicker and they can vibrate quicker. The energy behind the sound can start to be lost and the sound will become quieter or be lost faster.
At normal room temperatures, the speed of sound will be a lot higher than it would be in the exact same room when the temperature is at freezing.
There are many different liquids that have a higher or lower density but for the most part, it is in water where we would be interested in hearing a sound. If we go swimming or put a small portable speaker close to the water, we would like to hear the sound as loud as possible. But it just doesn’t always work like that.
Let’s see how sound reacts in water or other liquids.
Sound In Water
The molecules in water are a lot more tightly packed than it is in gas. That is why sound travels much faster in water than it would travel in the air. Sound can actually travel in water almost four times faster than it can be in the air.
That is really impressive. And still, if you submerge your head underwater, you will hear the sound but it might sound muffled and not quite like the sound that you are used to.
Why Humans Hear Muffled Sounds in Water
The water molecules are more tightly packed and the energy that it uses to carry sound is transported faster. In theory, you should be able to hear noises a lot louder when you are underwater. But that is not how we perceive this sound.
This is because our ears are created to listen to sounds in the air. We pick up on sounds through our ear canal and these sounds are then transported to the brain that makes sense of it all. When you only submerge your ears, sounds will sound very muffled since the ears can’t take these sounds along the ear canal.
When you submerge your head fully suddenly the sound is clearer and louder. Although it could still be somewhat muffled compared to outside the water. Our heads contain a lot of water, and inside the water, it will be our tissue that picks up on the sound and detects it.
You could try to plug your ears but it will have very little effect on the volume of the noise under the water. The sound is not traveling along those normal lines.
At the same time, chances are that it is also very hard and almost impossible for you to figure out from which direction the sound is coming. When the sound travels along the normal route our brain has cues to determine if it comes from behind us or in front. But when the sound does not travel in those normal routes the brain has no way of telling us where it is coming from.
For humans communicating through sound under the water is not so easy. That is why divers have always used hand signals to communicate with their diving partners and why some have even started to use microphones that connect them. Allowing for a much better communication route.
We know that we can’t hear sound in the same way when we are inside water as when we are in the air. But what happens when we make a big sound inside the water, like shouting? Will someone that is on the outside be able to hear it clearly?
This is unlikely. That is because the surface of the water almost acts as a mirror for sound. Instead of the vibration moving outside of the water it gets reflected back. Making sure that very little sound is heard outside.
Animals In Water
Our ears might be designed to hear in air, but fish and mammals that live in the ocean can take advantage of the speed of sound inside the water. They are adapted to hearing noise completely clearly inside the water.
Since sound does travel quickly in water and they can hear it, they can use sounds to communicate over much larger distances than we are able to do with just our voice. Whales, for example, have been known to use their voice to communicate with other whales over massive distances in the ocean. The sound of a humpback whale can travel thousands of miles in the ocean. It also helps that the vibrations they can create are much larger than the ones our own vocal cords can produce.
Then finally there are solids and how sound reacts when they come into contact with a solid. Since sound starts to get muffled when there are a lot of solid objects in its path you would think that it travels a lot slower in solids. But surprisingly that is not the case. There are however reasons why it reacts in this way.
The Speed of Sound in Solids
A solid object is densely packed with its molecules. Each solid object will be a little bit different from the other depending on the material it is made of and how densely packed its molecules are. There are some materials that will work better as insolation to noise than others, but we will discuss the reason for this shortly. But for the most part, sound will travel a lot faster in solids than it will in both liquids and gasses.
This is because the source of the sound will create the vibration in the molecules of the sound and then these tightly packed molecules will quickly send the vibration further along. This means that the speed of sound is a lot faster when traveling in a solid object and that it will be a lot louder too.
Often a solid object will be a good source of amplification for a sound that you would like to enhance. The sound through a brass bugle gets enhanced through the design of the object and also through the material it is made of.
Examples Of Sounds in Solids
It can be hard to think of examples where solid objects are used to move sound and make it louder. Let’s discuss some simple examples of this.
You can put an ear to a solid object like a table and then make a soft tapping sound on the table. Compare how you heard it when your ear is on the solid compared to how loud it is when you hear the sound through the air. You will be surprised by how clearly the sound is enhanced by listening to it through a solid object.
Another great example of an experiment that many of us probably unknowingly did as children is a string telephone. You take two cups and a long line of string. The two cups are each connected to one side of the string, one person listens into one cup while another speaks into the cup at their end.
In this experiment, the vibrations are created and enhanced by the shape of the cup. Then these vibrations are transferred with the help of a solid object, the string, and the other person can hear your message at the other end of the string. Without raising your voice or shouting.
It is always amazing to see just how far this simple design can carry sound. Fun fact, the world record for the longest-ever string telephone, which was made with tin cans, was a whopping 796 feet long. That is almost the distance of three football fields. That is a long way for a piece of string and two cups to carry sound.
Then another great example of a sound being a lot louder when it is carried through a solid object is sounds that you can hear in the air. For example, hearing the sound of a horse coming closer, its hooves beating down on the ground.
It is already a pretty impressive sound when you hear it in normal circumstances. But try putting your head to the ground and listening to the approach in that way. The sound is much louder and you can almost feel the vibrations that are making the sound you hear.
Why Does Sound Get Muffled Through a Door?
We know now that sound travels much faster through solid objects than it does through gasses or liquids. You would think that a solid object like a wall or a door will enhance the sound but the opposite is true. A sound that is coming from a different room is more muffled.
If there is a lot of noise outside your home, for example, the neighbors having a party, it works to close the doors and windows and the sound won’t bother you as much. Even if you only have standard windows and doors.
How does that work? It works because the sound you are hearing does not originate from inside a solid object. It traveled through the air until it came to your door. There it encountered a solid object. And instead of making the vibrations louder this change in medium made the sound lose some of the energy that it was traveling with. This reduces the level of the noise and makes it less noticeable when there are doors that are closed.
Why Rooms Echo
This change in energy is also one of the reasons why a room will or won’t echo. When you go into an empty room there is a good chance that you can create an echo. That is because the empty room has no solid objects that break the energy of the noise down and stop it.
The vibrations bump only against the walls and reflect back. If you have a room that is still echoing even after your furniture has been installed, then there might not be enough solid objects that stop the speed and the energy of the sound. Something like a carpet that can absorb the vibration can help to stop the echo in the room.
How Sound Travels Through Solids, Liquids, and Gases
Sound needs a medium that can take the vibrations and move them along, allowing us to hear the sounds that are being created.
When it comes to the speed of sound, a solid object will allow the vibration to move much faster since it has the most densely packed molecules. It will also make the sound the loudest. After solids, liquids have the highest speed of sound. And then finally gas, that included our air since it is made up of gasses.
When a sound is traveling through one medium like air and then encounters another, like a solid door, it loses some of its energy and some of the volume will be lost. That is why solid insulation against sound is still one of the best options despite solids being a good conductor of sound.
We might not be able to take full advantage of the high speed of sound that can be found inside a liquid, but those living in the ocean sure can and that is why whale sounds can travel thousands of miles under the water.
Knowing how sound reacts to different mediums will allow us to understand it better. And that means that you should have better control over your recordings and all the ways that you like to create your own very unique sounds.
Leave a Reply Cancel Reply
Add Comment
Save my name, email, and website in this browser for the next time I comment.
Post Comment

How Far Does Sound Travel: The Science of Acoustics
Do you ever stop to think about how sound travels? It’s an interesting phenomenon that occurs everyday and yet we often take it for granted. In this blog post, we will explore the science of acoustics and how sound travels. We will answer the question of how far sound can travel and how it is affected by different factors. Stay tuned for an in-depth look at this fascinating topic!

Nature Of Sound
Sound is a mechanical wave that is an oscillation of pressure transmitted through some medium, such as air or water. Sound can propagate through solids and liquids better than gases because the density and stiffness are greater. So how far does sound travel? In this article we will answer how sound travels and how to calculate how far it travels in different scenarios.
Sound Transmits Conception
A common misconception with regard to how sound transmits itself between two points (for example from speaker to ear) is that the source creates waves of compression in the surrounding gas which then proceed on their way at a constant speed until they strike something else; either another solid object or our ears . This analogy might be okay for describing what goes on at low frequencies but once we go beyond around 1000 Hz, the propagation of sound becomes far more complex.

At low frequencies (below around 1000 Hz), sound waves tend to travel in all directions more or less equally and bounce off objects like a rubber ball would. As frequency increases however, the directivity of sound increases as well. So high-frequency sounds are more likely to travel in a straight line between two points than low frequencies. This is why we can often hear someone calling from some distance away when there is loud music playing – because the higher frequencies carry further than the lower ones.
How Far Can Sound Travel
There are three ways that sound can be transmitted: through air, through water, or through solids. The speed of sound through each medium is different and depends on the density and stiffness of the material.

The speed of sound through air is about 343 m/s (or 760 mph), and it travels faster in warmer air than colder air. The speed of sound through water is about 1500 m/s, and it travels faster in salt water than fresh water. The speed of sound through solids is much faster than through either gases or liquids – about 5000-15000 m/s. This is why we can often hear someone coming before we see them – the sound waves are travelling through the solid ground to our ears!
Now that we know how sound propagates and how its speed depends on the medium, let’s take a look at how to calculate how far it will travel between two points. We can use the equation
distance = speed x time
For example, if we want to know how far a sound will travel in one second, we have:
distance = 343 m/s x 0.001 s = 343 m
So sound travels 1 kilometer in roughly 3 seconds and 1 mile in roughly 5 seconds.
Does Вecibel Level affect the Sound Distance?
The surface area around a sound source’s location grows with the square of the distance from the source. This implies that the same amount of sound energy is dispersed over a larger surface, and that the energy intensity decreases as the square of the distance from the source (Inverse Square Law).
Experts of Acoustical control says, that
For every doubling of distance, the sound level reduces by 6 decibels (dB), (e.g. moving from 10 to 20 metres away from a sound source). But the next 6dB reduction means moving from 20 to 40 metres, then from 40 to 80 metres for a further 6dB reduction.
How Far Can Sound Travel In Real World
In real world, there are many factors that can affect how far a sound travels. Factors such as air density, temperature and humidity have an impact on its propagation; obstacles like buildings or mountains could also block some frequencies from going through while letting others pass (this happens because at high frequencies they behave more like waves).
Sounds can propagate through solids better than they can propagate through air because their density/stiffness are greater (this means that sound travels faster). In addition to this, we also know that it takes less time for a high frequency wave to reach us from its source compared with low frequencies. For example if there’s some kind of obstacle blocking our path then it might take longer for waves at higher frequencies than those below 1000 Hz to past them.
Can Sound Waves Travel Infinitely?
No. The higher the frequency of a sound wave, the shorter its wavelength becomes. As wavelength decreases, the amount of energy in a sound wave also decreases and eventually it dissipates completely. This is why we often can’t hear someone calling from very far away when there’s loud music playing – because the high frequencies are being blocked out by all the noise!
Can Sound Travel 20 Miles?
The air may be permeable to these lower-frequency, sub-audible sound waves generated by elephants. Some whale species’ frequencies might travel through seawater for 1500 kilometers or 900 miles.
How Far Can a Human Scream Travel?
The normal intelligible outdoor range of the male human voice in still air is 180 m (590 ft 6.6 in).

The Guiness World Record of the Farthest distance travelled by a human voice belongs the Spanish-speaking inhabitants of the Canary Island of La Gomera, is intelligible under ideal conditions at 8 km (5 miles).
In Conclusion
At the end of this blog post, you should have a better understanding of how sound travel and what factors affect it. If you want to learn more about acoustics and sounds, you can check out our resources here.
- Why PS4 Is So Loud and How to Fix It
- Best Quiet Electric Toothbrush – Buyer’s Guide
Ocean Noise Pollution: What Is It?
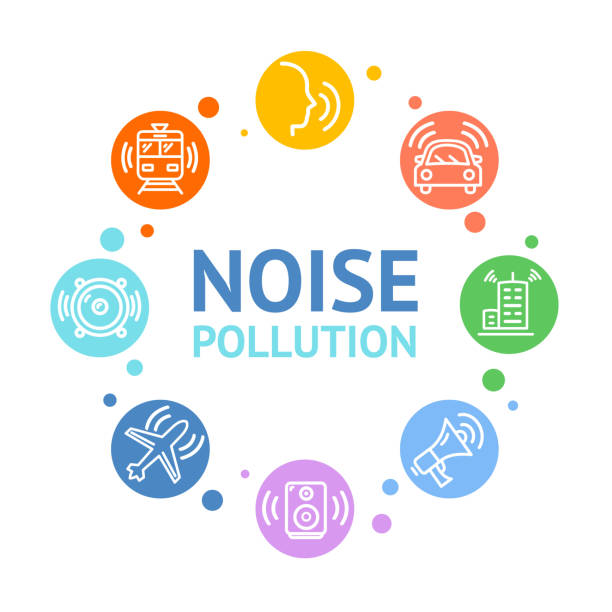
Ways to Reduce Noise Pollution in Your Life

Top 8 Organizations Against Noise Pollution
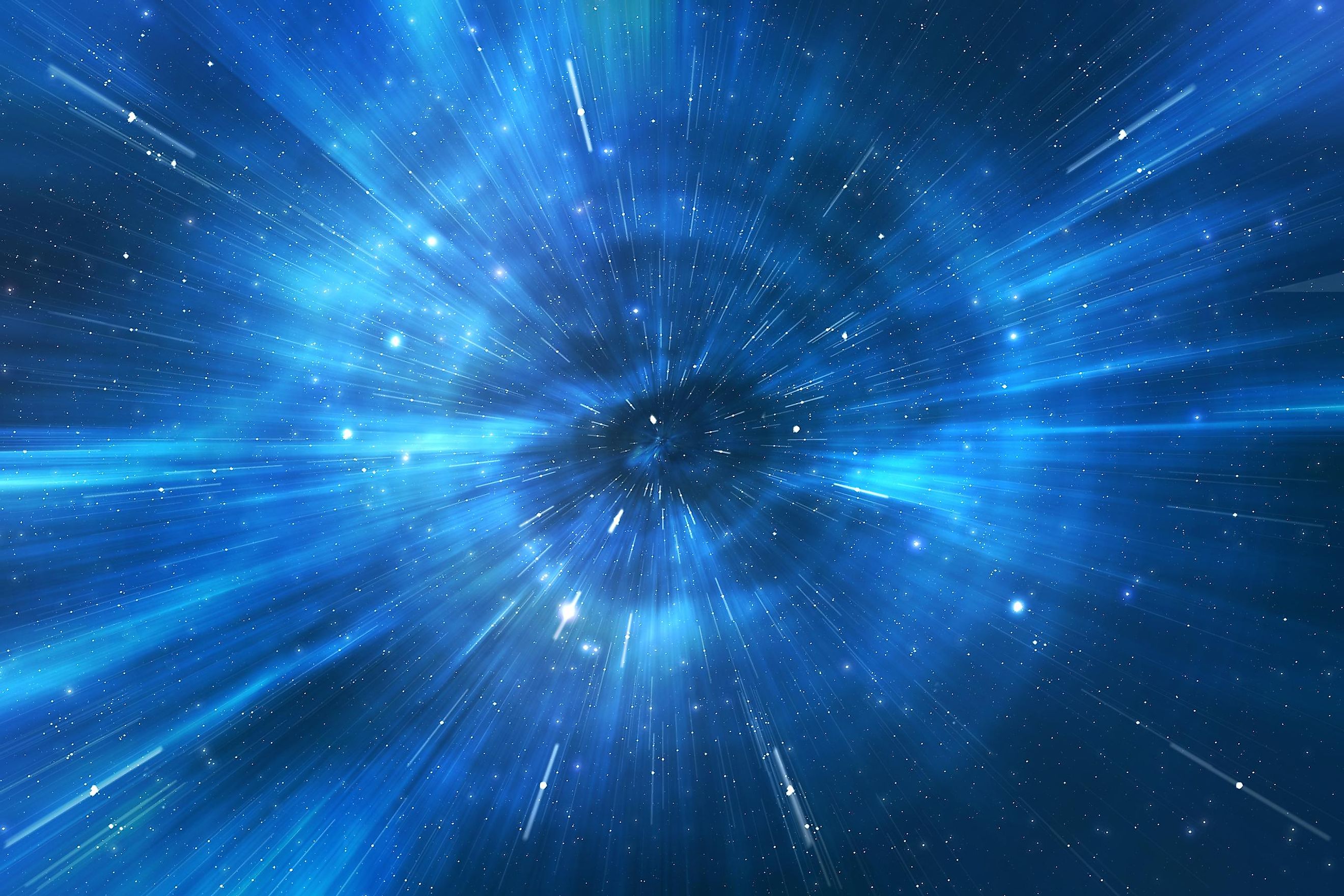
- Speed Of Sound vs Speed Of Light
The speed of sound and the speed of light are two common concepts that most of us likely learn about at some point in life. Both are defined rather simply, with the speed of sound being the speed that sound travels, and the speed of light being the speed at which light travels. Although these two concepts may sound similar, they are in fact radically different from one another. What are some of the differences between them?
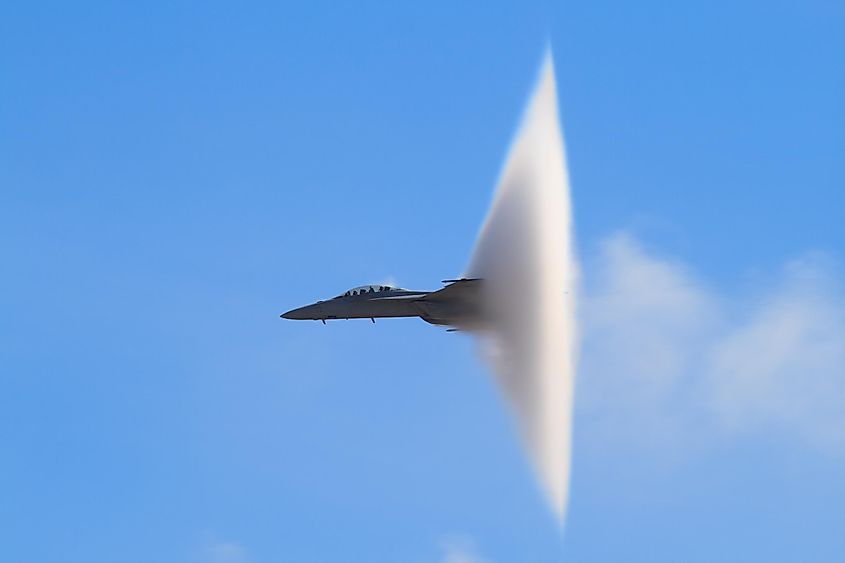
The first notable difference between the speed of sound and light is how fast they are. In Earth’s atmosphere , the speed of sound averages at about 761-miles per hour (1,225-kilometres per hour). That may seem fast, yet when compared to the speed of light, it seems quite small. Light travels at a staggering 670-million miles per hour (1.07-billion kilometres per hour). That’s around 880,000 times faster than the speed of sound.
Sound Must Have A Medium
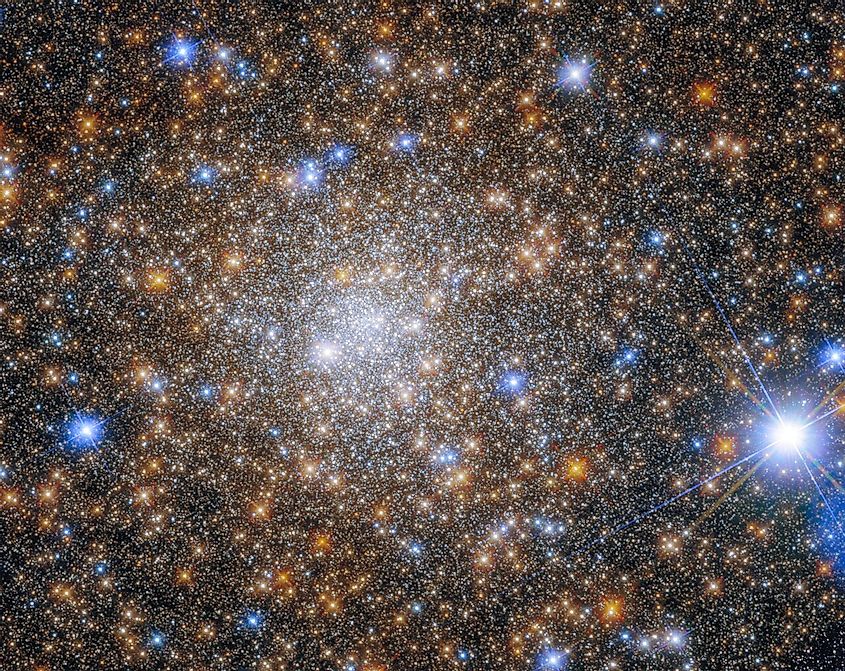
In order for sound to exist, it must have a medium to travel through. For sound, this medium is air, and that’s why sound does not exist in the emptiness of space. Meanwhile, light does not need a medium to exist. Rather, light travels independently of any medium, and thus it can travel through space, unlike sound.
The Speed Of Light Is Constant
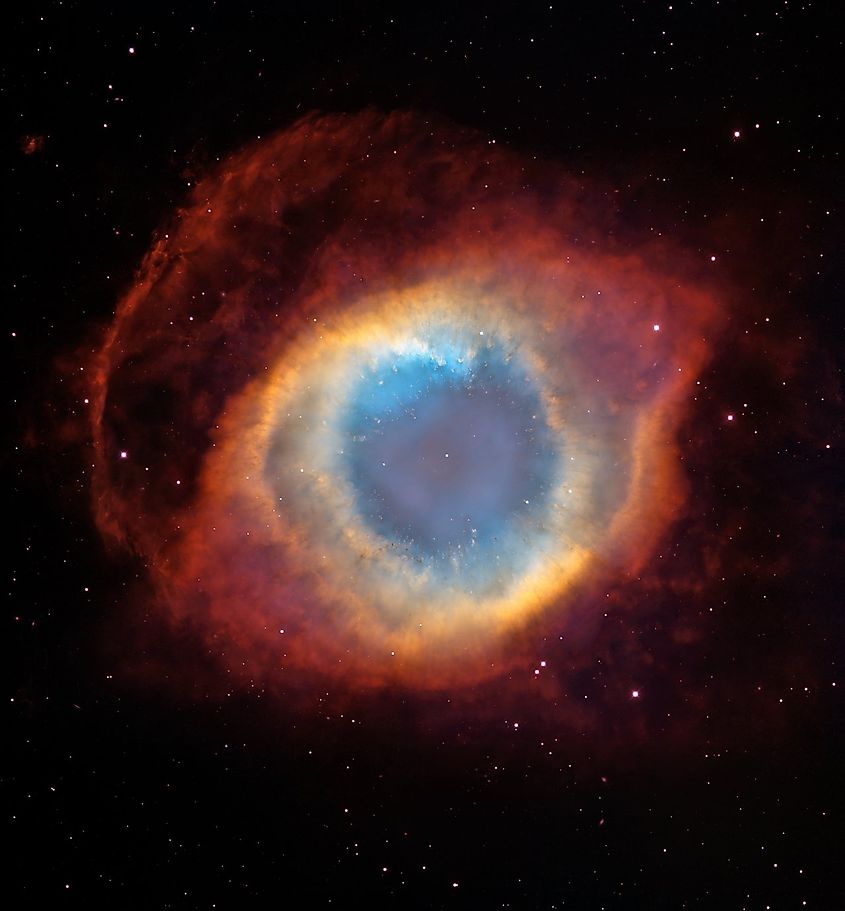
Another notable difference between light and sound is that the speed of light is constant while the speed of sound is not. Since the speed of sound requires air to exist, its speed is dependent upon the density and temperature of air. For example, when we say that sound travels at 761-miles per hour, this is only the case at sea level and when temperatures are around 59-degrees Fahrenheit (15-degrees Celsius). If you are at a higher elevation where the density of air is lower and temperatures are colder, the speed of sound will be different. Thus, the speed of sound varies. Light, however, has no such constraint. Rather, the speed of light is constant regardless of any other factor. No matter the conditions, it always travels at the same speed.
Things That Can Move Faster Than Sound
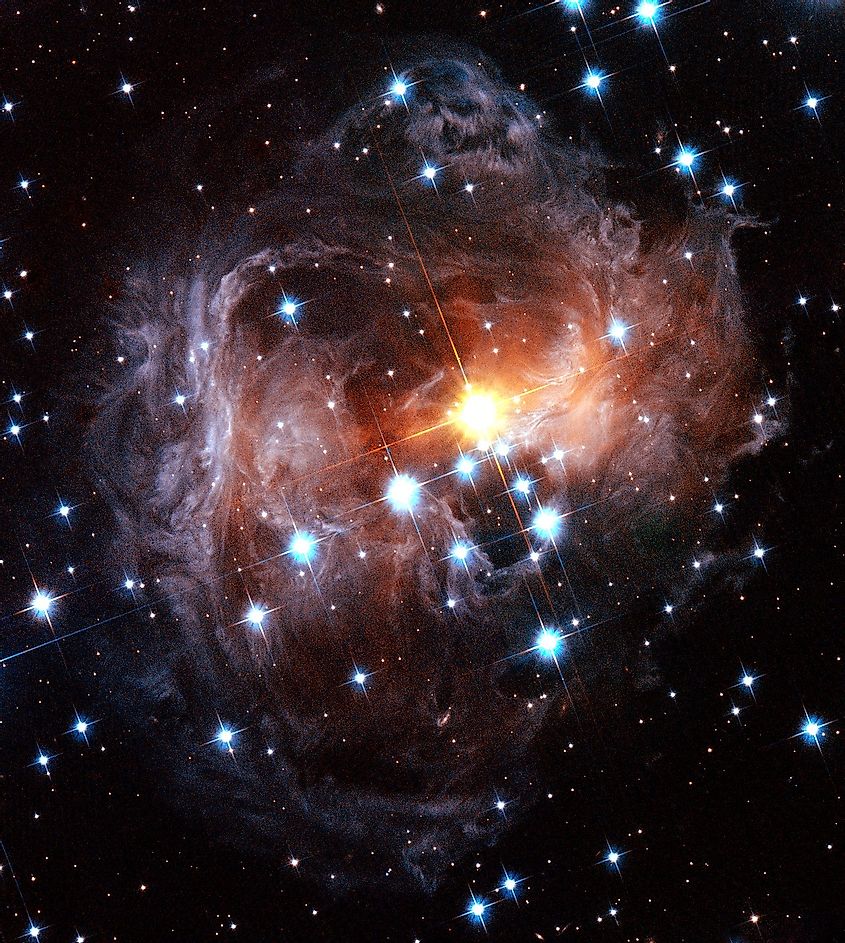
The speed of sound is fast, yet it is by no means the fastest thing in the universe. It is not even the fastest thing on Earth . Human’s have broken the sound barrier countless times, a speed known as supersonic speed. Light is a different story, however. The speed of light is a fixed law of nature, and it represents the fastest possible speed anything can move at. No matter how hard we try or how advanced technology becomes, the current understanding of the cosmos is that the light barrier cannot be broken. Thus, the speed of light is like a cosmic speed limit.
More in Science
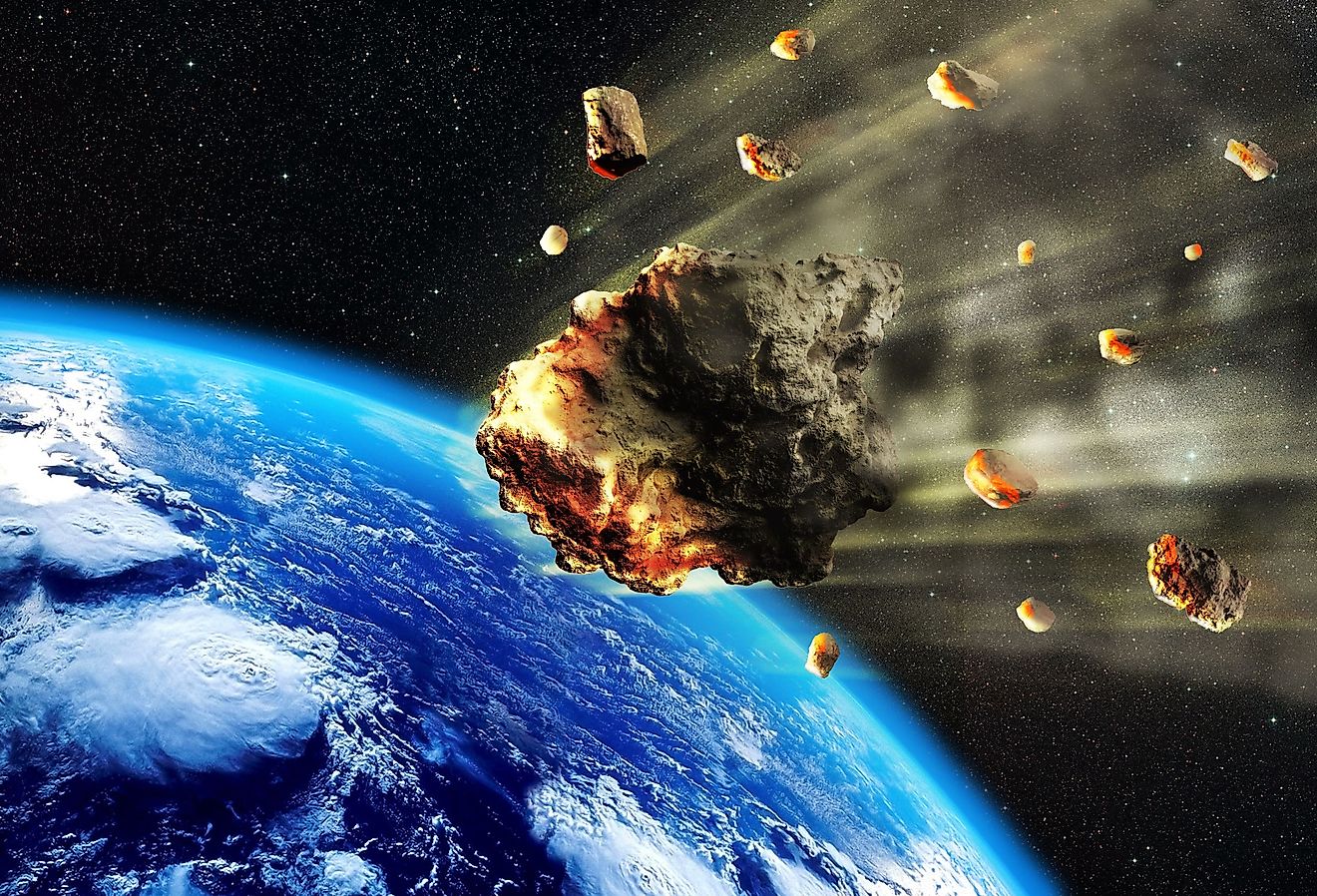
Where Do Most Earthquakes Occur In The US?
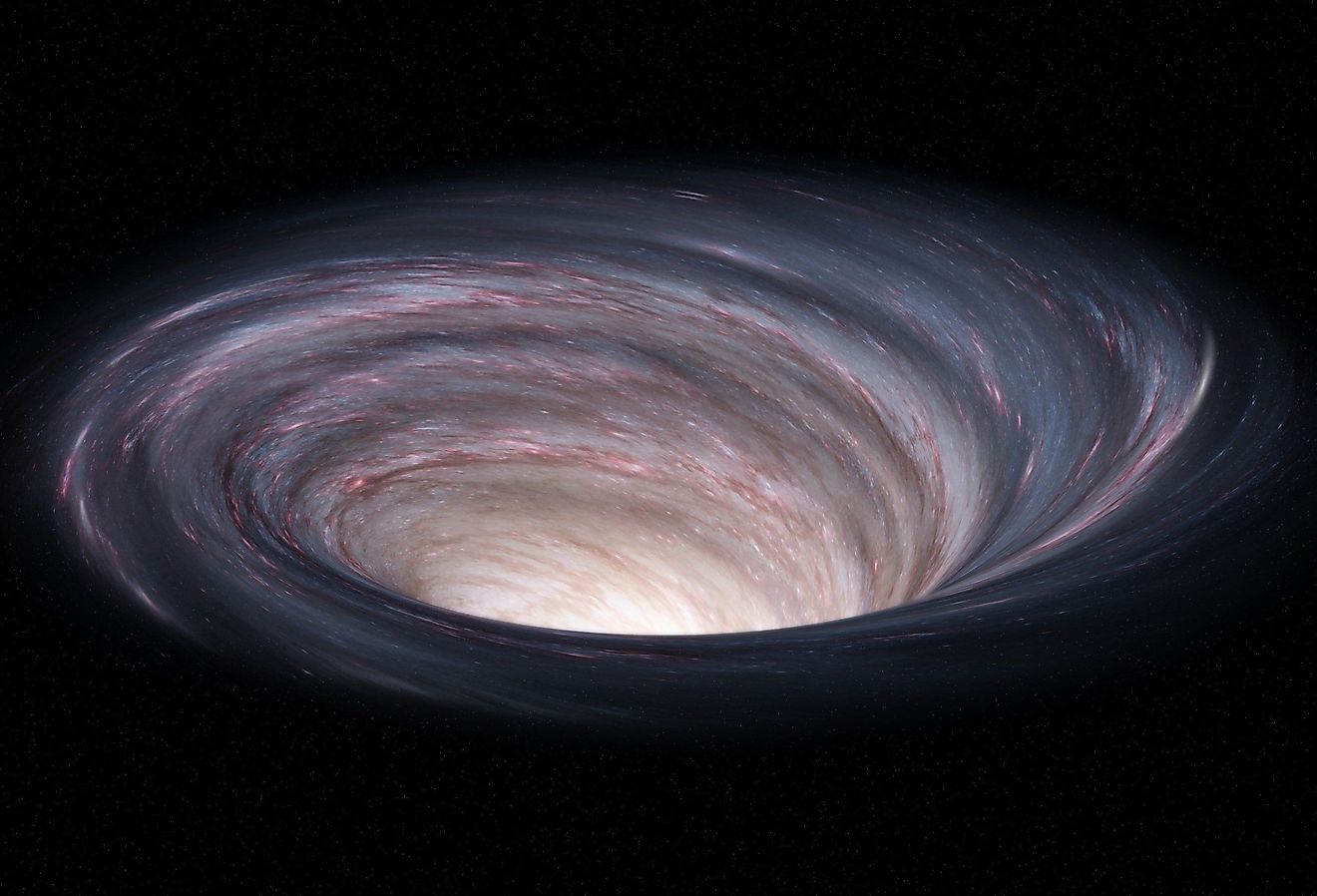
Black Holes Might be Defects in Space and Time
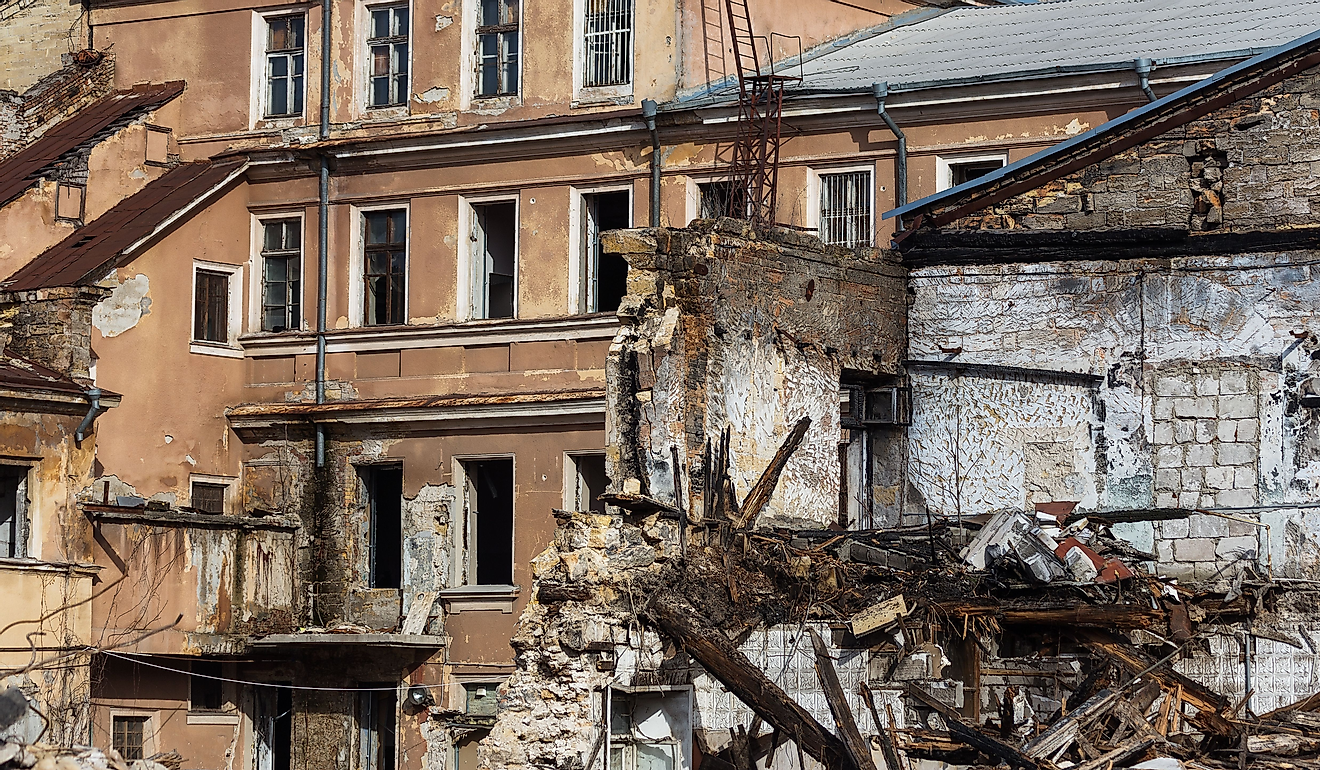
How Are Earthquakes Measured Using The Richter Scale?
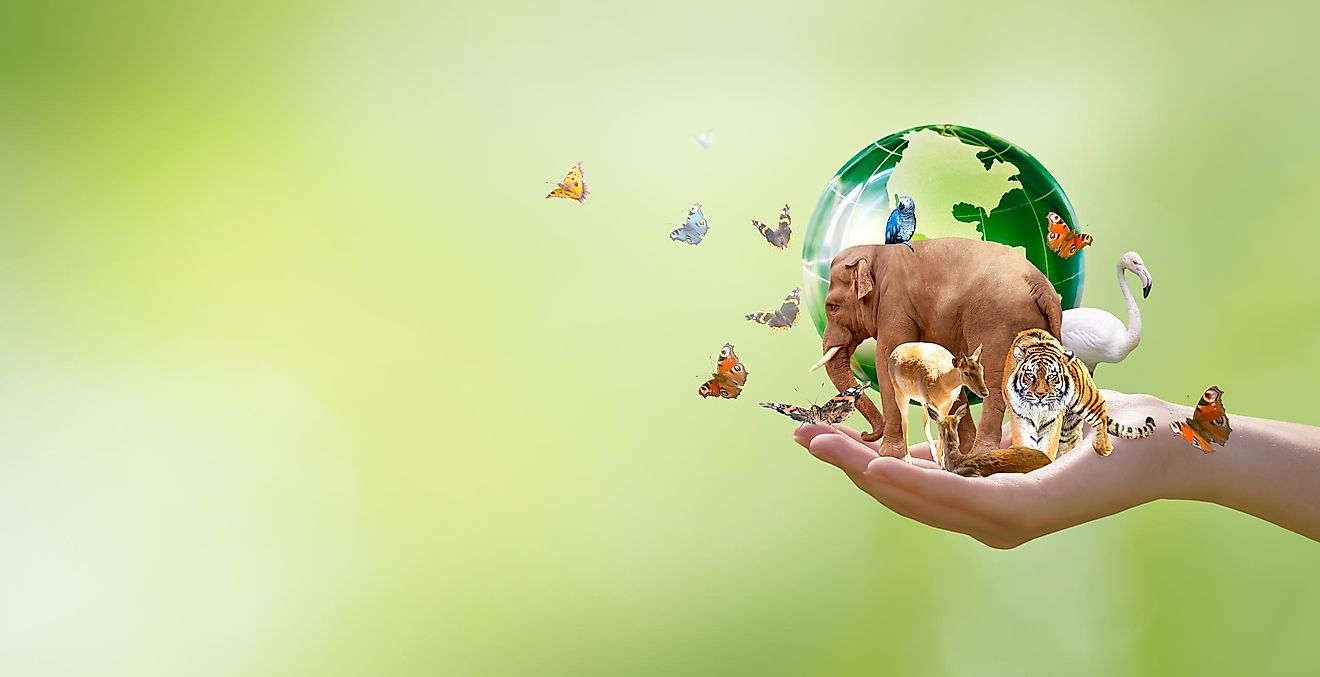
How Many New Species Are Discovered Every Year?
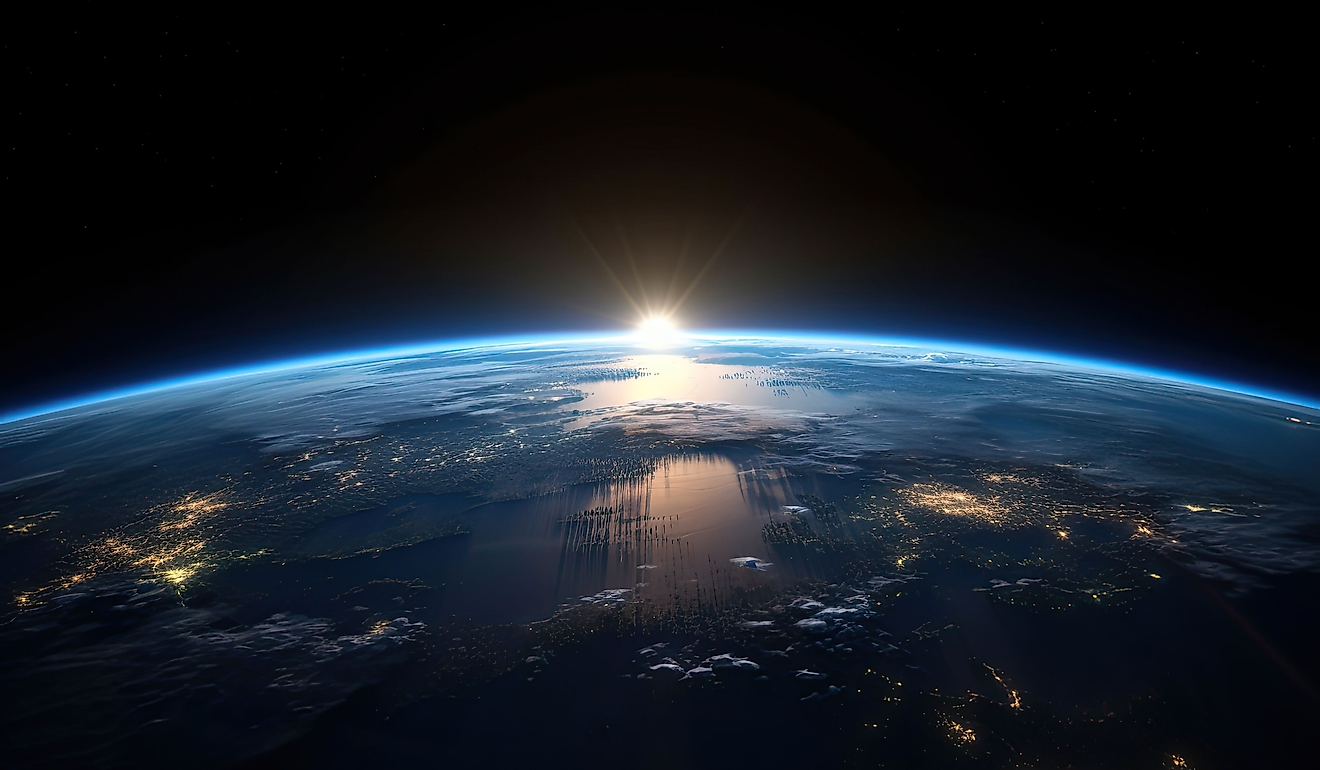
Where Does The Sun Rise And Set?
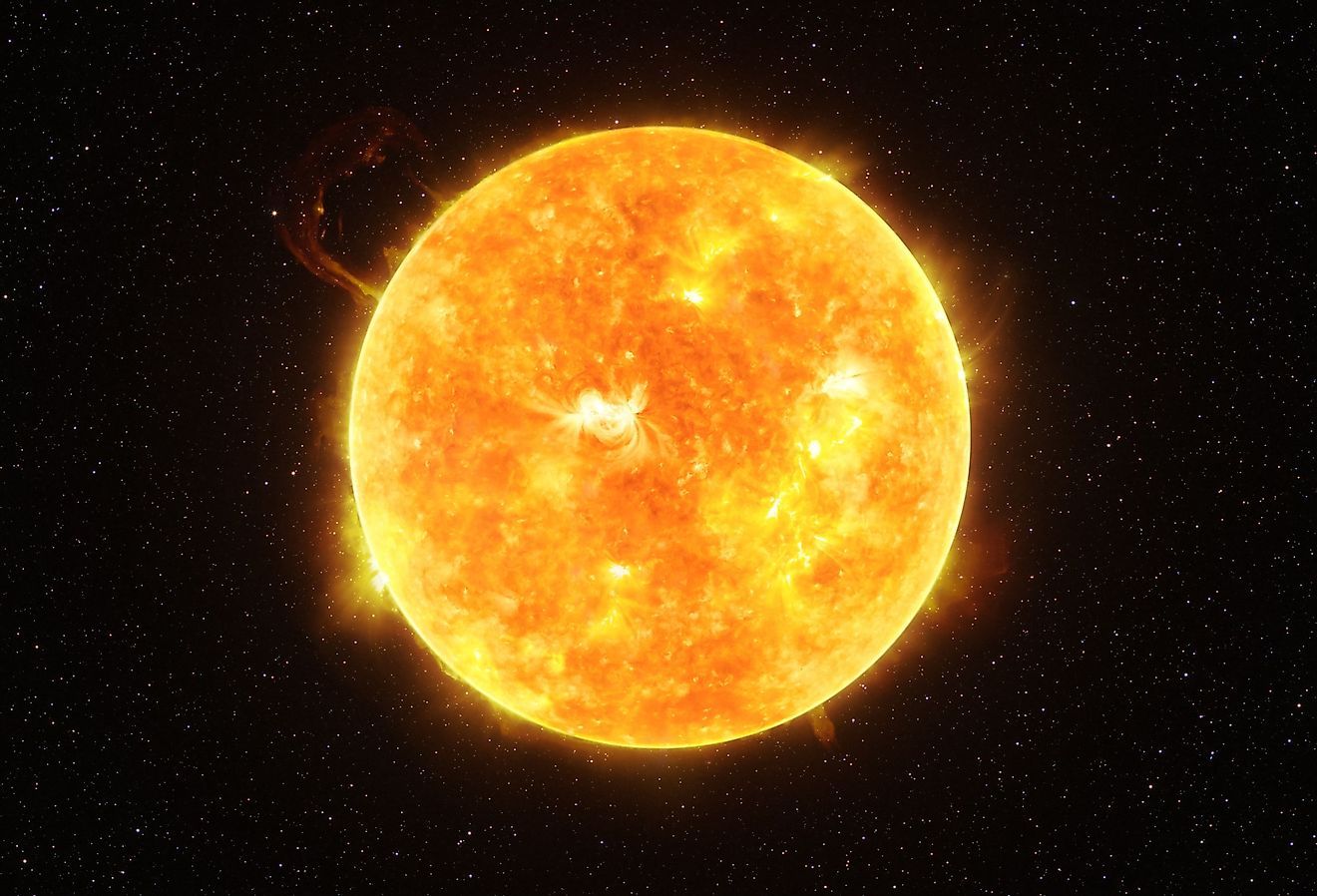
How Hot Is The Sun?
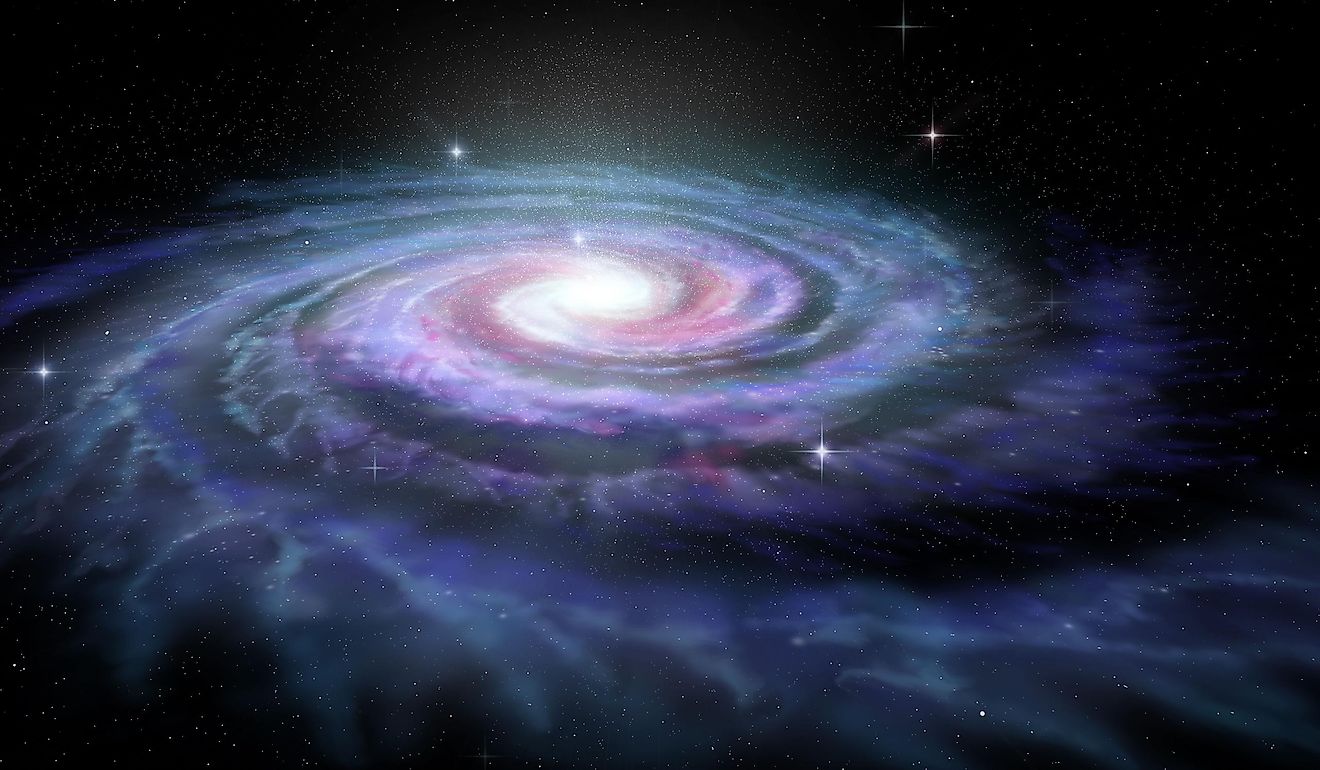
How Many Planets Are In The Milky Way?

In which medium sound travels fastest: air, water or steel?
Sound travels fastest through solids. this is because molecules in a solid medium are much closer together than those in a liquid or gas, allowing sound waves to travel more quickly through it. in fact, sound waves travel over 17 times faster through steel than through air..

Sound travels at the fastest speed in which medium?

If you're seeing this message, it means we're having trouble loading external resources on our website.
If you're behind a web filter, please make sure that the domains *.kastatic.org and *.kasandbox.org are unblocked.
To log in and use all the features of Khan Academy, please enable JavaScript in your browser.
High school physics - NGSS
Course: high school physics - ngss > unit 5, wave properties.
- Understand: mechanical waves
- Understand: electromagnetic waves
Want to join the conversation?
- Upvote Button navigates to signup page
- Downvote Button navigates to signup page
- Flag Button navigates to signup page

Video transcript
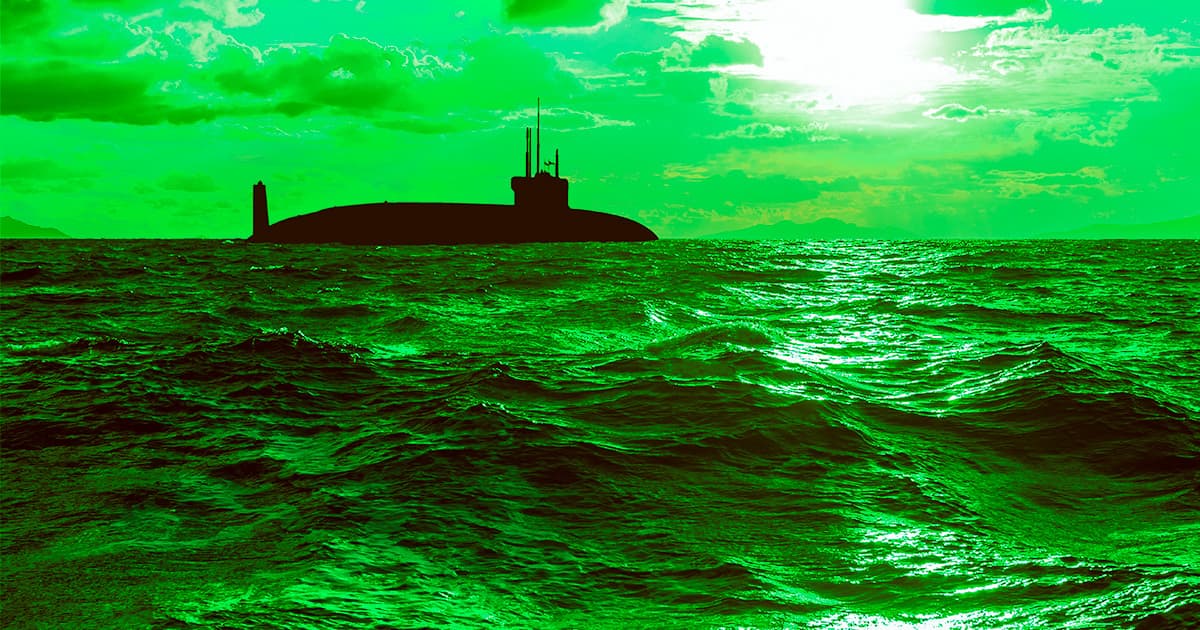
China Working on Super-Fast Submarines Powered by Lasers
We all lived in a laser submarine..
In China, scientists say they're developing technology that uses lasers to propel submarines nearly as fast as a jet engine.
As the South China Morning Post reports, engineers at the Harbin Engineering University in Heilongjiang province — where, notably, China's first experimental submarine was developed — claim that the country's military is close to achieving this colossal feat.
The idea behind the burgeoning technology is ingenious: lasers generate plasma underwater, which then creates a so-called "detonation wave" to propel a submarine vessel forward. As the SCMP notes, Japanese researchers first proposed this sort of laser propulsion methodology 20 years ago, and in China, scientists have been trying to figure out how to hack it for at least a decade .
Until now, attempts at making laser propulsion happen have been for naught, as scientists found it nearly impossible to generate a force that would push submarines in one specific direction.
But now, the Harbin researchers say they think they've cracked the code. Submarines using this technique would, as the engineers say in a recent paper in China's Acta Optica Sinica journal, be coated in thin optical fibers — each thinner than a strand of human hair, the SCMP notes — that would emit laser power.
The researchers claim that this method would be able to produce up to 70,000 newtons of thrust, which is a bit less than a commercial jet engine, using only two megawatts of laser power.
Aside from propelling a submarine forward, the directed laser energy would also result in what's known as "supercavitation," which occurs when bubbles coat the surface of an underwater projectile and increase its velocity. Theoretically, this could make a sub travel faster than the speed of sound — and hide it from sonar because, without mechanical power, it doesn't produce any mechanical noise vibrations, either.
News of this theoretical advancement comes after reports from last year indicating that the United States is concerned about losing the so-called submarine arms race to China, which has invested big in researching new underwater weapons technology.
While the concept of laser-propelled submarines sounds a bit "Star Wars"-esque, the weaponization — literally — of such tech is enough to give one pause.
"This method can also be applied to underwater weapons, causing a supercavitation phenomenon, thereby significantly increasing the underwater range of projectiles, underwater missiles, or torpedoes," project leader Ge Yang wrote in the paper, as quoted by the SCMP .
More on China: Report: Thanks to AI, China's Data Centers Will Drink More Water Than All of South Korea by 2030
Share This Article
Fast-food businesses hiking prices because of higher minimum wage sound like Gordon Gekko
'greed is right,' the 'wall street' character claimed. wrong. it's outrageous that multibillion dollar corporations begrudge workers a living wage and now take it out on their customers..
Conservative economists have been warning us about the dangers of raising the minimum wage for as long as I can remember, which is back to when I earned about $2.00 an hour, the federal minimum wage in 1975 .
So it should be no surprise that in California, which recently raised the minimum wage for large fast-food chains to at least $20 an hour, up from $16 an hour , those restaurants are raising prices – or threatening to – and planning to increase the use of kiosks to replace counter workers (though plans for such automation were likely already in place).
I remember when it was commonplace to hear arguments for abolishing the minimum wage. Let the free market sort out wages and prices, went the logic. Government intervention leads to inflation and unemployment. My college economics professor made the case passionately. If you don’t like your pay, get another job, get trained, go back to school, etc. The economy works best and grows fastest when everyone is out for themselves. Sure, people will fall through the cracks. Just don’t let it be you.
I was reminded of this when I watched the first "Wall Street" movie in which Gordon Gekko , played brilliantly by Michael Douglas and not accidentally named (though spelled slightly differently) after a reptile, gives the famous “greed is good” speech to the shareholders of a company he wishes to take over and pillage.
" Greed – for lack of a better word – is good ," Gekko says. "Greed is right. Greed works. Greed clarifies, cuts through, and captures the essence of the evolutionary spirit. Greed, in all of its forms – greed for life, for money, for love, knowledge – has marked the upward surge of mankind. And greed – you mark my words – will not only save Teldar Paper, but that other malfunctioning corporation called the USA.”
His reference to “the evolutionary spirit” seems an obvious nod, on the part of screenwriters Oliver Stone (also the film’s director) and Stanley Weiser , to the social Darwinism of the late 19th and early 20th centuries, a bogus theory used to justify racism and other inhumanity, a cinematic commentary on the shameless rapacity of corporate raiders during the 1980s.
How 'shareholder primacy' justifies greed
I’m afraid that “evolutionary spirit” is very much with us today: billionaires less interested in curbing climate change than preparing their personal bunkers to survive it; an airplane manufacturer that apparently has been putting profit ahead of safety; fire-arms manufacturers – and the politicians who carry their water – who seem to believe that any sale of a weapon is a good sale, even if it is to someone with mental illness or a grudge against a former spouse or co-worker, even if it results in mass slaughter; and all manner of other corporations maximizing profits regardless of the harm.
Such cravenness is often justified by the principle of “shareholder primacy,” the belief that corporations are bound by an obligation to maximize profits for the sake of their shareholders, and those of us who own stock or mutual funds or have a public pension are the financial beneficiaries of such blind loyalty to profit. But not all of us wish to be held morally hostage by our financial futures.
Pay your workers a living wage: 'Tipflation' is unfair to employees and customers
I believe there are many people, like me, with a modest share of the collective prosperity who are willing to enjoy a smaller financial return for the sake of those trying to subsist on the minimum wage in economically depressed communities. We find it outrageous that multibillion dollar corporations begrudge their workers a living wage and are willing to take it out on their own disadvantaged customers.
According to the National Conference of State Legislatures , 34 states, territories and districts have minimum wages above $7.25 an hour. Georgia and Wyoming have a minimum below that. Five states have not adopted a state minimum: Alabama, Louisiana, Mississippi, South Carolina and Tennessee. The federal minimum wage governs those seven states.
What's worth more to me than my hourly wage
That economics professor of mine used to say that capitalism was human nature, that competing for resources and consolidating wealth were as natural as the flowers in spring.
I thought he made sense then, but after 32 years teaching mostly underprivileged children, I am no longer so sure.
I am paid for my work and am not shy about demanding compensation – though, like many teachers , I often do more than I am required. I don’t mind it because what I get from helping students overcome their circumstances is worth more to me than my hourly wage.
What do you need to get into college? How 'objective' assessments fail my students.
This is something over which affluent friends have expressed envy. Perhaps some of that is patronizing, but I am quite sure that the endless pursuit of wealth really is a shallow endeavor doomed to leave most people feeling rather empty.
Many smart people know this and try to balance their lives – to pursue financial stability but not at the expense of their souls.
Wouldn’t we all be better off if the laws that govern business reflected that more?
Wouldn’t the future of humanity benefit from the most powerful corporations making a sincere commitment to the communities that make them so wealthy?
Larry Strauss, a high school English teacher in South Los Angeles since 1992, is the author of more than a dozen books, including “ Students First and Other Lies: Straight Talk From a Veteran Teacher ” and his new novel, " Light Man. "
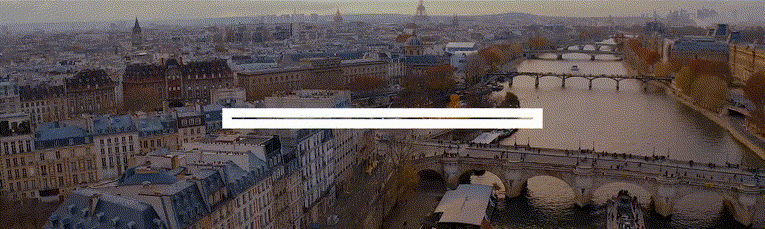
IMAGES
VIDEO
COMMENTS
For instance, if you heat up the air that a sound wave is travelling through, the density of the air decreases. This explains why sound travels faster through hotter air compared to colder air. The speed of sound at 20 degrees Celsius is about 343 meters per second, but the speed of sound at zero degrees Celsius is only about 331 meters per second.
They found that sound could travel close to the theoretical limit of 79,200 mph (127,460 km/h), confirming their initial calculations. In contrast, the speed of sound in air is roughly 767 mph ...
Table 17.2.1 17.2. 1 makes it apparent that the speed of sound varies greatly in different media. The speed of sound in a medium is determined by a combination of the medium's rigidity (or compressibility in gases) and its density. The more rigid (or less compressible) the medium, the faster the speed of sound.
The faster a sound wave travels, the more distance it will cover in the same period of time. If a sound wave were observed to travel a distance of 700 meters in 2 seconds, then the speed of the wave would be 350 m/s. A slower wave would cover less distance - perhaps 660 meters - in the same time period of 2 seconds and thus have a speed of 330 ...
However, the speed of sound varies from substance to substance: typically, sound travels most slowly in gases, faster in liquids, and fastest in solids. For example, while sound travels at 343 m/s in air, it travels at 1,481 m/s in water (almost 4.3 times as fast) and at 5,120 m/s in iron (almost 15 times as fast). In an exceptionally stiff ...
Table 17.3.1 17.3. 1 shows that the speed of sound varies greatly in different media. The speed of sound in a medium depends on how quickly vibrational energy can be transferred through the medium. For this reason, the derivation of the speed of sound in a medium depends on the medium and on the state of the medium.
As expected, the speed of sound on Mars is slower than on Earth. It travels at around 240 m/s or about 30% slower than on Earth. What scientists did not expect is that the speed of sound varies for different frequencies. A high pitched sound, like from the rover's laser, travels faster at around 250 m/s.
Both types of earthquake waves travel slower in less rigid material, such as sediments. P-waves have speeds of 4 to 7 km/s, and S-waves range in speed from 2 to 5 km/s, both being faster in more rigid material. The P-wave gets progressively farther ahead of the S-wave as they travel through Earth's crust.
In non-humid air at 20 degrees Celsius, the speed of sound is about 343 meters per second or 767 miles per hour. We can also watch the speed of sound of a repeating simple harmonic wave. The speed of the wave can again be determined by the speed of the compressed regions as they travel through the medium.
By Queen Mary University of London October 11, 2020. Scientists find the ultimate speed of sound: about 36 km per second (80,500 mph), twice as fast as sound in diamond, the hardest known material. A research collaboration between Queen Mary University of London, the University of Cambridge, and the Institute for High Pressure Physics in ...
So sound travels much faster in warm air near the ground than in colder air higher up, for example. And it travels roughly three times faster in helium gas than in ordinary air, because helium has much lighter molecules. That's why people who breathe in helium talk in funny voices: the sound waves their voices make travel faster—with higher ...
14.1. where v is the speed of sound (in units of m/s), f is its frequency (in units of hertz), and λ λ is its wavelength (in units of meters). Recall that wavelength is defined as the distance between adjacent identical parts of a wave. The wavelength of a sound, therefore, is the distance between adjacent identical parts of a sound wave.
This may seem fast, but it's tiny compared to light, which travels roughly a million times faster than sound (roughly 300,000,000 m/s). The medium. Sound requires some material in which to propagate (i.e. travel). This material sound travels through is called the medium. You can show that sound requires a medium by putting a cell phone inside ...
How fast do different types of waves travel? This chapter of the Physics LibreTexts book on sound explains the factors that affect the speed of waves, such as the medium, the frequency, and the tension. You will learn how to calculate the speed of waves using formulas and examples. You will also explore the differences between transverse and longitudinal waves, and how they affect the speed of ...
When it comes to the speed of sound, a solid object will allow the vibration to move much faster since it has the most densely packed molecules. It will also make the sound the loudest. After solids, liquids have the highest speed of sound. And then finally gas, that included our air since it is made up of gasses.
The speed of sound through air is about 343 m/s (or 760 mph), and it travels faster in warmer air than colder air. The speed of sound through water is about 1500 m/s, and it travels faster in salt water than fresh water. The speed of sound through solids is much faster than through either gases or liquids - about 5000-15000 m/s.
Sound travels faster through mediums with higher temperatures than at lower ones. Sound travels faster through hot air than cold air. Lyla was hammering nails into a wall so that she could hang pictures. Three of her friends each stood in nearby rooms that were about 40 meters away from the hammer and nail: Melissa, who stood in a cold room ...
The first notable difference between the speed of sound and light is how fast they are. In Earth's atmosphere, the speed of sound averages at about 761-miles per hour (1,225-kilometres per hour). That may seem fast, yet when compared to the speed of light, it seems quite small. Light travels at a staggering 670-million miles per hour (1.07 ...
Sound travels fastest through solids. This is because molecules in a solid medium are much closer together than those in a liquid or gas, allowing sound waves to travel more quickly through it. In fact, sound waves travel over 17 times faster through steel than through air. Suggest Corrections. 134.
It travels much faster than the sound associated with the lightning strike. Electromagnetic waves are special not only because they travel really fast, but they also don't need a medium to travel through. The thunder on the other hand is a sound wave traveling slower than the light. So you'll see the lightning before you hear the thunder.
Which of the following conditions will produce a sound wave? tuning fork when struck. A sound wave passes through three identical containers, each filled with one of three substances. In which one will sound travel fastest? container of solid iron. A sound wave traveling at 343 m/s takes 10.0 s to go from a speaker to a detector.
Study with Quizlet and memorize flashcards containing terms like Through which medium will sound travel most rapidly? A) vacuum B) air C) water D) steel, Sound waves in air A) are longitudinal waves. B) are due to compression and rarefaction. C) undergo refraction when they pass from warm to cold air. D) All of the choices are correct., The time that is required for a vibrating object to ...
Theoretically, this could make a sub travel faster than the speed of sound — and hide it from sonar because, without mechanical power, it doesn't produce any mechanical noise vibrations, either.
Fast-food businesses hiking prices because of higher minimum wage sound like Gordon Gekko 'Greed is right,' the 'Wall Street' character claimed.