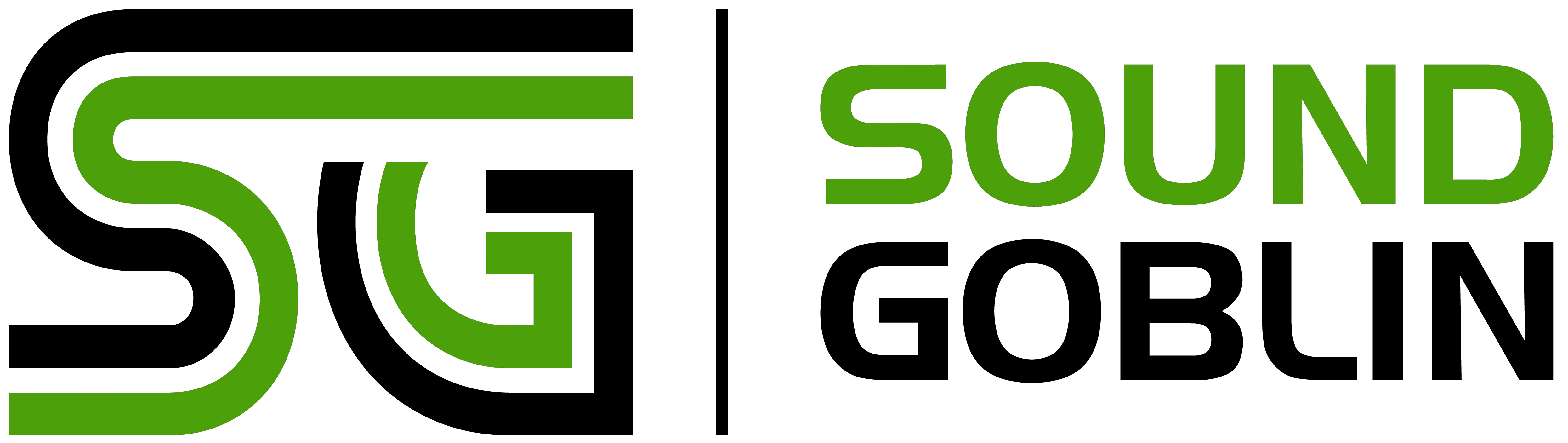
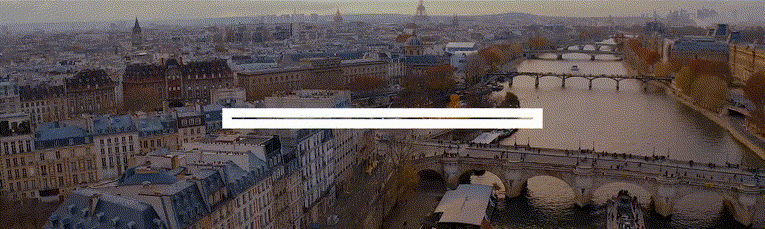
How Sound Travels Through Solids, Liquids and Gases
- August 13, 2022
We love to work with sound. Many of us record our own music, podcast, or other forms of sound. Knowing how sound travels through different mediums will allow you to have better control over the sound that you generate. That is what we will be looking at today. How does sound travel through solids, liquids, and gases?
Can the way that you produce sound and the medium that it moves in make a difference in the volume that you will hear? How does this change when it comes to the different mediums? Will the furniture in a room have any impact on the acoustics of the room? How can you change it to create the perfect recording environment?
These are just some of the things that we will discuss today. Knowing how sound travels through solids, liquids and gases are not only interesting, but it can have an impact on the way we record sounds and how we change things up.
Why Is the Way That Sound Travels Through Mediums Important?
One of the main reasons why it is important to understand how sound acts, is that when you understand something better, you can control it. As a youngster, I loved swimming. I still do. But one of my main attractions was that underneath the water it was the one place where everything went quiet. It always felt like the world stopped and it was just me and complete calm.
I loved my family but there was always so much going on that it was just a great place to just be with my own imagination and thoughts. I could make up imaginary worlds and people, and have millions of stories running through my mind. All because there was a lack of sound under the water.
But in reality, when you look at how sound behaves in a liquid, scientifically, this should not be the case. In fact, there should be more sound under the water than there is in the fresh air. Why? And why doesn’t it work that way? I wanted to find out.
For many of us who record sound, it is important to be connected to it. If you understand what makes something sound fuller, what makes a noise loud, and how things act, you can have better control and your recording will end up being closer to what you intended in the first place.
You might be recording a podcast, but for some reason, your voice keeps sounding muffled, understanding sound can help you identify what the cause could be and how you can fix it.
What Is Sound?
To understand why sound acts differently in different mediums we first have to understand what sound is.
First, you need to know that sound can not exist in a void. This is different from light that does travel through nothingness. That is why we can see light shining from space where there is a void and no atmosphere. But those that have been to space say it is completely quiet. It must be an almost eerie feeling.
Sound happens when something creates a vibration. This is done through musical instruments, our voices, speakers, and many other things. This then causes the medium around it, like the water or the air, to also vibrate and carry this sound with them. Without a medium, sound would not exist. That is because the molecules of the medium react and bump into those next to it and this allows the sound to travel on.
At the same time, the medium that is used will determine just how loud the sound will be, it will also determine how far can travel and how the sound will generally react. This is because different solutions will have molecules that are more or less densely packed.
Your surroundings will have a big impact. People who create a sound studio try to make the acoustics of the room as powerful as possible. This should help you do that.
Let us now look at the three mediums that sound can travel through, solids, liquids, and gases, and how they change the reaction of the sound.
First up we can look at gasses. You might wonder why gasses are mentioned when speaking of mediums that sound can move in. You may be visualizing a bunch of fog at a concert that makes the lights look incredible and makes the crowd go wild.
And that is one possibility of gasses that can be used as a medium for sound to travel through. But most of the time, our air is the only gas that sound needs to continue its vibration.
What Is the Air Made Of?
We have already mentioned that sound can not exist in a void. But we can hear each other when we speak out in the open. We can hear music when it is being played under the starry sky and we can even hear kids shouting in a park a block away .
That is because most of the air in our atmosphere is made up of gasses. Our atmosphere is not just a void, or we wouldn’t be able to live here anyway, but is made up of lots of gasses we can’t see. The atmosphere is made up of 78% Nitrogen, and 21% Oxygen, and the rest is a mixture of carbon dioxide, neon, and hydrogen.
This gives us all the ability to breathe without needing a space suit, but it also gives sound the ability to travel in our atmosphere. We make a vibration and the molecules of the gases that we can’t even see start to bump into each other and takes that vibration further, making it possible for us to hear sounds. It is pretty amazing when you think about it.
How Does Sound Travel Through Gases?
Gas is the medium that will have the slowest speed of sound of all of them. This is because the molecules of the gases surrounding us are expanded and far away from each other. The vibrations do get passed over to each other but it takes longer to do.
This is also why we often need things that can amplify our sounds like a microphone when we are speaking to a bigger group of people. These help us to make the vibrations bigger and to allow them to travel further than we would have been able to achieve with only our voice.
Some Things That Can Influence Sound in Gases
Have you ever felt that things are so much quieter after a big snowstorm? How the world seems almost different then? Turns out it might not just be your imagination. This is because the volume and speed of sound can be impacted by the temperature of the air and in turn the gas that is surrounding us.
At lower temperatures, the molecules move around quicker and they can vibrate quicker. The energy behind the sound can start to be lost and the sound will become quieter or be lost faster.
At normal room temperatures, the speed of sound will be a lot higher than it would be in the exact same room when the temperature is at freezing.
There are many different liquids that have a higher or lower density but for the most part, it is in water where we would be interested in hearing a sound. If we go swimming or put a small portable speaker close to the water, we would like to hear the sound as loud as possible. But it just doesn’t always work like that.
Let’s see how sound reacts in water or other liquids.
Sound In Water
The molecules in water are a lot more tightly packed than it is in gas. That is why sound travels much faster in water than it would travel in the air. Sound can actually travel in water almost four times faster than it can be in the air.
That is really impressive. And still, if you submerge your head underwater, you will hear the sound but it might sound muffled and not quite like the sound that you are used to.
Why Humans Hear Muffled Sounds in Water
The water molecules are more tightly packed and the energy that it uses to carry sound is transported faster. In theory, you should be able to hear noises a lot louder when you are underwater. But that is not how we perceive this sound.
This is because our ears are created to listen to sounds in the air. We pick up on sounds through our ear canal and these sounds are then transported to the brain that makes sense of it all. When you only submerge your ears, sounds will sound very muffled since the ears can’t take these sounds along the ear canal.
When you submerge your head fully suddenly the sound is clearer and louder. Although it could still be somewhat muffled compared to outside the water. Our heads contain a lot of water, and inside the water, it will be our tissue that picks up on the sound and detects it.
You could try to plug your ears but it will have very little effect on the volume of the noise under the water. The sound is not traveling along those normal lines.
At the same time, chances are that it is also very hard and almost impossible for you to figure out from which direction the sound is coming. When the sound travels along the normal route our brain has cues to determine if it comes from behind us or in front. But when the sound does not travel in those normal routes the brain has no way of telling us where it is coming from.
For humans communicating through sound under the water is not so easy. That is why divers have always used hand signals to communicate with their diving partners and why some have even started to use microphones that connect them. Allowing for a much better communication route.
We know that we can’t hear sound in the same way when we are inside water as when we are in the air. But what happens when we make a big sound inside the water, like shouting? Will someone that is on the outside be able to hear it clearly?
This is unlikely. That is because the surface of the water almost acts as a mirror for sound. Instead of the vibration moving outside of the water it gets reflected back. Making sure that very little sound is heard outside.
Animals In Water
Our ears might be designed to hear in air, but fish and mammals that live in the ocean can take advantage of the speed of sound inside the water. They are adapted to hearing noise completely clearly inside the water.
Since sound does travel quickly in water and they can hear it, they can use sounds to communicate over much larger distances than we are able to do with just our voice. Whales, for example, have been known to use their voice to communicate with other whales over massive distances in the ocean. The sound of a humpback whale can travel thousands of miles in the ocean. It also helps that the vibrations they can create are much larger than the ones our own vocal cords can produce.
Then finally there are solids and how sound reacts when they come into contact with a solid. Since sound starts to get muffled when there are a lot of solid objects in its path you would think that it travels a lot slower in solids. But surprisingly that is not the case. There are however reasons why it reacts in this way.
The Speed of Sound in Solids
A solid object is densely packed with its molecules. Each solid object will be a little bit different from the other depending on the material it is made of and how densely packed its molecules are. There are some materials that will work better as insolation to noise than others, but we will discuss the reason for this shortly. But for the most part, sound will travel a lot faster in solids than it will in both liquids and gasses.
This is because the source of the sound will create the vibration in the molecules of the sound and then these tightly packed molecules will quickly send the vibration further along. This means that the speed of sound is a lot faster when traveling in a solid object and that it will be a lot louder too.
Often a solid object will be a good source of amplification for a sound that you would like to enhance. The sound through a brass bugle gets enhanced through the design of the object and also through the material it is made of.
Examples Of Sounds in Solids
It can be hard to think of examples where solid objects are used to move sound and make it louder. Let’s discuss some simple examples of this.
You can put an ear to a solid object like a table and then make a soft tapping sound on the table. Compare how you heard it when your ear is on the solid compared to how loud it is when you hear the sound through the air. You will be surprised by how clearly the sound is enhanced by listening to it through a solid object.
Another great example of an experiment that many of us probably unknowingly did as children is a string telephone. You take two cups and a long line of string. The two cups are each connected to one side of the string, one person listens into one cup while another speaks into the cup at their end.
In this experiment, the vibrations are created and enhanced by the shape of the cup. Then these vibrations are transferred with the help of a solid object, the string, and the other person can hear your message at the other end of the string. Without raising your voice or shouting.
It is always amazing to see just how far this simple design can carry sound. Fun fact, the world record for the longest-ever string telephone, which was made with tin cans, was a whopping 796 feet long. That is almost the distance of three football fields. That is a long way for a piece of string and two cups to carry sound.
Then another great example of a sound being a lot louder when it is carried through a solid object is sounds that you can hear in the air. For example, hearing the sound of a horse coming closer, its hooves beating down on the ground.
It is already a pretty impressive sound when you hear it in normal circumstances. But try putting your head to the ground and listening to the approach in that way. The sound is much louder and you can almost feel the vibrations that are making the sound you hear.
Why Does Sound Get Muffled Through a Door?
We know now that sound travels much faster through solid objects than it does through gasses or liquids. You would think that a solid object like a wall or a door will enhance the sound but the opposite is true. A sound that is coming from a different room is more muffled.
If there is a lot of noise outside your home, for example, the neighbors having a party, it works to close the doors and windows and the sound won’t bother you as much. Even if you only have standard windows and doors.
How does that work? It works because the sound you are hearing does not originate from inside a solid object. It traveled through the air until it came to your door. There it encountered a solid object. And instead of making the vibrations louder this change in medium made the sound lose some of the energy that it was traveling with. This reduces the level of the noise and makes it less noticeable when there are doors that are closed.
Why Rooms Echo
This change in energy is also one of the reasons why a room will or won’t echo. When you go into an empty room there is a good chance that you can create an echo. That is because the empty room has no solid objects that break the energy of the noise down and stop it.
The vibrations bump only against the walls and reflect back. If you have a room that is still echoing even after your furniture has been installed, then there might not be enough solid objects that stop the speed and the energy of the sound. Something like a carpet that can absorb the vibration can help to stop the echo in the room.
How Sound Travels Through Solids, Liquids, and Gases
Sound needs a medium that can take the vibrations and move them along, allowing us to hear the sounds that are being created.
When it comes to the speed of sound, a solid object will allow the vibration to move much faster since it has the most densely packed molecules. It will also make the sound the loudest. After solids, liquids have the highest speed of sound. And then finally gas, that included our air since it is made up of gasses.
When a sound is traveling through one medium like air and then encounters another, like a solid door, it loses some of its energy and some of the volume will be lost. That is why solid insulation against sound is still one of the best options despite solids being a good conductor of sound.
We might not be able to take full advantage of the high speed of sound that can be found inside a liquid, but those living in the ocean sure can and that is why whale sounds can travel thousands of miles under the water.
Knowing how sound reacts to different mediums will allow us to understand it better. And that means that you should have better control over your recordings and all the ways that you like to create your own very unique sounds.
Leave a Reply Cancel Reply
Add Comment
Save my name, email, and website in this browser for the next time I comment.
Post Comment
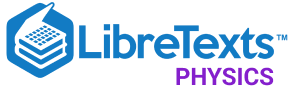
- school Campus Bookshelves
- menu_book Bookshelves
- perm_media Learning Objects
- login Login
- how_to_reg Request Instructor Account
- hub Instructor Commons
- Download Page (PDF)
- Download Full Book (PDF)
- Periodic Table
- Physics Constants
- Scientific Calculator
- Reference & Cite
- Tools expand_more
- Readability
selected template will load here
This action is not available.
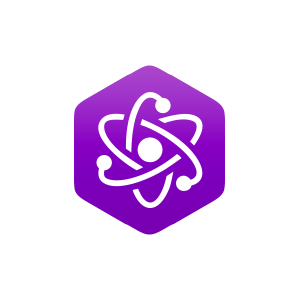
- You do not have permission to view this page - please try signing in.
- Password reset
Welcome to the LibreTexts library. For other users, please log in with LibreOne.
- Random article
- Teaching guide
- Privacy & cookies
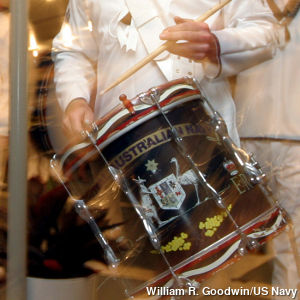
by Chris Woodford . Last updated: July 23, 2023.
Photo: Sound is energy we hear made by things that vibrate. Photo by William R. Goodwin courtesy of US Navy and Wikimedia Commons .
What is sound?
Photo: Sensing with sound: Light doesn't travel well through ocean water: over half the light falling on the sea surface is absorbed within the first meter of water; 100m down and only 1 percent of the surface light remains. That's largely why mighty creatures of the deep rely on sound for communication and navigation. Whales, famously, "talk" to one another across entire ocean basins, while dolphins use sound, like bats, for echolocation. Photo by Bill Thompson courtesy of US Fish and Wildlife Service .
Robert Boyle's classic experiment
Artwork: Robert Boyle's famous experiment with an alarm clock.
How sound travels
Artwork: Sound waves and ocean waves compared. Top: Sound waves are longitudinal waves: the air moves back and forth along the same line as the wave travels, making alternate patterns of compressions and rarefactions. Bottom: Ocean waves are transverse waves: the water moves back and forth at right angles to the line in which the wave travels.
The science of sound waves
Picture: Reflected sound is extremely useful for "seeing" underwater where light doesn't really travel—that's the basic idea behind sonar. Here's a side-scan sonar (reflected sound) image of a World War II boat wrecked on the seabed. Photo courtesy of U.S. National Oceanographic and Atmospheric Administration, US Navy, and Wikimedia Commons .
Whispering galleries and amphitheaters
Photos by Carol M. Highsmith: 1) The Capitol in Washington, DC has a whispering gallery inside its dome. Photo credit: The George F. Landegger Collection of District of Columbia Photographs in Carol M. Highsmith's America, Library of Congress , Prints and Photographs Division. 2) It's easy to hear people talking in the curved memorial amphitheater building at Arlington National Cemetery, Arlington, Virginia. Photo credit: Photographs in the Carol M. Highsmith Archive, Library of Congress , Prints and Photographs Division.
Measuring waves
Understanding amplitude and frequency, why instruments sound different, the speed of sound.
Photo: Breaking through the sound barrier creates a sonic boom. The mist you can see, which is called a condensation cloud, isn't necessarily caused by an aircraft flying supersonic: it can occur at lower speeds too. It happens because moist air condenses due to the shock waves created by the plane. You might expect the plane to compress the air as it slices through. But the shock waves it generates alternately expand and contract the air, producing both compressions and rarefactions. The rarefactions cause very low pressure and it's these that make moisture in the air condense, producing the cloud you see here. Photo by John Gay courtesy of US Navy and Wikimedia Commons .
Why does sound go faster in some things than in others?
Chart: Generally, sound travels faster in solids (right) than in liquids (middle) or gases (left)... but there are exceptions!
How to measure the speed of sound
Sound in practice, if you liked this article..., find out more, on this website.
- Electric guitars
- Speech synthesis
- Synthesizers
On other sites
- Explore Sound : A comprehensive educational site from the Acoustical Society of America, with activities for students of all ages.
- Sound Waves : A great collection of interactive science lessons from the University of Salford, which explains what sound waves are and the different ways in which they behave.
Educational books for younger readers
- Sound (Science in a Flash) by Georgia Amson-Bradshaw. Franklin Watts/Hachette, 2020. Simple facts, experiments, and quizzes fill this book; the visually exciting design will appeal to reluctant readers. Also for ages 7–9.
- Sound by Angela Royston. Raintree, 2017. A basic introduction to sound and musical sounds, including simple activities. Ages 7–9.
- Experimenting with Sound Science Projects by Robert Gardner. Enslow Publishers, 2013. A comprehensive 120-page introduction, running through the science of sound in some detail, with plenty of hands-on projects and activities (including welcome coverage of how to run controlled experiments using the scientific method). Ages 9–12.
- Cool Science: Experiments with Sound and Hearing by Chris Woodford. Gareth Stevens Inc, 2010. One of my own books, this is a short introduction to sound through practical activities, for ages 9–12.
- Adventures in Sound with Max Axiom, Super Scientist by Emily Sohn. Capstone, 2007. The original, graphic novel (comic book) format should appeal to reluctant readers. Ages 8–10.
Popular science
- The Sound Book: The Science of the Sonic Wonders of the World by Trevor Cox. W. W. Norton, 2014. An entertaining tour through everyday sound science.
Academic books
- Master Handbook of Acoustics by F. Alton Everest and Ken Pohlmann. McGraw-Hill Education, 2015. A comprehensive reference for undergraduates and sound-design professionals.
- The Science of Sound by Thomas D. Rossing, Paul A. Wheeler, and F. Richard Moore. Pearson, 2013. One of the most popular general undergraduate texts.
Text copyright © Chris Woodford 2009, 2021. All rights reserved. Full copyright notice and terms of use .
Rate this page
Tell your friends, cite this page, more to explore on our website....
- Get the book
- Send feedback
Speed of Sound in Solids
Claudia Tiller, Spring 2023
This page discusses the speed of sound in various solids, how to calculate them, and examples of such calculations.
- 1 The Main Idea
- 2.1 A Mathematical Model
- 2.2 Speeds of Various Compositions
- 3.1 Theoretical
- 3.2 Numerical Example
- 4 Connectedness
- 6.1 Further reading
- 6.2 External links
- 7 References
The Main Idea
The speed of sound can be defines as the distance travelled per a unit of time by a sound wave as it travels through an elastic medium. Elasticity refers to the ability of a body to resist distorting influence and return to its original shape when that influence is removed.
Factors that control the speed that sound travels in solids is measured by the solid's density and elasticity, as they affect the vibrational energy of the sound. Overall, the way the solid is composed determines the sound's speed limit through that solid.
The Structure of Solids and Effects on Sound Travel
Mediums are composed of particles that can be closely knit together or spread apart. Solids are characterized by an arrangement of atoms, ions, or molecules, where these components are generally locked in their positions. The particles can also be defined as elastic or inelastic. Particles that are closer to each other allow sound to be transferred quicker through the medium. Since particles that are compressed closer together allow sound to travel faster, it can be reasoned that sound travels slower in air.
With an increase in density, the space between particles in the solid decreases. The smaller distance between particles, or interatomic distance, the higher the speed. With an increase in elasticity of the atoms that make up the object, the lower the speed of sound in the object. Particles that have a high elasticity take more time to return to their place once they received vibrational energy. However, if the solid is completely inelastic then the sound cannot travel through it.
The practicality of this concept is highly applicable. The human ear captures sound waves through the outer cartilage of the ear, called the Pinna. The sound waves then travel up the ear canal and arrive at the ear drum which vibrates from the sound waves. After traveling through the inner ear, the vibrations arrive at the Cochlea. The Cochlea transfers the vibrations into information that auditory nerves can analyze.
A Mathematical Model
The speed of sound in solids [math]\displaystyle{ {V_{s}} }[/math] can be determined by the equation. Young's Modulus is a measure of elasticity of an object, and it can be computed to solve for interatomic values, such as interatomic bond stiffness or interatomic bond length.
[math]\displaystyle{ {V_{s}} = d \cdot \sqrt{\frac{K_{s}}{m_{atom}}} }[/math]
Alternative speed equation:
[math]\displaystyle{ {V_{s}} = \sqrt{\frac{B}{\rho}} }[/math]
[math]\displaystyle{ ρ }[/math] = density
[math]\displaystyle{ B }[/math] = Bulks Modulus
Bulks Modulus = [math]\displaystyle{ {\frac {ΔP}{ΔV/V}} }[/math]
[math]\displaystyle{ d }[/math] = interatomic bond length
[math]\displaystyle{ K_{s} }[/math] = Interatomic bond stiffness
Youngs Modulus = ( [math]\displaystyle{ Y = K_{s}/d }[/math] )
Youngs Modulus: [math]\displaystyle{ Y ={\frac{Stress}{Strain}} }[/math]
[math]\displaystyle{ Stress = {\frac{F_{tension}}{Area_{Cross Sectional}}} }[/math]
[math]\displaystyle{ Strain = {\frac{ΔL_{wire}}{L_{0}}} }[/math]
Speeds of Various Compositions
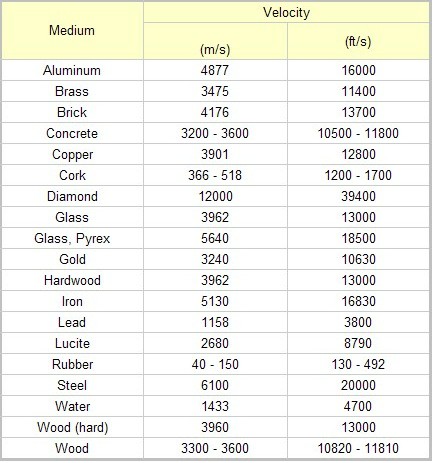
Theoretical
Two metal rods are made of different elements. The interatomic spring stiffness of element A is four times larger than the interatomic spring stiffness for element B. The mass of an atom of element A is four times greater than the mass of an atom of element B. The atomic diameters are approximately the same for A and B. What is the ratio of the speed of sound in rod A to the speed of sound in rod B?
Solution: In this situation, the ratio of the speed of sound in rod A to the speed of sound in rod B is 1.
Looking at the formula for computing speed of sound in solids, [math]\displaystyle{ {V_{s}} = d \cdot \sqrt{\frac{K_{s}}{m_{atom}}} }[/math] , you see that velocity depends three factors, interatomic stiffness, the mass of one atom, and interatomic bond length. The two rods differences in atomic mass and interatomic stiffness offset each other when the equations are set equal, and the ratio is determined to be 1.
[math]\displaystyle{ d \cdot \sqrt{\frac{4 \cdot K_{s}}{m_{atom}}} = d \cdot \sqrt{\frac{4 \cdot K_{s}}{m_{atom}}} }[/math] After simplification [math]\displaystyle{ V_{s_{1}} = V_{s{2}} }[/math]
Numerical Example
The Young's Modulus value of silver is 7.75e+10, atomic mass of silver is 108 g/mole, and the density of silver is 10.5 g/cm3. Using this information, calculate the speed of sound in silver.
Solution: The key to solving this problem is to realize the micro-macro connection of Young's Modulus. You are given that Young's Modulus is equal to 7.75e+10, and we know that Youngs Modulus = ( [math]\displaystyle{ K_{s}/d }[/math] ). In this situation, we need to calculate the interatomic bond length and use it and our Young's Modulus value to determine our interatomic stiffness.
To solve for d , we use the given density of silver (10.5 g/cm3). Using the basic equation for volume in relation to density and mass ( [math]\displaystyle{ V=m*d }[/math] ), we can find d , since d is equal to the cube root of volume.
Once d is solved for, it can be plugged back into the the equation [math]\displaystyle{ Y = K_{s}/d }[/math] to solve for [math]\displaystyle{ K_{s} }[/math]
Now, we have solved for both interatomic bond length and stiffness. The only quantity in the final speed of sound equation we need is the mass of one atom, which can be determined using Avogardro's number and the atomic mass. [math]\displaystyle{ m_{atom} = }[/math] atomic mass / [math]\displaystyle{ 6.022e23 }[/math]
Now that all variables are solved for, we can substitute values into our [math]\displaystyle{ {V_{s}} = d \cdot \sqrt{\frac{K_{s}}{m_{atom}}} }[/math] equation.
[math]\displaystyle{ {V_{s}} = 1.6 \cdot 10^{-10} \cdot \sqrt{ \frac{78534.7}{1.79 \cdot 10^{-22}}} }[/math]
[math]\displaystyle{ {V_{s}} = 2723 }[/math] m/s
Connectedness
Computing the speed of sound in solids depends on a mass' interatomic properties, such as interatomic bond length. In this specific case, an object's elasticity depends on the interatomic bond length. There are many applications that connect to the ability to compute the speed of sounds.
Seismic and ultrasonic imaging are also fields that benefit greatly from the calculation of sound speeds in solids. Seismic imaging refers to capturing images of the subsurface structure of the Earth. Seismic waves can be generated by earthquakes or other sources. Engineers and scientists can then locate gas and oil reservoirs, monitor activity such as volcano eruptions, and geological formations. In ultrasonic imaging, ultrasonic waves can be sent through the body and have the time it takes to bounce back be measured. This then creates images of internal organs or tissues, used in cases such as prenatal imaging as well as medical diagnosing. In more specific fields such as in Industrial Engineering, these calculations could be applied to questions regarding how to build a soundproof area. It would therefore be optimal to select a solid with a low speed of sound velocity, with a solid that has tightly packed particles. There are many vast applications to this, as an object's ability to block or allow sound waves through it. However, some cases require contractors to build structures that allow sound to travel through. Material testing uses the calculation of sound in solids to determine mechanical properties of such materials. Engineers can then calculate the material's elasticity, stiffness, and other properties. Knowledge regarding how solids are structured and how they correlate with the speed of sounds in those solids are vital to building structures that meet the criteria.
Overall, being able to calculate the speed of sounds in solids has a wide range of applications in engineering, medicine, and science.
The speed of sound in air was first measured by Sir Isaac Newton, and first correctly computed by Pierre-Simon Laplace in 1816. Before this precise measurement, attempts had been made across Europe during the 1700s, most famously Reverend William Derham's experiment in 1709 across the town of Upminister, England. Reverend Derham used a shotgun's noise and several known landmarks around time to measure the time it took for the sound of the blast to be heard from select distances.
Young's Modulus was named after English physicist Thomas Young. In actuality, the concept was developed earlier by physicists Leonhard Euler and Giordano Riccati in the 1720s.
Youngs Modulus: [1] Interatomic Bonds: [2]
Further reading
Further Information can be found on the speed of sound in solids in Matter and Interactions, 4th Edition by Ruth W. Chabay & Bruce A. Sherwood
External links
Internet resources on this topic can be found at:
Engineering Tool Box [3]
Hyperphysics [4]
Potto Project [5]
NDT Resource Center [6]
The Engineering ToolBox [7]
Ear Image [8]
Yew Chung [9]
This section contains the the references used while writing this page
Chart from [10]
Matter and Interactions 4th Edition by Chabay and Sherwood
Wikipage created by Daiven Patel
Navigation menu
FREE K-12 standards-aligned STEM
curriculum for educators everywhere!
Find more at TeachEngineering.org .
- TeachEngineering
- Traveling Sound
Hands-on Activity Traveling Sound
Grade Level: 4 (3-5)
Time Required: 30 minutes
Expendable Cost/Group: US $2.00
Group Size: 2
Activity Dependency: None
Subject Areas: Physical Science, Reasoning and Proof, Science and Technology
NGSS Performance Expectations:
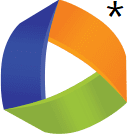
Curriculum in this Unit Units serve as guides to a particular content or subject area. Nested under units are lessons (in purple) and hands-on activities (in blue). Note that not all lessons and activities will exist under a unit, and instead may exist as "standalone" curriculum.
- Seeing and Feeling Sound Vibrations
- Pitch and Frequency
- Sound Visualization Stations
TE Newsletter
Engineering connection, learning objectives, materials list, worksheets and attachments, more curriculum like this, pre-req knowledge, introduction/motivation, vocabulary/definitions, troubleshooting tips, activity extensions, activity scaling, user comments & tips.
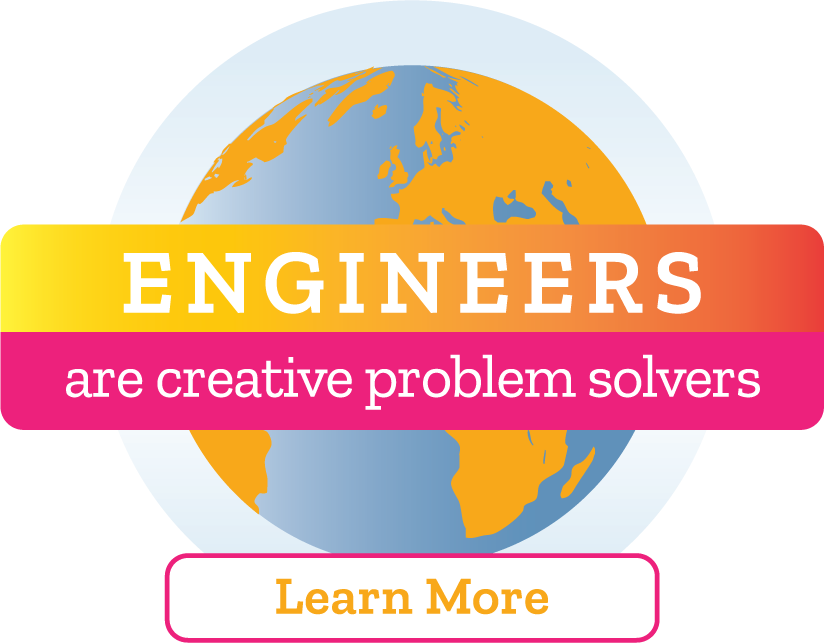
Sound and acoustic engineers know that the shape of a room and its materials greatly impact how sound waves travel. Recording studios are designed in soundproof booths so that the recorded music does not contain any unwanted external noise. Libraries are designed to reduce any introduced noises, to assure a quiet, non-distracting learning environment. Concert halls are designed so that sound generated on the stage travels to the back of the space without being distorted.
After this activity, students should be able to:
- Explain that sound can move through solids, liquids and gases.
- Describe how sound needs molecules to move and that changing the medium that it travels through changes the sound.
- Describe how engineers use sound energy when designing spaces, such as movie theaters.
Educational Standards Each TeachEngineering lesson or activity is correlated to one or more K-12 science, technology, engineering or math (STEM) educational standards. All 100,000+ K-12 STEM standards covered in TeachEngineering are collected, maintained and packaged by the Achievement Standards Network (ASN) , a project of D2L (www.achievementstandards.org). In the ASN, standards are hierarchically structured: first by source; e.g. , by state; within source by type; e.g. , science or mathematics; within type by subtype, then by grade, etc .
Ngss: next generation science standards - science, international technology and engineering educators association - technology.
View aligned curriculum
Do you agree with this alignment? Thanks for your feedback!
State Standards
Colorado - science.
Each group needs:
- large bowl (metal works best)
- 2 metal objects, such as spoons, to knock together
- Traveling Sound Worksheet , one per student
A basic understanding of the phases of matter: liquids, solids and gases.
Sound engineers are especially interested in the way sound travels. Can you hear as well when you sit in the back of the class as when you sit in the front? What about in the assembly hall or gymnasium? On the playground? Can you think of other times when you cannot hear as well as someone else? What happened? How about in a movie theater? What do engineers do so that the sound quality is good for everyone in a movie theater? (Possible answers: Add speakers around the room, curtains, carpet the walls, cone-shaped theaters act like a megaphone and help to direct sound waves further.)
Which is louder—walking on carpet or on tile? It is quieter on carpet because the carpet absorbs the sound energy . Sound energy, light energy and other types of energy, need molecules to travel through and vibrate , but sometimes sound energy is absorbed by an object or material. Engineers use this idea when designing rooms that are meant to be quiet. Have you ever noticed how the walls of a movie theater are covered with carpet or fabric? This is to prevent echoing of the sound system. Sometimes when you are in an empty room, your voice echoes or sounds hollow. This is because an empty room has no materials in it that might absorb the sound energy, so the sound bounces off the hard walls, back at you. This makes it hard to hear clearly.
Do you think sound energy can travel through air? Of course it can! That is how sound energy travels when you talk to a friend. How about water? Can you hear sound travel under water? How about a solid? Can sound move through a solid object? Engineers want to know if sound can travel through solids, liquids and gases so they can develop ways to send messages to people all over the world. Can you imagine how great sound would be if it could travel anywhere?
Understanding the properties of sound and how sound waves travel helps engineers determine the best room shape and construction materials when designing libraries, classrooms, sound recording studios, concert halls and theatres. Room shape and materials can impact how sound waves travel since sound waves bounce off different object in different ways. In this activity, we are going to study how sound waves travel through liquids, solids and gases, and think about how engineers might use this information.
Before the Activity
- Gather materials and make copies of the Traveling Sound Worksheet .
- Divide the class into teams of two students each.
With the Students
- Ask the students to predict if sound can move through solids, liquids and gases.
- Have the students complete the worksheet, which leads them through traveling sound wave activities.
- Can sound energy travel through solids? Students place their ears on a desk or table as they tap or scratch on the top. They compare that to the same sound made when their ear is not pressed to the table.
- Can sound energy traveling through liquids? Fill a large bowl or bucket (metal works best) with water. One student taps two spoons together under the water. Two other students observe and compare the tapping sound they hear, as heard through the air and as heard by placing an ear against the bowl.
- Can sound energy traveling through gases (air)? The students feel their throats gently during each of these tasks:
- Hum with your mouth and nose open.
- Hum with your mouth open and nose closed.
- Hum with your mouth closed and nose open.
- Hum with your mouth and nose closed.
- Discuss with the students what happened. Were their predictions correct? Can sound travel through air, water and solids? (Answer: Yes!) Sound needs molecules to move. Solids, liquids and gases are all made of molecules. The characteristics of the molecules (for example, the space between the molecules) determine whether the sound becomes muffled or changes in some way.
- How might engineers use the knowledge that sound travels through solids, liquids and gases? (Possible answers: Engineers create devices that send sound anywhere — through water to a submarine in the ocean, through wires to your TV, and through the air in surround sound movie theaters or emergency broadcast signals.)
echo: Repetition of a sound by reflection of sound waves from a surface.
frequency: The rate of vibrations in different pitches.
pitch: The highness or lowness of a sound.
sound energy: Audible energy that is released when you talk, play musical instruments or slam a door.
sound wave: A longitudinal pressure wave of audible or inaudible sound.
vibration: When something moves back and forth, it is said to vibrate. Sound is made by vibrations that are usually too fast to see.
volume: When sound becomes louder or softer.
wave: A disturbance that travels through a medium, such as air or water.
Pre-Activity Assessment
Prediction: Ask students if they think sound can move through solid, liquid, and gas. If so what are some examples? (Possible examples: Students may recall talking under water or using tin can and string telephones.)
Activity Embedded Assessment
Worksheet: Have students use the Traveling Sounds Worksheet to guide them in the activity and as a place to record their observations. Review their answers to gauge their mastery of the subject.
Post-Activity Assessment
Toss-a-Question: Ask students to independently think of an answer to the question below and write it on a half sheet of paper. Have students wad up and toss the paper to another team member who then adds their answer idea. After all students have written down ideas, have them toss the paper wad to another team, who reads the answers aloud to the class. Discuss answers with the class.
- What is an example of something through which sound can travel?
Neighbor Check: Have the students compare their activity observations with a neighbor. Are they the same or different? Have each team report some of their similar and dissimilar observations to the rest of the class.
Engineering Design: The supply of air on Earth is running out! Several futuristic cities for human habitation are being designed either underwater or deep inside mountains. Have each student group become a city planning engineering team and draw a communication system for sending emergency messages between the new cities. Make sure to illustrate and describe how the sound energy (message) will move through air, water or solid rock.
This activity can be very loud. Ask students to not disturb others while they learn and have fun.
To bring some humor to the activity, ask each student to hum a small part of their favorite song while feeling their throat. Have each student alternate between having their nose and mouth open or closed while humming non-stop. Why does the sound change depending on whether you close your nose or mouth? What happens if you block your ears? What does this activity teach us about sound? (Answer: Sound vibrations must travel through air for us to hear them. Like a musical instrument [perhaps a recorder or flute], if you change the holes where sound escapes, it changes the pitch, but not the frequency/vibrations of the sound.)
If a metal bowl is used during the activity, the vibrations from the objects colliding underwater vibrate the bowl, creating the illusion that the bowl is being struck. Have students draw the vibrations in the bowl on a piece of paper. Do the vibrations change if the objects are tapped together increasing softly?
Have students think about different forms of communications. Does sound travel most often through solids, liquids or gases? Have students poll their friends, family and neighbors to solicit their ideas.
For lower grades, conduct the activities as a class instead of in teams. Younger students could also draw pictures of their observations instead of writing in sentence form.

Students are introduced to the sound environment as an important aspect of a room or building. Several examples of acoustical engineering design for varied environments are presented.

Students learn how different materials reflect and absorb sound.

Students learn that sound is energy and has the ability to do work. Students discover that sound is produced by a vibration and they observe soundwaves and how they travel through mediums. They understand that sound can be absorbed, reflected or transmitted.
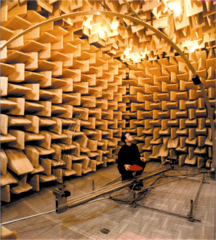
Students use the engineering design process to design and create soundproof rooms that use only one type of material. They learn and explore about how these different materials react to sound by absorbing or reflecting sound and then test their theories using a box as a proxy for a soundproof room. ...
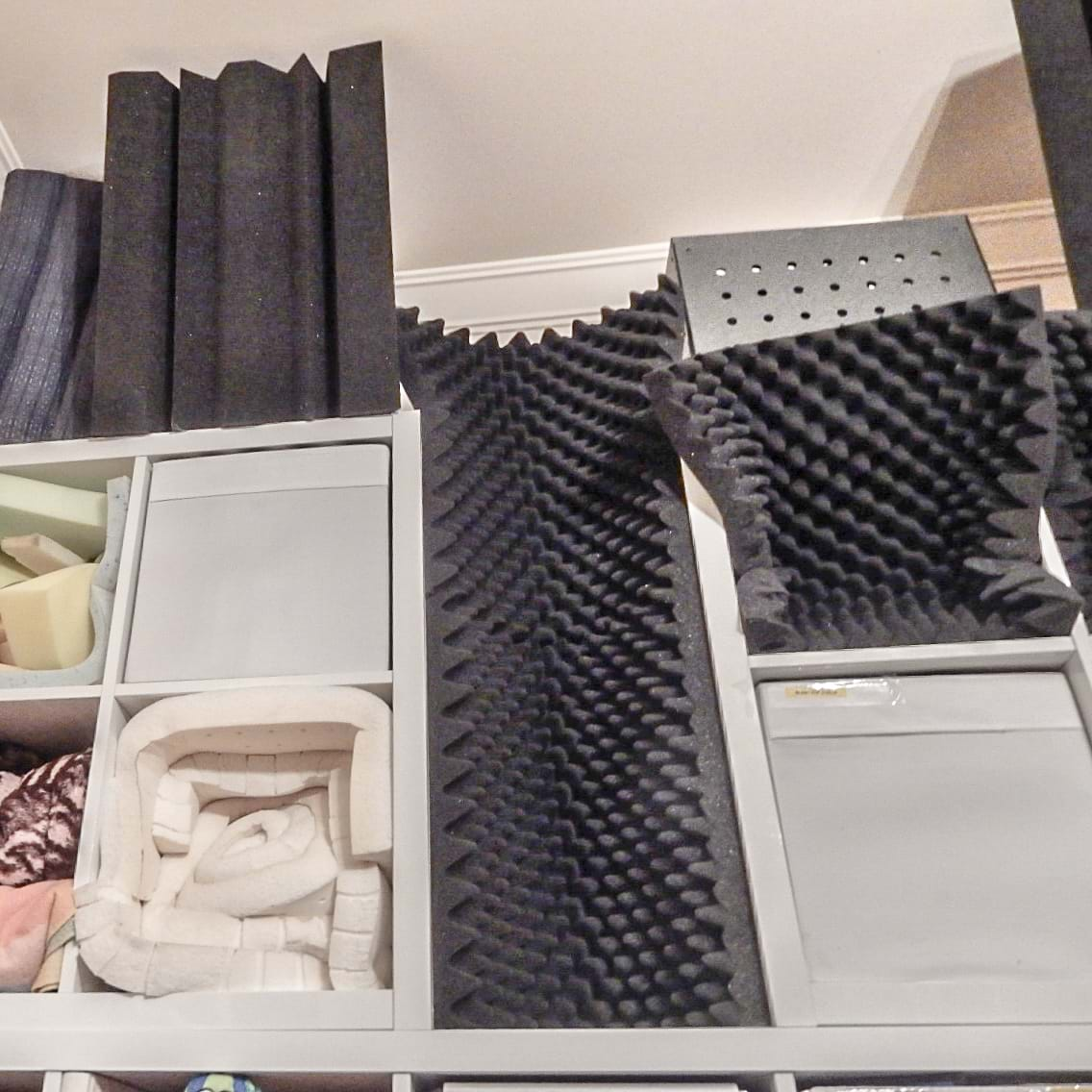
Dictionary.com. Lexico Publishing Group, LLC. Accessed December 19, 2005. (Source of some vocabulary definitions, with some adaptation.) http://www.dictionary.com
Contributors
Supporting program, acknowledgements.
The contents of this digital library curriculum were developed under grants from the Fund for the Improvement of Postsecondary Education (FIPSE), U.S. Department of Education and National Science Foundation (GK-12 grant no. 0338326). However, these contents do not necessarily represent the policies of the Department of Education or National Science Foundation, and you should not assume endorsement by the federal government.
Last modified: March 17, 2021
Want to create or adapt books like this? Learn more about how Pressbooks supports open publishing practices.
Traveling waves
17 How sound moves
Speed of sound.
There’s a delay between when a sound is created and when it is heard. In everyday life, the delay is usually too short to notice. However, the delay can be noticeable if the distance between source and detector is large enough. You see lightning before you hear the thunder. If you’ve sat in the outfield seats in a baseball stadium, you’ve experienced the delay between seeing the player hit the ball and the sound of the “whack.” Life experiences tell us that sound travels fast, but not nearly as fast as light does. Careful experiments confirm this idea.
The speed of sound in air is roughly 340 m/s. The actual value depends somewhat on the temperature and humidity. In everyday terms, sound travels about the length of three and a half foot ball fields every second- about 50% faster than a Boeing 747 (roughly 250 m/s). This may seem fast, but it’s tiny compared to light, which travels roughly a million times faster than sound (roughly 300,000,000 m/s).
Sound requires some material in which to propagate (i.e. travel). This material sound travels through is called the medium . You can show that sound requires a medium by putting a cell phone inside a glass jar connected to a vacuum pump. As the air is removed from the jar, the cell phone’s ringer gets quieter and quieter. A youTube video (2:05 min) produced by the UNSW PhysClips project shows the demo with a drumming toy monkey [1] instead of a cell phone.
What affects the speed of sound?
Sound travels at different speeds though different materials. The physical properties of the medium are the only factors that affect the speed of sound- nothing else matters.
The speed of sound in a material is determined mainly by two properties- the stiffness of the material and the density of the material. Sound travels fastest through materials that are stiff and light. In general, sound travels fastest through solids, slower through liquids and slowest through gasses. (See the table on this page). This may seem backwards- after all, metals are quite dense. However, the high density of metals is more than offset by far greater stiffness (compared to liquids and solids).
The speed of sound in air depends mainly on temperature. The speed of sound is 331 m/s in dry air at 0 o Celsius and increases slightly with temperature- about 0.6 m/s for every 1 o Celsius for temperatures commonly found on Earth. Though speed of sound in air also depends on humidity, the effect is tiny- sound travels only about 1 m/s faster in air with 100% humidity air at 20 o C than it does in completely dry air at the same temperature.
Nothing else matters
The properties of the medium are the only factors that affect the speed of sound- nothing else matters.
Frequency of the sound does not matter- high frequency sounds travel at the same speed as low frequency sounds. If you’ve ever listened to music, you’ve witnessed this- the low notes and the high notes that were made simultaneously reach you simultaneously, even if you are far from the stage. If you’ve heard someone shout from across a field, you’ve noticed that the entire shout sound (which contains many different frequencies at once) reaches you at the same time. If different frequencies traveled at different rates, some frequencies would arrive before others.
The amplitude of the sound does not matter- loud sounds and quiet ones travel at the same speed. Whisper or yell- it doesn’t matter. The sound still takes the same amount of time to reach the listener. You’ve probably heard that you can figure out how far away the lightning by counting the seconds between when you see lightning and hear thunder. If the speed of sound depended on loudness, this rule of thumb would have to account for loudness- yet there is nothing in the rule about loud vs. quiet thunder. The rule of thumb works the same for all thunder- regardless of loudness . That’s because the speed of sound doesn’t depend on amplitude.
Stop to thinks
- Which takes longer to cross a football field: the sound of a high pitched chirp emitted by a fruit bat or the (relatively) low pitched sound emitted by a trumpet?
- Which sound takes longer to travel 100 meters: the sound of a snapping twig in the forest or the sound of a gunshot?
- Which takes longer to travel the distance of a football field: the low pitched sound of a whale or the somewhat higher pitched sound of a human being?
Constant speed
Sound travels at a constant speed. Sound does not speed up or slow down as it travels (unless the properties of the material the sound is going through changes). I know what you’re thinking- how is that possible? Sounds die out as they travel, right? True. That means sounds must slow down and come to a stop, right? Wrong. As sound travels, its amplitude decreases- but that’s not the same thing as slowing down. Sound (in air) covers roughly 340 meters each and every second, even as its amplitude shrinks. Eventually, the amplitude gets small enough that the sound is undetectable. A sound’s amplitude shrinks as it travels, but its speed remains constant.
The basic equation for constant speed motion (shown below) applies to sound.
[latex]d=vt[/latex]
In this equation, [latex]d[/latex] represents the distance traveled by the sound, [latex]t[/latex] represents the amount of time it took to go that distance and [latex]v[/latex] represents the speed.
Rule of thumb for lightning example
Example: thunder and lightning.
The rule of thumb for figuring out how far away a lightning strike is from you is this:
Count the number of seconds between when you see the lightning and hear the thunder. Divide the number of seconds by five to find out how many miles away the lightning hit.
According to this rule, what is the speed of sound in air? How does the speed of sound implied by this rule compare to 340 m/s?
Identify important physics concept : This question is about speed of sound.
List known and unknown quantities (with letter names and units):
At first glance, it doesn’t look like there’s enough information to solve the problem. We were asked to find speed, but not given either a time or a distance. However, the problem does allow us to figure out a distance if we know the time- “Divide the number of seconds by five to find out how many miles away the lightning hit.” So, let’s make up a time and see what happens; if the time is 10 seconds, the rule of thumb says that the distance should be 2 miles.
[latex]v= \: ?[/latex]
[latex]d=2 \: miles[/latex]
[latex]t=10 \: seconds[/latex]
You might ask “Is making stuff up OK here?” The answer is YES! If the rule of thumb is right, it should work no matter what time we choose. (To check if the rule is OK, we should probably test it with more than just one distance-time combination, but we’ll assume the rule is OK for now).
Do the algebra: The equation is already solved for speed. Move on to the next step.
Do unit conversions (if needed) then plug in numbers: If you just plug in the numbers, the speed comes out in miles per second:
[latex]v = \frac{2 \: miles} {10 \: seconds}=0.2 \: \frac{miles} {second}[/latex]
We are asked to compare this speed to 340 m/s, so a unit conversion is in order; since there are 1609 meters in a mile:
[latex]v =0.2 \: \frac{miles} {second}*\frac{1609 \: meters} {1 \:mile}=320 \frac{m}{s}[/latex]
Reflect on the answer:
- The answer for speed from the rule of thumb is less than 10% off the actual value of roughly 340 m/s- surprisingly close!
- At the beginning, we assumed a time of 10 seconds. Does the result hold up for other choices? A quick check shows that it does! For instance, if we use a time of 5 seconds, the rule of thumb gives a distance of 1 mile, and the math still gives a speed of 0.2 miles/second. The speed will be the same no matter what time we pick. The reason is this: The more time it takes the thunder to arrive, the farther away the lightning strike is, but the speed remains the same. In the equation for speed, both time and distance change by the same factor and the overall fraction remains unchanged.
Stop to think answers
- Both sounds take the same amount of time. (High and low pitched sounds travel at the same speed).
- Both sounds take the same amount of time. (Quiet sounds and loud sounds travel at the same speed).
- The sound of the whale travels the distance in less time- assuming sound from the whale travels in water and sound from the human travels in air. Sound travels faster in water than in air. (The info about frequency was given just to throw you off- frequency doesn’t matter).
- Wolfe, J. (2014, February 20). Properties of Sound. Retrieved from https://www.youtube.com/watch?v=P8-govgAffY ↵
Understanding Sound Copyright © by dsa2gamba and abbottds is licensed under a Creative Commons Attribution 4.0 International License , except where otherwise noted.
Share This Book
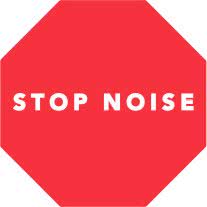
Noise Control Help Line: 1-800-854-2948 M - F 8a.m. - 5p.m. (Central Time)
Acoustical Surfaces, Inc.
Sound Proofing | Acoustics | Noise & Vibration Control

Speed of Sound: How Sound Travels Through Objects and Materials
Posted by Acoustical Surfaces on 03/14/2024 11:54 am | Leave a Comment
From the snap of a twig to the booming echo of a drumbeat, sound plays an important role in helping us navigate our space. While sound is incredibly important, many people rarely stop to ponder its physical properties.
In physics, sound is defined as an acoustic vibration that sends waves through a medium, such as a solid, liquid, or gas. 1 These mediums’ properties can influence sound’s speed. By understanding the relation between material properties and the speed of sound, you can optimize the acoustics of various spaces, from professional recording studios to company conference rooms.
We’ll be diving into the fascinating mechanics of sound propagation and explain how different objects’ density, elasticity, and temperature all influence the speed of sound (plus how to contain or absorb those fast-moving sound waves).
Table of Contents
What is the Formula for the Speed of Sound?
Many people assume that the speed of sound is a constant number, but this isn’t the case. The materials that transmit sound can influence its speed considerably. Even so, there is a general speed of sound formula, which multiplies the sound’s wavelength by its frequency. 2 In mathematical terms:
In this formula:
- v represents the speed of sound
- f is the frequency of the sound wave
- λ is the length of the sound wave
While this general formula is an excellent baseline, the speed of sound can vary greatly from one medium to the next, depending on its temperature, density, and elasticity.
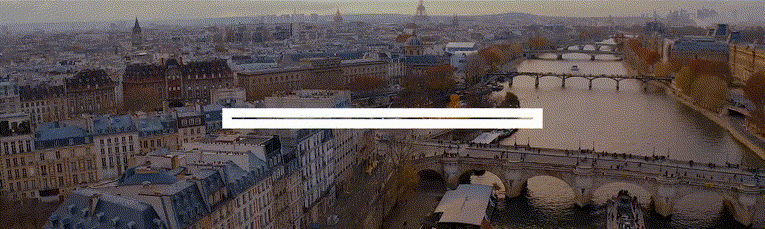
Speed of Sound: Example Equation
To clarify the connection between the sound’s speed, frequency, and wavelength, let’s take a look at a real-life example.
Let’s say that you’re measuring a sound with a frequency of 261 Hz, also known as middle C or concert pitch. 3 This sound’s wavelength is around 1.3 meters. After plugging these numbers into the speed of sound formula, you can calculate sound using the following equation:
v=(261 Hz)(1.3 m)
v=341.9 m/s
With this equation in mind, let’s take a closer look at the role of various materials’ properties on the speed of sound.
How Does a Material’s Density Impact the Speed of Sound?
Density, which measures the mass per unit volume of a given material, can influence the speed of sound significantly. That’s because density dictates how closely a material’s molecules are packed together.
Since sound is kinesthetic energy that travels by passing from one molecule to the next, materials with more densely packed molecules facilitate faster sound propagation. For this reason, sound travels faster through solids than liquids and faster through liquids than gasses.
Varying Densities of Different Elements
Denser elements often feature heavier molecules, which take more energy to vibrate, subsequently slowing down sound’s speed. As a result, sound can travel through aluminum nearly twice as fast as it moves through gold, due to the differences in the molecules. 4
How Does Material Density Relate to Soundproofing?
If you’re interested in optimizing the acoustics of a space, you’ll likely employ soundproofing solutions at some point. Soundproofing materials add mass to walls and ceilings to prevent sound from escaping.
Since these materials are often quite dense, it begs the question, “Why use dense, solid materials for soundproofing if sound waves travel through them faster?”
When dense materials are thick enough, they contain enough molecules to drain the sound wave of energy before it can reach the other side. That’s why most soundproofing solutions, from double-layer drywall to solid-core doors, are so thick.
How Does a Material’s Elasticity Impact the Speed of Sound?
Another key factor that influences the speed of sound through materials is their elasticity, which refers to a material’s ability to maintain its shape when placed under stress. For example, a rigid material like steel won’t lose its shape as easily as a more flexible material like rubber.
Materials with atoms that are strongly attracted to each other end up being more rigid, due to their powerful internal bonds. The strength of these bonds ultimately determines how quickly a material will return to its original shape.
So, what does this mean for the speed of sound? Particles that quickly regain their shape after an external force will also vibrate at higher speeds. As a result, they enable sound to travel faster than materials with lower elastic properties. 4
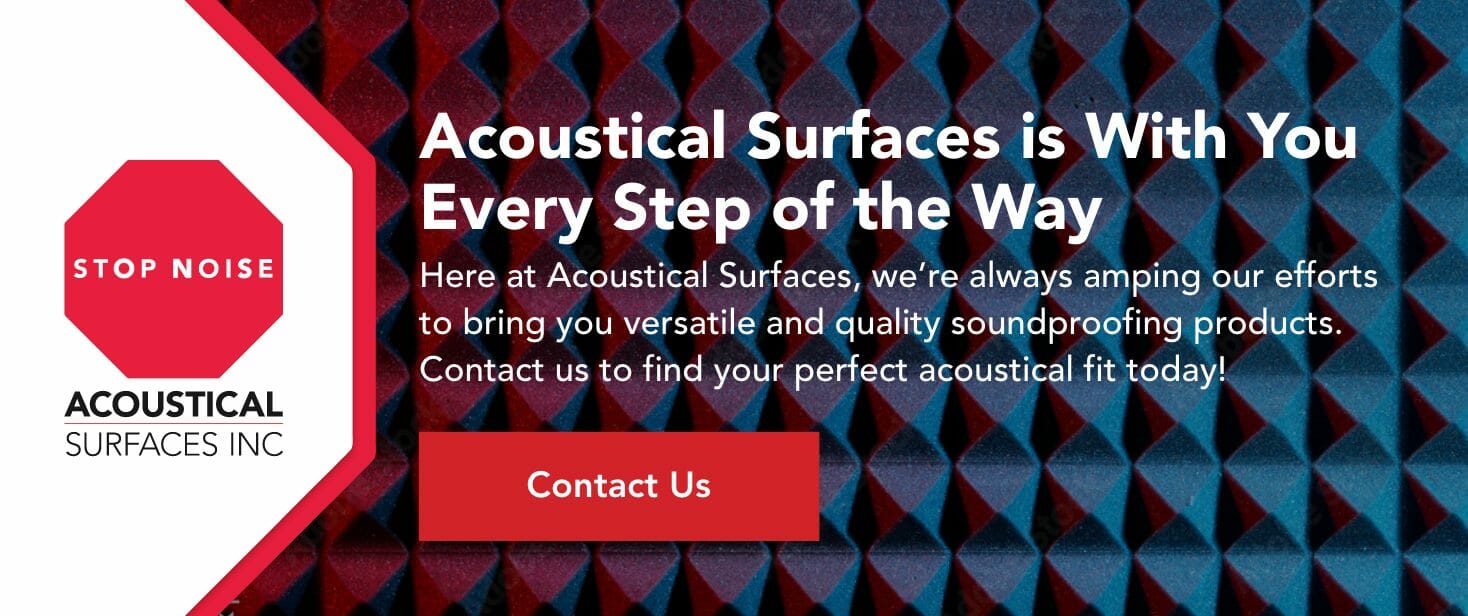
Speed of Sound Elasticity Equation
If you want to flex your mathematical muscles, you can calculate the speed of sound while taking into account different material’s elastic properties.
This more complicated formula divides the elastic property by the inertial property and takes the resulting number’s square root, as showcased by this equation 2 :
Here’s a quick breakdown:
- Elastic property is a material’s ability to deform and reform in the face of an external force.
- Inertial property looks at whether a material will stay at rest or in motion in the absence of an external force.
How Does a Material’s Temperature Impact the Speed of Sound?
Many people are often surprised to learn that temperature can influence the speed of sound. Typically, higher temperatures facilitate faster sound travel, especially through gasses.
So, what’s behind this phenomenon? Well, heat is a form of kinetic energy. Increasing the temperature speeds up the vibration of molecules within a material, and causes sound waves to jump from one molecule to the next more quickly.
This explains why room-temperature air has a speed of sound of 346 m/s, while air at water’s freezing point (0°C / 32°F) has a speed of sound of 331 m/s. 4 While this fascinating connection between thermal dynamics and acoustics is often overlooked, it can make a noteworthy difference in a room’s sound quality.
Speed of Sound Formula With Temperature
If you want to measure the average speed of sound for various temperatures, you can use this formula 5 :
v = 331 m/s + 0.61T
Here’s how the equation breaks down:
- v is the speed of sound
- 331 m/s is the speed of sound at 0°C
- 0.61 is a constant that represents the increase in sound’s speed with every additional degree
- T is the air’s temperature in Celcius
What is the Speed of Sound for Common Materials?
Now that you understand the basic components that affect the speed of sound, let’s take a look at how quickly sound moves through the following mediums 2 :
Gasses at 0°C or 32°F
- Air – 331 m/s
- Carbon dioxide – 259 m/s
- Oxygen – 316 m/s
- Helium – 965 m/s
- Hydrogen – 1,290 m/s
Liquids at 20°C or 68°F
- Ethanol – 1,160 m/s
- Mercury – 1,450 m/s
- Freshwater – 1,480 m/s
- Sea water – 1,540 m/s
- Rubber – 60 m/s
- Polyethylene – 920 m/s
- Lead – 1,210 m/s
- Gold – 3,240 m/s
- Marble – 3,810 m/s
- Copper – 4,600 m/s
- Aluminum – 5,120 m/s
- Iron – 5,120 m/s
- Glass – 5,640 m/s
- Steel – 5,960 m/s
- Diamond – 12,000 m/s
As you can see, the effect of these materials’ varying properties on the speed of sound is quite pronounced—sound travels nearly 35 times faster through a diamond than it does through air. 6
Soundproofing Solutions
The speed of sound is a complex and fascinating phenomenon, but thankfully, soundproofing solutions tend to be relatively simple.
Here are some popular soundproofing solutions we offer at Acoustical Surfaces:
- Noise S.T.O.P.™ Vinyl Barrier Mass Loaded Vinyl Barrier
- Noise S.T.O.P.™ Interior Soundproof Glass Window
- SoundBreak XP Acoustically Enhanced Gypsum Boar d
- Green Glue Viscoelastic Damping Compound
- Resilient Sound Isolation Clips (RSICs)
Sound Absorption Solutions
Soundproofing solutions have many valuable applications, but they’re not always right for your acoustical goals. Maybe you want to enhance the acoustics of a space instead, whether that involves dampening distracting echoes and reverberations or balancing the sound within a space. In this case, sound absorption is what you need.
While soundproofing materials isolate sound within a space, sound absorption materials are often soft and foamy, enabling them to soak up excess sound waves like a sponge and stop them from bouncing around, creating an unpleasant cacophony in their wake.
Some of our best-selling sound absorption products at Acoustical Surfaces are:
- Poly Max™ Acoustical Panels
- Envirocoustic™ Wood Wool Cementitious Wood-Fiber Acoustic Ceiling and Wall Panels
- Echo Eliminator Bonded Acoustical Cotton Panels
- NOISE S.T.O.P. Fabric-Wrapped Acoustical Panels
- Flat Faced Open Cell Melamine Acoustical Foam
- WALLMATE® Fabric Wall System
Learn More About Sound With Acoustical Surfaces
From music to meteorology, the speed of sound is an important concept. It’s especially relevant to understand how sound speeds change with varying levels of density, elasticity, and temperature.
By understanding the way sound travels through different materials, you can make smarter acoustical decisions. However, you don’t have to navigate this process alone—just reach out to our team of sound experts at Acoustical Surfaces . We’ve been providing comprehensive sound solutions for over 35 years.
If you want custom sound solutions for your space, whether that’s a classroom, restaurant, recording studio, or home, we can help you select the ideal products. Contact our team today to receive tailored soundproofing or sound absorption support.
- BYJU’s. Sound Waves. https://byjus.com/physics/sound-waves/
- LibreTexts. 17.3: Speed of Sound. https://phys.libretexts.org/Bookshelves/University_Physics/University_Physics_(OpenStax)/Book%3A_University_Physics_I_-_Mechanics_Sound_Oscillations_and_Waves_(OpenStax)/17%3A_Sound/17.03%3A_Speed_of_Sound
- SFU. Wavelength. https://www.sfu.ca/sonic-studio-webdav/handbook/Wavelength.html
- Iowa State University. Sound. https://www.nde-ed.org/Physics/Sound/index.xhtml
- University of Rhode Island. Sound Waves. https://penrose.uri.edu/labs/PHY275/sound_waves/sound_waves.pdf
- Inspirit. Speed Of Sound Study Guide. https://www.inspiritvr.com/speed-of-sound-study-guide/
Additional Resources
Creating better-sounding rooms.
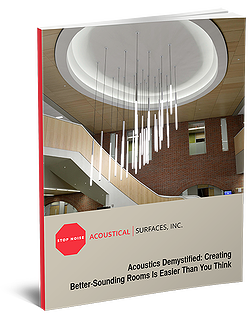
Solutions to Common Noise Problems
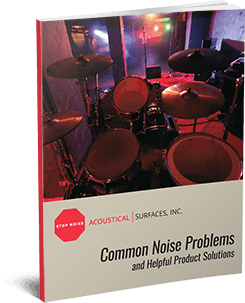
CAD, CSI, & Revit Library
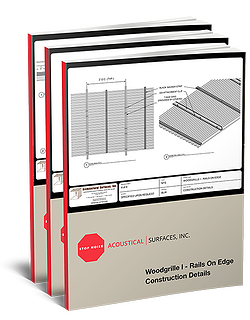
Leave a Reply Cancel reply
Your email address will not be published. Required fields are marked *
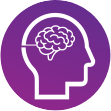
Book a free consultation now
100+ Video Tutorials, Flashcards and Weekly Seminars
- Revision notes >
- GCSE Physics Revision Notes >
- Edexcel GCSE Physics Revision Notes
Sound Waves Through Solids (GCSE Physics)
Sound waves through solids, travelling through solids.
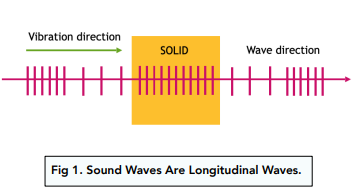
- Sound waves can be reflected and refracted. Like we will see with all other waves, sound waves can be reflected or refracted . The reflection of sound waves produces echoes.
Sound waves are a type of mechanical wave that travels through a medium, such as air, water, or solids. They are caused by the vibration of an object and are detected by our ears as sound.
In solids, sound waves are able to travel because the particles in the solid are tightly packed together. The particles vibrate back and forth, transmitting the sound wave through the material.
The difference between sound waves in solids and liquids or gases is that the particles in solids are much more tightly packed together. This means that sound waves are able to travel faster and with more energy through solids than they can through liquids or gases.
Some examples of sound waves traveling through solids include the vibration of the strings in a guitar, the vibration of the reed in a saxophone, and the vibration of the soundboard in a piano.
Sound waves are used in many everyday devices, such as speakers and microphones. They are also used in medical imaging, such as ultrasound, and in industrial testing, such as ultrasonic testing for metal fatigue.
No, sound waves cannot travel through a vacuum. This is because sound waves need a medium to travel through, and a vacuum does not provide a medium for the wave to move through.
Still got a question? Leave a comment
Leave a comment, cancel reply.
Save my name, email, and website in this browser for the next time I comment.
CIE IGCSE - 1 Motion, forces & energy
Types of waves (gcse physics), fluid pressures part 2 (gcse physics), fluid pressures part 1 (gcse physics), atmospheric pressure (gcse physics), balancing moments (gcse physics), levers & gears (gcse physics), work done in a spring (gcse physics), changes in momentum (gcse physics), forces and elasticity (gcse physics), momentum (gcse physics), cie igcse - 2 thermal physics, pressure in gases (gcse physics), specific latent heat calculations (gcse physics), specific heat capacity calculations (gcse physics), changes in thermal energy (gcse physics), energy of particles (gcse physics), changes of state (gcse physics), movement of particles (gcse physics), solids, liquids and gases (gcse physics), energy resources: heating (gcse physics), thermal radiation (gcse physics), cie igcse - 3 waves, magnification (gcse physics), convex & concave lenses (gcse physics), visible light and colours (gcse physics), appearance of objects (gcse physics), producing and absorbing radio waves (gcse physics), dangers of radiation (gcse physics), applications of electromagnetic waves (gcse physics), electromagnetic spectrum (gcse physics), properties of electromagnetic waves (gcse physics), frequency range (gcse physics), cie igcse - 4 electricity & magnetism, power input & output (gcse physics), ratio of potential differences (gcse physics), step up & step down transformers(gcse physics), transformers (gcse physics), induced potential & the generator effect(gcse physics), electric motors (gcse physics), fleming’s left hand rule (gcse physics), electromagnetism (gcse physics), magnetic fields (gcse physics), poles of a magnet (gcse physics), cie igcse - 5 nuclear physics, associated hazards and precautions (gcse physics), nuclear fusion (gcse physics), nuclear fission (gcse physics), evaluation of uses (gcse physics), uses of nuclear radiation (gcse physics), background radiation (gcse physics), radioactive irradiation and contamination (gcse physics), deflection (gcse physics), penetration and ionisation (gcse physics), alpha, beta and gamma (gcse physics), cie igcse - 6 space physics, edexcel 1 key concepts of physics, resistance (gcse physics), edexcel 10 static electricity, structure of an atom (gcse physics), transporting electrical energy (gcse physics), dangers of electricity (gcse physics), mains electricity (gcse physics), power: work done (gcse physics), alternating and direct potential difference (gcse physics), power: current and resistance (gcse physics), power: current and potential difference (gcse physics), transfers of energy (gcse physics), circuit components (gcse physics), edexcel 11 magnetism and the motor effect, sparks (gcse physics), drawing electric field patterns (gcse physics), electric fields (gcse physics), static electricity (gcse physics), charged objects (gcse physics), edexcel 12 particle model, edexcel 2 motion and forces, conservation of momentum (gcse physics), factors affecting braking distance (gcse physics), newton’s third law (gcse physics), stopping distance (gcse physics), reaction time (gcse physics), newton’s first law (gcse physics), newton’s second law (gcse physics), terminal velocity (gcse physics), edexcel 3 conservation of energy, energy resources: trends in usage (gcse physics), non-renewable energy sources (gcse physics), renewable energy sources (gcse physics), energy resources: electricity generation (gcse physics), energy resources: transport (gcse physics), reducing energy waste (gcse physics), efficiency (gcse physics), the law of conservation of energy (gcse physics), energy changes in a system (gcse physics), edexcel 4 waves, waves for detection & exploration(gcse physics), the ear (gcse physics), measuring the speed: water waves (gcse physics), measuring the speed: sound waves (gcse physics), wave speed (gcse physics), ray diagrams (gcse physics), transmission: reflection (gcse physics), transmission: refraction (gcse physics), edexcel 5 light & the electromagnetic spectrum, temperature of the earth (gcse physics), radiation and temperature (gcse physics), perfect black bodies (gcse physics), edexcel 6 radioactivity, fusion in stars (gcse physics), chain reactions (gcse physics), risks of radiation (gcse physics), measuring radiation (gcse physics), radiation dose (gcse physics), edexcel 7 astronomy, observations and questions (gcse physics), receding galaxies (gcse physics), red-shift (gcse physics), big bang theory (gcse physics), life cycle of a star (gcse physics), formation of stars (gcse physics), comparing types of orbits (gcse physics), gravity & orbits (gcse physics), orbital motion (gcse physics), our solar system (gcse physics), edexcel 8 energy, energy transfers (gcse physics), work done (gcse physics), calculating power (gcse physics), power (gcse physics), using the gravitational potential energy equation (gcse physics), edexcel 9 electricity and circuits, velocity (gcse physics), resolving parallel & non-parallel forces (gcse physics), forces in equilibrium (gcse physics), vector diagrams (gcse physics), free body diagram examples (gcse physics), types of forces (gcse physics), scalar & vector quantities (gcse physics), drawing vector diagrams (gcse physics), ocr 1.1 the particle model, charge, mass number & atomic number (gcse physics), development of the atomic model part 2 (gcse physics), development of the atomic model part 1 (gcse physics), investigating density (gcse physics), density calculations (gcse physics), density (gcse physics), ocr 1.2 changes of state, ocr 1.3 pressure, earth’s atmosphere (gcse physics), ocr 2.1 motion, calculating distance travelled (gcse physics), velocity-time graphs (gcse physics), uniform acceleration calculations (gcse physics), uniform acceleration (gcse physics), acceleration (gcse physics), curved distance-time graphs (gcse physics), distance-time graph calculations (gcse physics), distance-time relationship (gcse physics), non-uniform motion (gcse physics), speed calculation examples (gcse physics), ocr 2.2 newton’s laws, ocr 2.3 forces in action, weight and mass (gcse physics), gravitational field strength (gcse physics), weight and gravity (gcse physics), ocr 3.1 static and charge, more on static electricity (gcse physics), charging materials (gcse physics), series and parallel circuits (gcse physics), charge flow calculations (gcse physics), current (gcse physics), electrical charge (gcse physics), ocr 3.2 simple circuits, investigating current-voltage characteristics (gcse physics), thermistors and ldrs (gcse physics), current-voltage graphs (gcse physics), ocr 4.1 magnets and magnetic fields, ocr 4.2 uses of magnetism, microphones (gcse physics), loudspeakers (gcse physics), ocr 5.1 wave behaviour, waves at boundaries (gcse physics), ocr 5.2 the electromagnetic spectrum, ocr 5.3 wave interactions, ocr 6.1 radioactive emissions, types of nuclear radiation (gcse physics), radioactive decay & half life (gcse physics), spectrums (gcse physics), electromagnetic radiation (gcse physics), ionisation (gcse physics), isotopes (gcse physics), ocr 6.2 radioactivity - uses and hazards, ocr 7.1 work done, work done and power (gcse physics), gcse specific heat capacity, the elastic potential energy equation (gcse physics), elastic potential energy (gcse physics), linking the ke and gpe (gcse physics), gravitational potential energy (gcse physics), ocr 7.2 power and efficiency, power efficiency (gcse physics), energy transfers in electronic appliances (gcse physics), comparing the rate of energy transfer (gcse physics), ocr 8.1 physics on the move, speed values (gcse physics), ocr 8.2 powering earth, ocr 8.3 beyond earth, the solar system over time(gcse physics), pearson igcse - 1 forces & motion, pearson igcse - 2 electricity, pearson igcse - 3 waves, pearson igcse - 4 energy resources & transfers, thermal convection (gcse physics), thermal conduction (gcse physics), pearson igcse - 5 solids, liquids, gases, heating and cooling graphs (gcse physics), pearson igcse - 6 magnetism & electromagnetism, pearson igcse - 7 radioactivity and particles, pearson igcse - 8 astrophysics, orbital speed (gcse physics).
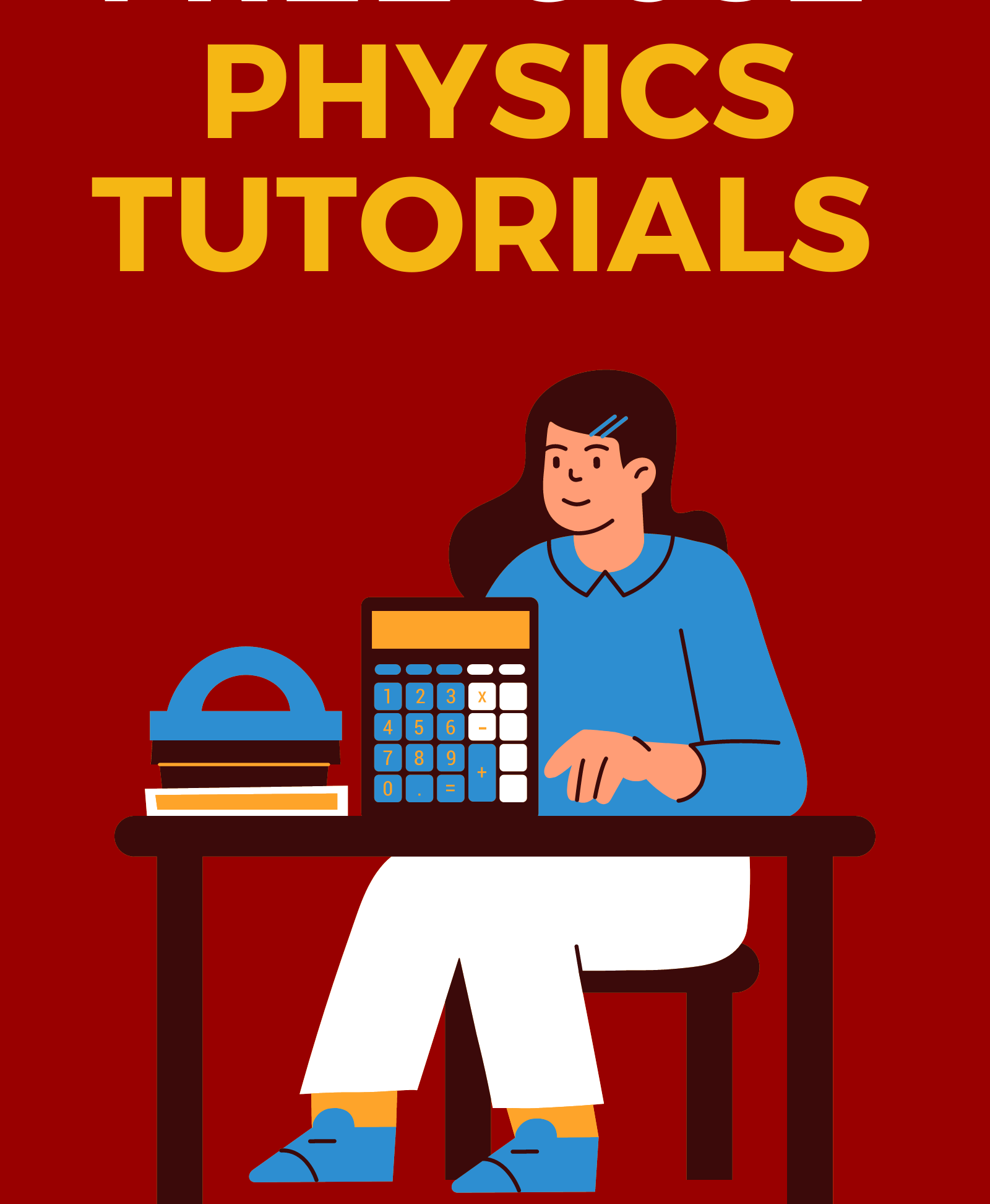
Let's get acquainted ? What is your name?
Nice to meet you, {{name}} what is your preferred e-mail address, nice to meet you, {{name}} what is your preferred phone number, what is your preferred phone number, just to check, what are you interested in, when should we call you.
It would be great to have a 15m chat to discuss a personalised plan and answer any questions
What time works best for you? (UK Time)
Pick a time-slot that works best for you ?
How many hours of 1-1 tutoring are you looking for?
My whatsapp number is..., for our safeguarding policy, please confirm....
Please provide the mobile number of a guardian/parent
Which online course are you interested in?
What is your query, you can apply for a bursary by clicking this link, sure, what is your query, thank you for your response. we will aim to get back to you within 12-24 hours., lock in a 2 hour 1-1 tutoring lesson now.
If you're ready and keen to get started click the button below to book your first 2 hour 1-1 tutoring lesson with us. Connect with a tutor from a university of your choice in minutes. (Use FAST5 to get 5% Off!)
NOTIFICATIONS
Sound – visualising sound waves.
- + Create new collection
Sound is a form of energy that is caused by the vibration of matter. Sound is transmitted through waves, which travel through solids, liquids and gases. We are most used to the sound travelling through air, but sound is able to travel faster and further in solids and liquids .
‘Seeing’ sound
If we could see the molecules that make up the air around us, we would see sound as a series of more and less dense areas of air that are moving away from the source of the sound at about 340 metres per second. We say sound is a wave because the air molecules move back and forth while the sound travels along. The air behaves much like a longitudinal or compression wave on a spring.
It is difficult to draw compression waves , so waves are generally represented as transverse waves for simplicity. The dense areas of the compression wave are the peaks of the transverse wave and the sparse areas are the troughs.
Microphone – transforming sound energy into electrical energy
In order to visualise a sound wave, we can use a microphone to transform sound energy into electrical energy. A simple microphone is made up of a very thin membrane with a coil of very fine wire attached. A magnet is positioned so that it is just inside the coil of wire but not touching it. When a sound wave strikes the membrane , it jiggles (vibrates) back and forth because of the high and low pressure areas of the wave. This causes the coil to jiggle, and when a coil moves in a magnetic field, an electrical current is produced. If we look at the electrical current using an oscilloscope, we can see the sound as a series of peaks and troughs.
The sound from single pitch or note will make a simple sine wave on the screen. The wave will change as you change the volume or pitch of the note.
Related content
This article is part of an article series :
- Sound – understanding standing waves
- Sound – resonance
- Sound – wave interference
- Sound – beats, the Doppler effect and sonic booms
with the accompanying investigations:
- Measuring the speed of sound
- Investigating sound wave resonance
Additional articles and activity ideas
Find out more about studying sound under water and read about what is needed for sound to be heard , and how sound travels through water to understand some of the key science concepts.
Investigating waves and energy uses slinkies to explore longitudinal and transverse waves.
Make and use a hydrophone explains how to construct an underwater microphone.
Sound on an oscilloscope uses a computer’s microphone to create a visual display.
The PLD article Physical World – Sound curates Hub resources for the early years through to year 10.
Visit the sound topic for additional resources.
See our newsletters here .
Would you like to take a short survey?
This survey will open in a new tab and you can fill it out after your visit to the site.

- Science Notes Posts
- Contact Science Notes
- Todd Helmenstine Biography
- Anne Helmenstine Biography
- Free Printable Periodic Tables (PDF and PNG)
- Periodic Table Wallpapers
- Interactive Periodic Table
- Periodic Table Posters
- How to Grow Crystals
- Chemistry Projects
- Fire and Flames Projects
- Holiday Science
- Chemistry Problems With Answers
- Physics Problems
- Unit Conversion Example Problems
- Chemistry Worksheets
- Biology Worksheets
- Periodic Table Worksheets
- Physical Science Worksheets
- Science Lab Worksheets
- My Amazon Books
Speed of Sound in Physics

In physics, the speed of sound is the distance traveled per unit of time by a sound wave through a medium. It is highest for stiff solids and lowest for gases. There is no sound or speed of sound in a vacuum because sound (unlike light ) requires a medium in order to propogate.
What Is the Speed of Sound?
Usually, conversations about the speed of sound refer to the speed of sound of dry air (humidity changes the value). The value depends on temperature.
- at 20 ° C or 68 ° F: 343 m/s or 1234.8 kph or 1125ft/s or 767 mph
- at 0 ° C or 32 ° F: 331 m/s or 1191.6 kph or 1086 ft/s or 740 mph
Mach Numher
The Mach number is the ratio of air speed to the speed of sound. So, an object at Mach 1 is traveling at the speed of sound. Exceeding Mach 1 is breaking the sound barrier or is supersonic . At Mach 2, the object travels twice the speed of sound. Mach 3 is three times the speed of sound, and so on.
Remember that the speed of sound depends on temperature, so you break sound barrier at a lower speed when the temperature is colder. To put it another way, it gets colder as you get higher in the atmosphere, so an aircraft might break the sound barrier at a higher altitude even if it does not increase its speed.
Solids, Liquids, and Gases
The speed of sound is greatest for solids, intermediate for liquids, and lowest for gases:
v solid > v liquid >v gas
Particles in a gas undergo elastic collisions and the particles are widely separated. In contrast, particles in a solid are locked into place (rigid or stiff), so a vibration readily transmits through chemical bonds.
Here are examples of the difference between the speed of sound in different materials:
- Diamond (solid): 12000 m/s
- Copper (solid): 6420 m/s
- Iron (solid): 5120 m/s
- Water (liquid) 1481 m/s
- Helium (gas): 965 m/s
- Dry air (gas): 343 m/s
Sounds waves transfer energy to matter via a compression wave (in all phases) and also shear wave (in solids). The pressure disturbs a particle, which then impacts its neighbor, and continues traveling through the medium. The speed is how quickly the wave moves, while the frequency is the number of vibrations the particle makes per unit of time.
The Hot Chocolate Effect
The hot chocolate effect describes the phenomenon where the pitch you hear from tapping a cup of hot liquid rises after adding a soluble powder (like cocoa powder into hot water). Stirring in the powder introduces gas bubbles that reduce the speed of sound of the liquid and lower the frequency (pitch) of the waves. Once the bubbles clear, the speed of sound and the frequency increase again.
Speed of Sound Formulas
There are several formulas for calculating the speed of sound. Here are a few of the most common ones:
For gases these approximations work in most situations:
For this formula, use the Celsius temperature of the gas.
v = 331 m/s + (0.6 m/s/C)•T
Here is another common formula:
v = (γRT) 1/2
- γ is the ratio of specific heat values or adiabatic index (1.4 for air at STP )
- R is a gas constant (282 m 2 /s 2 /K for air)
- T is the absolute temperature (Kelvin)
The Newton-Laplace formula works for both gases and liquids (fluids):
v = (K s /ρ) 1/2
- K s is the coefficient of stiffness or bulk modulus of elasticity for gases
- ρ is the density of the material
So solids, the situation is more complicated because shear waves play into the formula. There can be sound waves with different velocities, depending on the mode of deformation. The simplest formula is for one-dimensional solids, like a long rod of a material:
v = (E/ρ) 1/2
- E is Young’s modulus
Note that the speed of sound decreases with density! It increases according to the stiffness of a medium. This is not intuitively obvious, since often a dense material is also stiff. But, consider that the speed of sound in a diamond is much faster than the speed in iron. Diamond is less dense than iron and also stiffer.
Factors That Affect the Speed of Sound
The primary factors affecting the speed of sound of a fluid (gas or liquid) are its temperature and its chemical composition. There is a weak dependence on frequency and atmospheric pressure that is omitted from the simplest equations.
While sound travels only as compression waves in a fluid, it also travels as shear waves in a solid. So, a solid’s stiffness, density, and compressibility also factor into the speed of sound.
Speed of Sound on Mars
Thanks to the Perseverance rover, scientists know the speed of sound on Mars. The Martian atmosphere is much colder than Earth’s, its thin atmosphere has a much lower pressure, and it consists mainly of carbon dioxide rather than nitrogen. As expected, the speed of sound on Mars is slower than on Earth. It travels at around 240 m/s or about 30% slower than on Earth.
What scientists did not expect is that the speed of sound varies for different frequencies. A high pitched sound, like from the rover’s laser, travels faster at around 250 m/s. So, for example, if you listened to a symphony recording from a distance on Mars you’d hear the various instruments at different times. The explanation has to do with the vibrational modes of carbon dioxide, the primary component of the Martian atmosphere. Also, it’s worth noting that the atmospheric pressure is so low that there really isn’t any much sound at all from a source more than a few meters away.
Speed of Sound Example Problems
Find the speed of sound on a cold day when the temperature is 2 ° C.
The simplest formula for finding the answer is the approximation:
v = 331 m/s + (0.6 m/s/C) • T
Since the given temperature is already in Celsius, just plug in the value:
v = 331 m/s + (0.6 m/s/C) • 2 C = 331 m/s + 1.2 m/s = 332.2 m/s
You’re hiking in a canyon, yell “hello”, and hear an echo after 1.22 seconds. The air temperature is 20 ° C. How far away is the canyon wall?
The first step is finding the speed of sound at the temperature:
v = 331 m/s + (0.6 m/s/C) • T v = 331 m/s + (0.6 m/s/C) • 20 C = 343 m/s (which you might have memorized as the usual speed of sound)
Next, find the distance using the formula:
d = v• T d = 343 m/s • 1.22 s = 418.46 m
But, this is the round-trip distance! The distance to the canyon wall is half of this or 209 meters.
If you double the frequency of sound, it double the speed of its waves. True or false?
This is (mostly) false. Doubling the frequency halves the wavelength, but the speed depends on the properties of the medium and not its frequency or wavelength. Frequency only affects the speed of sound for certain media (like the carbon dioxide atmosphere of Mars).
- Everest, F. (2001). The Master Handbook of Acoustics . New York: McGraw-Hill. ISBN 978-0-07-136097-5.
- Kinsler, L.E.; Frey, A.R.; Coppens, A.B.; Sanders, J.V. (2000). Fundamentals of Acoustics (4th ed.). New York: John Wiley & Sons. ISBN 0-471-84789-5.
- Maurice, S.; et al. (2022). “In situ recording of Mars soundscape:. Nature. 605: 653-658. doi: 10.1038/s41586-022-04679-0
- Wong, George S. K.; Zhu, Shi-ming (1995). “Speed of sound in seawater as a function of salinity, temperature, and pressure”. The Journal of the Acoustical Society of America . 97 (3): 1732. doi: 10.1121/1.413048
Related Posts
- Anatomy & Physiology
- Astrophysics
- Earth Science
- Environmental Science
- Organic Chemistry
- Precalculus
- Trigonometry
- English Grammar
- U.S. History
- World History
... and beyond
- Socratic Meta
- Featured Answers

How do sound waves travel through solids?

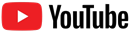
- TPC and eLearning
- Read Watch Interact
- What's NEW at TPC?
- Practice Review Test
- Teacher-Tools
- Subscription Selection
- Seat Calculator
- Ad Free Account
- Edit Profile Settings
- Classes (Version 2)
- Student Progress Edit
- Task Properties
- Export Student Progress
- Task, Activities, and Scores
- Metric Conversions Questions
- Metric System Questions
- Metric Estimation Questions
- Significant Digits Questions
- Proportional Reasoning
- Acceleration
- Distance-Displacement
- Dots and Graphs
- Graph That Motion
- Match That Graph
- Name That Motion
- Motion Diagrams
- Pos'n Time Graphs Numerical
- Pos'n Time Graphs Conceptual
- Up And Down - Questions
- Balanced vs. Unbalanced Forces
- Change of State
- Force and Motion
- Mass and Weight
- Match That Free-Body Diagram
- Net Force (and Acceleration) Ranking Tasks
- Newton's Second Law
- Normal Force Card Sort
- Recognizing Forces
- Air Resistance and Skydiving
- Solve It! with Newton's Second Law
- Which One Doesn't Belong?
- Component Addition Questions
- Head-to-Tail Vector Addition
- Projectile Mathematics
- Trajectory - Angle Launched Projectiles
- Trajectory - Horizontally Launched Projectiles
- Vector Addition
- Vector Direction
- Which One Doesn't Belong? Projectile Motion
- Forces in 2-Dimensions
- Being Impulsive About Momentum
- Explosions - Law Breakers
- Hit and Stick Collisions - Law Breakers
- Case Studies: Impulse and Force
- Impulse-Momentum Change Table
- Keeping Track of Momentum - Hit and Stick
- Keeping Track of Momentum - Hit and Bounce
- What's Up (and Down) with KE and PE?
- Energy Conservation Questions
- Energy Dissipation Questions
- Energy Ranking Tasks
- LOL Charts (a.k.a., Energy Bar Charts)
- Match That Bar Chart
- Words and Charts Questions
- Name That Energy
- Stepping Up with PE and KE Questions
- Case Studies - Circular Motion
- Circular Logic
- Forces and Free-Body Diagrams in Circular Motion
- Gravitational Field Strength
- Universal Gravitation
- Angular Position and Displacement
- Linear and Angular Velocity
- Angular Acceleration
- Rotational Inertia
- Balanced vs. Unbalanced Torques
- Getting a Handle on Torque
- Torque-ing About Rotation
- Properties of Matter
- Fluid Pressure
- Buoyant Force
- Sinking, Floating, and Hanging
- Pascal's Principle
- Flow Velocity
- Bernoulli's Principle
- Balloon Interactions
- Charge and Charging
- Charge Interactions
- Charging by Induction
- Conductors and Insulators
- Coulombs Law
- Electric Field
- Electric Field Intensity
- Polarization
- Case Studies: Electric Power
- Know Your Potential
- Light Bulb Anatomy
- I = ∆V/R Equations as a Guide to Thinking
- Parallel Circuits - ∆V = I•R Calculations
- Resistance Ranking Tasks
- Series Circuits - ∆V = I•R Calculations
- Series vs. Parallel Circuits
- Equivalent Resistance
- Period and Frequency of a Pendulum
- Pendulum Motion: Velocity and Force
- Energy of a Pendulum
- Period and Frequency of a Mass on a Spring
- Horizontal Springs: Velocity and Force
- Vertical Springs: Velocity and Force
- Energy of a Mass on a Spring
- Decibel Scale
- Frequency and Period
- Closed-End Air Columns
- Name That Harmonic: Strings
- Rocking the Boat
- Wave Basics
- Matching Pairs: Wave Characteristics
- Wave Interference
- Waves - Case Studies
- Color Addition and Subtraction
- Color Filters
- If This, Then That: Color Subtraction
- Light Intensity
- Color Pigments
- Converging Lenses
- Curved Mirror Images
- Law of Reflection
- Refraction and Lenses
- Total Internal Reflection
- Who Can See Who?
- Formulas and Atom Counting
- Atomic Models
- Bond Polarity
- Entropy Questions
- Cell Voltage Questions
- Heat of Formation Questions
- Reduction Potential Questions
- Oxidation States Questions
- Measuring the Quantity of Heat
- Hess's Law
- Oxidation-Reduction Questions
- Galvanic Cells Questions
- Thermal Stoichiometry
- Molecular Polarity
- Quantum Mechanics
- Balancing Chemical Equations
- Bronsted-Lowry Model of Acids and Bases
- Classification of Matter
- Collision Model of Reaction Rates
- Density Ranking Tasks
- Dissociation Reactions
- Complete Electron Configurations
- Elemental Measures
- Enthalpy Change Questions
- Equilibrium Concept
- Equilibrium Constant Expression
- Equilibrium Calculations - Questions
- Equilibrium ICE Table
- Intermolecular Forces Questions
- Ionic Bonding
- Lewis Electron Dot Structures
- Limiting Reactants
- Line Spectra Questions
- Mass Stoichiometry
- Measurement and Numbers
- Metals, Nonmetals, and Metalloids
- Metric Estimations
- Metric System
- Molarity Ranking Tasks
- Mole Conversions
- Name That Element
- Names to Formulas
- Names to Formulas 2
- Nuclear Decay
- Particles, Words, and Formulas
- Periodic Trends
- Precipitation Reactions and Net Ionic Equations
- Pressure Concepts
- Pressure-Temperature Gas Law
- Pressure-Volume Gas Law
- Chemical Reaction Types
- Significant Digits and Measurement
- States Of Matter Exercise
- Stoichiometry Law Breakers
- Stoichiometry - Math Relationships
- Subatomic Particles
- Spontaneity and Driving Forces
- Gibbs Free Energy
- Volume-Temperature Gas Law
- Acid-Base Properties
- Energy and Chemical Reactions
- Chemical and Physical Properties
- Valence Shell Electron Pair Repulsion Theory
- Writing Balanced Chemical Equations
- Mission CG1
- Mission CG10
- Mission CG2
- Mission CG3
- Mission CG4
- Mission CG5
- Mission CG6
- Mission CG7
- Mission CG8
- Mission CG9
- Mission EC1
- Mission EC10
- Mission EC11
- Mission EC12
- Mission EC2
- Mission EC3
- Mission EC4
- Mission EC5
- Mission EC6
- Mission EC7
- Mission EC8
- Mission EC9
- Mission RL1
- Mission RL2
- Mission RL3
- Mission RL4
- Mission RL5
- Mission RL6
- Mission KG7
- Mission RL8
- Mission KG9
- Mission RL10
- Mission RL11
- Mission RM1
- Mission RM2
- Mission RM3
- Mission RM4
- Mission RM5
- Mission RM6
- Mission RM8
- Mission RM10
- Mission LC1
- Mission RM11
- Mission LC2
- Mission LC3
- Mission LC4
- Mission LC5
- Mission LC6
- Mission LC8
- Mission SM1
- Mission SM2
- Mission SM3
- Mission SM4
- Mission SM5
- Mission SM6
- Mission SM8
- Mission SM10
- Mission KG10
- Mission SM11
- Mission KG2
- Mission KG3
- Mission KG4
- Mission KG5
- Mission KG6
- Mission KG8
- Mission KG11
- Mission F2D1
- Mission F2D2
- Mission F2D3
- Mission F2D4
- Mission F2D5
- Mission F2D6
- Mission KC1
- Mission KC2
- Mission KC3
- Mission KC4
- Mission KC5
- Mission KC6
- Mission KC7
- Mission KC8
- Mission AAA
- Mission SM9
- Mission LC7
- Mission LC9
- Mission NL1
- Mission NL2
- Mission NL3
- Mission NL4
- Mission NL5
- Mission NL6
- Mission NL7
- Mission NL8
- Mission NL9
- Mission NL10
- Mission NL11
- Mission NL12
- Mission MC1
- Mission MC10
- Mission MC2
- Mission MC3
- Mission MC4
- Mission MC5
- Mission MC6
- Mission MC7
- Mission MC8
- Mission MC9
- Mission RM7
- Mission RM9
- Mission RL7
- Mission RL9
- Mission SM7
- Mission SE1
- Mission SE10
- Mission SE11
- Mission SE12
- Mission SE2
- Mission SE3
- Mission SE4
- Mission SE5
- Mission SE6
- Mission SE7
- Mission SE8
- Mission SE9
- Mission VP1
- Mission VP10
- Mission VP2
- Mission VP3
- Mission VP4
- Mission VP5
- Mission VP6
- Mission VP7
- Mission VP8
- Mission VP9
- Mission WM1
- Mission WM2
- Mission WM3
- Mission WM4
- Mission WM5
- Mission WM6
- Mission WM7
- Mission WM8
- Mission WE1
- Mission WE10
- Mission WE2
- Mission WE3
- Mission WE4
- Mission WE5
- Mission WE6
- Mission WE7
- Mission WE8
- Mission WE9
- Vector Walk Interactive
- Name That Motion Interactive
- Kinematic Graphing 1 Concept Checker
- Kinematic Graphing 2 Concept Checker
- Graph That Motion Interactive
- Two Stage Rocket Interactive
- Rocket Sled Concept Checker
- Force Concept Checker
- Free-Body Diagrams Concept Checker
- Free-Body Diagrams The Sequel Concept Checker
- Skydiving Concept Checker
- Elevator Ride Concept Checker
- Vector Addition Concept Checker
- Vector Walk in Two Dimensions Interactive
- Name That Vector Interactive
- River Boat Simulator Concept Checker
- Projectile Simulator 2 Concept Checker
- Projectile Simulator 3 Concept Checker
- Hit the Target Interactive
- Turd the Target 1 Interactive
- Turd the Target 2 Interactive
- Balance It Interactive
- Go For The Gold Interactive
- Egg Drop Concept Checker
- Fish Catch Concept Checker
- Exploding Carts Concept Checker
- Collision Carts - Inelastic Collisions Concept Checker
- Its All Uphill Concept Checker
- Stopping Distance Concept Checker
- Chart That Motion Interactive
- Roller Coaster Model Concept Checker
- Uniform Circular Motion Concept Checker
- Horizontal Circle Simulation Concept Checker
- Vertical Circle Simulation Concept Checker
- Race Track Concept Checker
- Gravitational Fields Concept Checker
- Orbital Motion Concept Checker
- Angular Acceleration Concept Checker
- Balance Beam Concept Checker
- Torque Balancer Concept Checker
- Aluminum Can Polarization Concept Checker
- Charging Concept Checker
- Name That Charge Simulation
- Coulomb's Law Concept Checker
- Electric Field Lines Concept Checker
- Put the Charge in the Goal Concept Checker
- Circuit Builder Concept Checker (Series Circuits)
- Circuit Builder Concept Checker (Parallel Circuits)
- Circuit Builder Concept Checker (∆V-I-R)
- Circuit Builder Concept Checker (Voltage Drop)
- Equivalent Resistance Interactive
- Pendulum Motion Simulation Concept Checker
- Mass on a Spring Simulation Concept Checker
- Particle Wave Simulation Concept Checker
- Boundary Behavior Simulation Concept Checker
- Slinky Wave Simulator Concept Checker
- Simple Wave Simulator Concept Checker
- Wave Addition Simulation Concept Checker
- Standing Wave Maker Simulation Concept Checker
- Color Addition Concept Checker
- Painting With CMY Concept Checker
- Stage Lighting Concept Checker
- Filtering Away Concept Checker
- InterferencePatterns Concept Checker
- Young's Experiment Interactive
- Plane Mirror Images Interactive
- Who Can See Who Concept Checker
- Optics Bench (Mirrors) Concept Checker
- Name That Image (Mirrors) Interactive
- Refraction Concept Checker
- Total Internal Reflection Concept Checker
- Optics Bench (Lenses) Concept Checker
- Kinematics Preview
- Velocity Time Graphs Preview
- Moving Cart on an Inclined Plane Preview
- Stopping Distance Preview
- Cart, Bricks, and Bands Preview
- Fan Cart Study Preview
- Friction Preview
- Coffee Filter Lab Preview
- Friction, Speed, and Stopping Distance Preview
- Up and Down Preview
- Projectile Range Preview
- Ballistics Preview
- Juggling Preview
- Marshmallow Launcher Preview
- Air Bag Safety Preview
- Colliding Carts Preview
- Collisions Preview
- Engineering Safer Helmets Preview
- Push the Plow Preview
- Its All Uphill Preview
- Energy on an Incline Preview
- Modeling Roller Coasters Preview
- Hot Wheels Stopping Distance Preview
- Ball Bat Collision Preview
- Energy in Fields Preview
- Weightlessness Training Preview
- Roller Coaster Loops Preview
- Universal Gravitation Preview
- Keplers Laws Preview
- Kepler's Third Law Preview
- Charge Interactions Preview
- Sticky Tape Experiments Preview
- Wire Gauge Preview
- Voltage, Current, and Resistance Preview
- Light Bulb Resistance Preview
- Series and Parallel Circuits Preview
- Thermal Equilibrium Preview
- Linear Expansion Preview
- Heating Curves Preview
- Electricity and Magnetism - Part 1 Preview
- Electricity and Magnetism - Part 2 Preview
- Vibrating Mass on a Spring Preview
- Period of a Pendulum Preview
- Wave Speed Preview
- Slinky-Experiments Preview
- Standing Waves in a Rope Preview
- Sound as a Pressure Wave Preview
- DeciBel Scale Preview
- DeciBels, Phons, and Sones Preview
- Sound of Music Preview
- Shedding Light on Light Bulbs Preview
- Models of Light Preview
- Electromagnetic Radiation Preview
- Electromagnetic Spectrum Preview
- EM Wave Communication Preview
- Digitized Data Preview
- Light Intensity Preview
- Concave Mirrors Preview
- Object Image Relations Preview
- Snells Law Preview
- Reflection vs. Transmission Preview
- Magnification Lab Preview
- Reactivity Preview
- Ions and the Periodic Table Preview
- Periodic Trends Preview
- Intermolecular Forces Preview
- Melting Points and Boiling Points Preview
- Reaction Rates Preview
- Ammonia Factory Preview
- Stoichiometry Preview
- Nuclear Chemistry Preview
- Gaining Teacher Access
- Tasks and Classes
- Tasks - Classic
- Subscription
- Subscription Locator
- 1-D Kinematics
- Newton's Laws
- Vectors - Motion and Forces in Two Dimensions
- Momentum and Its Conservation
- Work and Energy
- Circular Motion and Satellite Motion
- Thermal Physics
- Static Electricity
- Electric Circuits
- Vibrations and Waves
- Sound Waves and Music
- Light and Color
- Reflection and Mirrors
- About the Physics Interactives
- Task Tracker
- Usage Policy
- Newtons Laws
- Vectors and Projectiles
- Forces in 2D
- Momentum and Collisions
- Circular and Satellite Motion
- Balance and Rotation
- Electromagnetism
- Waves and Sound
- Atomic Physics
- Forces in Two Dimensions
- Work, Energy, and Power
- Circular Motion and Gravitation
- Sound Waves
- 1-Dimensional Kinematics
- Circular, Satellite, and Rotational Motion
- Einstein's Theory of Special Relativity
- Waves, Sound and Light
- QuickTime Movies
- About the Concept Builders
- Pricing For Schools
- Directions for Version 2
- Measurement and Units
- Relationships and Graphs
- Rotation and Balance
- Vibrational Motion
- Reflection and Refraction
- Teacher Accounts
- Task Tracker Directions
- Kinematic Concepts
- Kinematic Graphing
- Wave Motion
- Sound and Music
- About CalcPad
- 1D Kinematics
- Vectors and Forces in 2D
- Simple Harmonic Motion
- Rotational Kinematics
- Rotation and Torque
- Rotational Dynamics
- Electric Fields, Potential, and Capacitance
- Transient RC Circuits
- Light Waves
- Units and Measurement
- Stoichiometry
- Molarity and Solutions
- Thermal Chemistry
- Acids and Bases
- Kinetics and Equilibrium
- Solution Equilibria
- Oxidation-Reduction
- Nuclear Chemistry
- NGSS Alignments
- 1D-Kinematics
- Projectiles
- Circular Motion
- Magnetism and Electromagnetism
- Graphing Practice
- About the ACT
- ACT Preparation
- For Teachers
- Other Resources
- Newton's Laws of Motion
- Work and Energy Packet
- Static Electricity Review
- Solutions Guide
- Solutions Guide Digital Download
- Motion in One Dimension
- Work, Energy and Power
- Frequently Asked Questions
- Purchasing the Download
- Purchasing the CD
- Purchasing the Digital Download
- About the NGSS Corner
- NGSS Search
- Force and Motion DCIs - High School
- Energy DCIs - High School
- Wave Applications DCIs - High School
- Force and Motion PEs - High School
- Energy PEs - High School
- Wave Applications PEs - High School
- Crosscutting Concepts
- The Practices
- Physics Topics
- NGSS Corner: Activity List
- NGSS Corner: Infographics
- About the Toolkits
- Position-Velocity-Acceleration
- Position-Time Graphs
- Velocity-Time Graphs
- Newton's First Law
- Newton's Second Law
- Newton's Third Law
- Terminal Velocity
- Projectile Motion
- Forces in 2 Dimensions
- Impulse and Momentum Change
- Momentum Conservation
- Work-Energy Fundamentals
- Work-Energy Relationship
- Roller Coaster Physics
- Satellite Motion
- Electric Fields
- Circuit Concepts
- Series Circuits
- Parallel Circuits
- Describing-Waves
- Wave Behavior Toolkit
- Standing Wave Patterns
- Resonating Air Columns
- Wave Model of Light
- Plane Mirrors
- Curved Mirrors
- Teacher Guide
- Using Lab Notebooks
- Current Electricity
- Light Waves and Color
- Reflection and Ray Model of Light
- Refraction and Ray Model of Light
- Classes (Legacy Version)
- Teacher Resources
- Subscriptions

- Newton's Laws
- Einstein's Theory of Special Relativity
- About Concept Checkers
- School Pricing
- Newton's Laws of Motion
- Newton's First Law
- Newton's Third Law
Sound as a Longitudinal Wave
- Sound is a Mechanical Wave
- Sound is a Longitudinal Wave
- Sound is a Pressure Wave
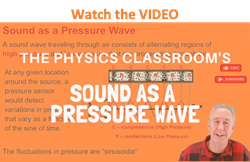
Sound waves in air (and any fluid medium) are longitudinal waves because particles of the medium through which the sound is transported vibrate parallel to the direction that the sound wave moves. A vibrating string can create longitudinal waves as depicted in the animation below. As the vibrating string moves in the forward direction, it begins to push upon surrounding air molecules, moving them to the right towards their nearest neighbor. This causes the air molecules to the right of the string to be compressed into a small region of space. As the vibrating string moves in the reverse direction (leftward), it lowers the pressure of the air immediately to its right, thus causing air molecules to move back leftward. The lower pressure to the right of the string causes air molecules in that region immediately to the right of the string to expand into a large region of space. The back and forth vibration of the string causes individual air molecules (or a layer of air molecules) in the region immediately to the right of the string to continually vibrate back and forth horizontally. The molecules move rightward as the string moves rightward and then leftward as the string moves leftward. These back and forth vibrations are imparted to adjacent neighbors by particle-to-particle interaction. Other surrounding particles begin to move rightward and leftward, thus sending a wave to the right. Since air molecules (the particles of the medium) are moving in a direction that is parallel to the direction that the wave moves, the sound wave is referred to as a longitudinal wave. The result of such longitudinal vibrations is the creation of compressions and rarefactions within the air.
Regardless of the source of the sound wave - whether it is a vibrating string or the vibrating tines of a tuning fork - sound waves traveling through air are longitudinal waves. And the essential characteristic of a longitudinal wave that distinguishes it from other types of waves is that the particles of the medium move in a direction parallel to the direction of energy transport.
We Would Like to Suggest ...
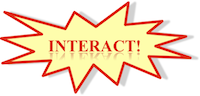
- Pitch and Frequency
- Skip to primary navigation
- Skip to main content
- Skip to footer
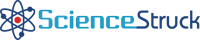
Science Struck
How Does Sound Travel? Here’s the Science Behind This Concept
When sound waves travel through a medium, the particles of the medium vibrate. Vibrations reach the ear and then the brain which senses them and we recognize sound. Read on for an explanation of how sound travels.
Like it? Share it!
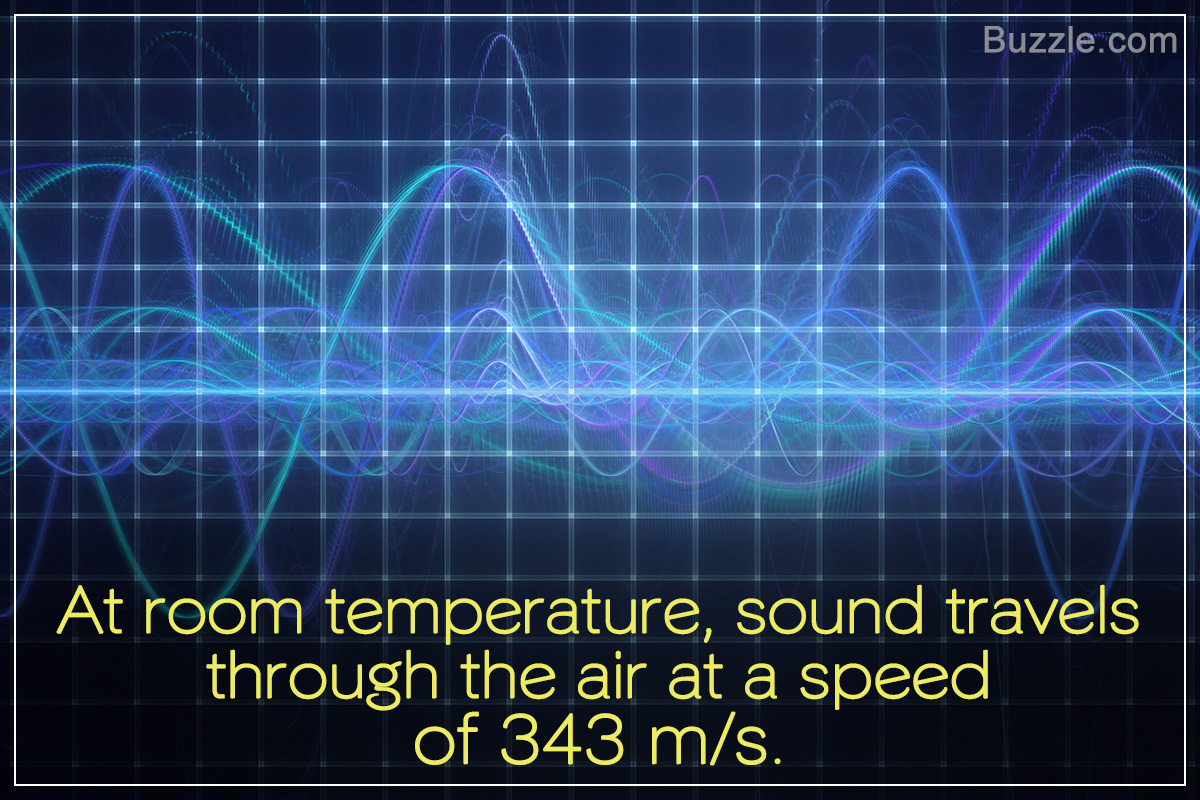
Sound is a series of compression and rarefraction waves that can travel long distances. It is produced by the vibration of the particles present in its medium; a medium is the material through which sound can travel. Presence of a medium is a must for the movement of sound waves. There are various types of medium through which sound waves can move like solids, liquids, gases, plasma, etc. Sound cannot travel through vacuum.
Characteristics of Sound Waves
The speed and the physical characteristics of sound largely varies with the change in its ambient conditions. The speed of sound depends on the density of the medium though which it is traveling. If its density is quite high, then sound would travel at a faster pace. When sound travels through gaseous medium, its speed varies with respect to changes in temperature.
The frequency of sound waves is nothing but the total number of vibrations that have been produced. The length of sound waves vary according to its frequency. Sound waves with long wavelengths have low frequency or low pitch; and those with short wavelengths have high frequency or high pitch. Our ears are capable of hearing only those sound waves which lie in the range between 20 and 20,000 vibrations per second.
How do Sound Waves Travel?
Basically, there are three things that are required for the transmission of sound. They are: a source that can transmit the sound, a medium through which sound can pass (like, water, air, etc.), and the receiver or the detector which receives the sound. The traveling process of sound has been explained below.
Creation of Sound
When a physical object moves in air, it causes vibrations which leads to formation of a series of compression waves in the air. These waves travel in the form of sound. For instance, when we strum the strings of a guitar or hit the head of a drum, the to-and-fro motion of the strings or the drum head creates compression waves of sound in the surrounding air. Similarly, when we speak, our vocal cords vibrate and the sound is created. This type of vibration occurs not just in atmospheric air but in other mediums like, solids and liquids as well. For instance, when a train is moving on a railroad made up of steel, the sound waves thus produced travel via these tracks.
At room temperature, sound travels through air with a speed of 343 m/s, through water at 1,482 m/s, and through steel at 5,960 m/s. As you can see, sound waves travel in a gaseous medium at a slow pace because its molecules are loosely bound and have to cover a long distance to collide with another molecule. In solid medium, the atoms are so closely packed that the vibration is readily transmitted to the neighboring atoms, and sound travels quite fast. In liquid medium, the bonding between the component particles are not as strong as in solids. Therefore, the sound waves move through it at a less speed as compared to solid.
Detection of Sound
When the sound waves hit the receiver, it causes some vibration in that object. The detector captures just a part of the energy from the moving sound wave. This energy of vibration is then converted to electrical signals. Thus, when the sound waves reach our ears, the eardrum present inside it vibrates. This vibration reaches our inner ear and is converted into nerve signals. As a result, we can hear the sound. Devices like microphone can detect sound. The sound waves create vibrations in its membrane which forms electrical signals that gets amplified and recorded.
So, how does sound travel? Vibration of an object causes vibrations of the same frequency in the surrounding medium. The vibrations are sent to the inner ear. After the auditory nerve picks up these vibrations, electrical signals are sent to the brain where the vibrations are recognized as sound.
Get Updates Right to Your Inbox
Privacy overview.

Advertisement
Can humans hear in space?
- Share Content on Facebook
- Share Content on LinkedIn
- Share Content on Flipboard
- Share Content on Reddit
- Share Content via Email
How Sound Travels
Sound travels in mechanical waves . A mechanical wave is a disturbance that moves and transports energy from one place to another through a medium . In sound, the disturbance is a vibrating object. And the medium can be any series of interconnected and interactive particles. This means that sound can travel through gases, liquids and solids.
Let's take a look at an example. Imagine a church bell. When a bell rings, it vibrates, which means the bell itself flexes inward and outward very rapidly. As the bell moves outward, it pushes against particles of air. Those air particles then push against other adjacent air particles, and so on. As the bell flexes inward, it pulls against the adjacent air particles, and they, in turn, pull against other air particles. This push and pull pattern is a sound wave. The vibrating bell is the original disturbance, and the air particles are the medium.
The bell's vibrations push and pull against adjacent air
Molecules, creating a sound wave..
Sound isn't restricted to moving through the air. Press your ear against a solid surface like a table and close your eyes. Tell someone else to tap his or her finger on the other end of the table. The tapping becomes the initial disturbance. Each tap sends vibrations through the table. The particles in the table collide with each other and become the medium for the sound. The particles in the table collide with air particles between the table and your eardrum . When a wave moves from one medium to another like this, it's called transmission .
The air particles collide with your ear's tympanic membrane , also known as the eardrum. This sets off a series of vibrations in several structures inside the ear. The brain interprets these vibrations as sounds. The whole process is pretty complex. You can learn more in How Hearing Works .
So, sound needs a physical medium in order to travel anywhere. Is there enough physical material in space to act as a medium for sound waves? Find out in the next section.
The speed of a sound wave depends upon the medium through which it travels. In general, sound travels faster through solids than through liquids or gases. Also, the denser the medium, the slower sound will travel through it. The same sound will travel at a different speed on a cold day than it would on a warm day.
Please copy/paste the following text to properly cite this HowStuffWorks.com article:
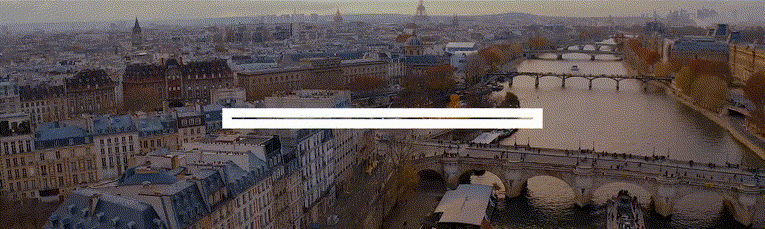
IMAGES
VIDEO
COMMENTS
After solids, liquids have the highest speed of sound. And then finally gas, that included our air since it is made up of gasses. When a sound is traveling through one medium like air and then encounters another, like a solid door, it loses some of its energy and some of the volume will be lost.
For example, let's consider a metal like iron. Iron is definitely more rigid and stiff than air so it has a much larger bulk modulus than air. This would tend to make sound waves travel faster through iron than it does through air. But iron also has a much higher density than air, which would tend to make sound waves travel slower through it.
The velocity relation looks like: vsound in fluid = B ρ−−√ (2.1.1) (2.1.1) v s o u n d i n f l u i d = B ρ. Sound will also travel through a solid, but in that case the interactions of the particles are different than in a fluid, and the constant that takes the place of tension is a different one: Young's modulus. But the formula ...
Measuring waves. All sound waves are the same: they travel through a medium by making atoms or molecules shake back and forth. But all sound waves are different too. There are loud sounds and quiet sounds, high-pitched squeaks and low-pitched rumbles, and even two instruments playing exactly the same musical note will produce sound waves that are quite different.
It's true that sound travels fastest through solids, but solid objects actually block sound waves from reaching a given space. The reason behind this is very simple: you see, when sound originates from a point, travels through a medium, and then encounters a solid object, it loses some of its energy. In other words, a change in the medium ...
For example, a plucked guitar string vibrates, sending sound waves in all directions. Sound travels through any space with molecules in it - whether solid, liquid, or gas. It cannot travel in a vacuum ('In space, no one can hear you scream") since there are very few to no molecules. Sound waves contain energy, and so it can
However, if the solid is completely inelastic then the sound cannot travel through it. The practicality of this concept is highly applicable. The human ear captures sound waves through the outer cartilage of the ear, called the Pinna. The sound waves then travel up the ear canal and arrive at the ear drum which vibrates from the sound waves.
Students explore how sound waves move through liquids, solids and gases in a series of simple sound energy experiments. Understanding the properties of sound and how sound waves travel helps engineers determine the best room shape and construction materials when designing sound recording studios, classrooms, libraries, concert halls and theatres.
The time for safe listening decreases as sound levels increase.For example, a sound as high as 100 dB can be safely listened to for only 15 minutes each day. ... sound can travel through solids ...
Sound travels as waves of energy, but, unlike light, the waves transmit energy by changing the motion of particles. ... but the sound doesn't reach the microphone on the camera and the people until 25 s into the video. The sound took 13 s to travel through the air, one group of particles hitting another, to get to the air particles that were ...
The speed of sound in a material is determined mainly by two properties- the stiffness of the material and the density of the material. Sound travels fastest through materials that are stiff and light. In general, sound travels fastest through solids, slower through liquids and slowest through gasses. (See the table on this page).
Copper - 4,600 m/s. Aluminum - 5,120 m/s. Iron - 5,120 m/s. Glass - 5,640 m/s. Steel - 5,960 m/s. Diamond - 12,000 m/s. As you can see, the effect of these materials' varying properties on the speed of sound is quite pronounced—sound travels nearly 35 times faster through a diamond than it does through air. 6.
Travelling Through Solids. Sound waves are longitudinal. Sound waves are longitudinal waves, which means they vibrate in the same line as the wave direction. These waves can travel through solids. Sound travels at different speeds. Sounds can travel at different speeds in different substances. Sounds travel fastest in solids, and slowest in gases.
Sound - visualising sound waves. Sound is a form of energy that is caused by the vibration of matter. Sound is transmitted through waves, which travel through solids, liquids and gases. We are most used to the sound travelling through air, but sound is able to travel faster and further in solids and liquids.
Speed of Sound in Physics. This entry was posted on June 17, 2023 by Anne Helmenstine (updated on June 22, 2023) The speed of sound in dry air at room temperature is 343 m/s or 1125 ft/s. In physics, the speed of sound is the distance traveled per unit of time by a sound wave through a medium. It is highest for stiff solids and lowest for gases.
In non-humid air at 20 degrees Celsius, the speed of sound is about 343 meters per second or 767 miles per hour. We can also watch the speed of sound of a repeating simple harmonic wave. The speed of the wave can again be determined by the speed of the compressed regions as they travel through the medium.
1 Answer. Sound waves are longitudinal waves, they propagate though space from particles colliding with each other. Gases are less dense than liquids or solids, so when sound moves through them, the gas molecules bump into each other less frequently because they are more spread out. This causes the velocity of the sound wave to normally be ...
Sound waves traveling through a fluid such as air travel as longitudinal waves. Particles of the fluid (i.e., air) vibrate back and forth in the direction that the sound wave is moving. This back-and-forth longitudinal motion creates a pattern of compressions (high pressure regions) and rarefactions (low pressure regions).
Share it! When sound waves travel through a medium, the particles of the medium vibrate. Vibrations reach the ear and then the brain which senses them and we recognize sound. Read on for an explanation of how sound travels. Sound is a series of compression and rarefraction waves that can travel long distances. It is produced by the vibration of ...
Teacher Tip: Use examples where sound travels through media other than air. Thinking about the teaching. You can have an interesting discussion with pupils about sounds travelling through solids and liquids. Most of them will be able to tell you that they can still hear muffled sounds while swimming underwater in a swimming pool. They may have ...
A mechanical wave is a disturbance that moves and transports energy from one place to another through a medium. In sound, the disturbance is a vibrating object. And the medium can be any series of interconnected and interactive particles. This means that sound can travel through gases, liquids and solids. Let's take a look at an example.
Cover one ear with your hand and the other ear with the bag of air. Have an assisstant tap the bag with a pencil. How does it sound? Now fill the bag with water (a liquid) and seal it. Hold this water-filled bag against one ear while covering the other ear with your hand. Have your assistant tap this bag with a pencil.
Sound energy is produced when an object vibrates. The sound vibrations cause waves of pressure that travel through a medium, such as air, water, wood or meta...