- Correspondence
- Open access
- Published: 15 June 2023
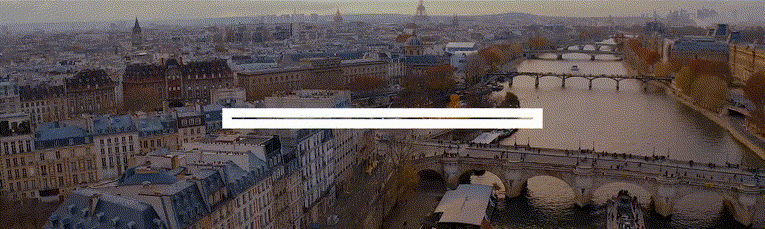
“Under pressure”: should we use diaphragm excursion to predict weaning success in patients receiving pressure support ventilation?
- Emma Sabourin 1 ,
- Christophe Carpentier 1 ,
- Christopher Lai 1 , 2 ,
- Xavier Monnet 1 , 2 &
- Tài Pham 1 , 3
Critical Care volume 27 , Article number: 238 ( 2023 ) Cite this article
1573 Accesses
3 Citations
Metrics details
The Original Article was published on 14 January 2023
We read with great interest the recent article published in Critical Care by Huan Ma et al. entitled “Using automatic speckle tracking imaging to measure diaphragm excursion and predict the outcome of mechanical ventilation weaning” [ 1 ]. Diaphragm ultrasound is an interesting technique to better understand weaning physiology and outcomes, and although we agree with the authors' perspective, we think that the results of the study should be interpreted with caution.
This prospective, multicenter, observational study aimed to evaluate the ability of diaphragm excursion (assessed with automatic speckle tracking) to predict weaning outcome. The authors found a significant correlation between the automatic measurement of mean excursion and velocity in speckle tracking imaging and its manual measurement. After analyzing the receiver operating characteristic (ROC) curve, they showed that diaphragmatic velocity and mean excursion were promising high diagnostic values for prolonged weaning. Yet, the diagnostic value of diaphragm excursion was moderate for predicting in-hospital death, withdrawal of treatment and weaning failure.
We would like to raise a few points that appear important when using ultrasound to evaluate diaphragm function and that might impact the interpretation of the study.
First of all, diaphragm excursion was measured in patients receiving invasive mechanical ventilation in pressure support mode with support set at 10–12 cm H 2 O. It appears to us that the value of assessing diaphragm excursion under assisted mechanical ventilation, such as in this study, should be subject to caution and cannot be interpreted as the patient’s own respiratory muscle strength.
Indeed, as well demonstrated in several studies [ 2 , 3 , 4 ], it is not possible to differentiate which part of the diaphragmatic excursion measured is due to the external force applied by the ventilator (passive), and which part of the excursion is due to the diaphragmatic contraction (active). Measures of diaphragm excursion under assisted mechanical ventilation will consequently be overestimated because the patient’s diaphragmatic contraction is added to the passive excursion generated by the ventilator in pressure support mode.
Conceptually, this would not allow for weaning outcome prediction, and in their study, Zombon et al. [ 2 ] emphasized the fact that diaphragm excursion measures should be limited to patients with spontaneous breathing, as it is not a marker per se of diaphragm contraction or respiratory effort but a marker of diaphragm movement is highly dependent on inspired volumes.
M. Llamas-Álvarez and co-workers [ 3 ] also highlighted the fact that diaphragm excursion is only relevant in patients without ventilator support, and showed that diaphragm excursion interpretation entails several biases as diaphragm excursion may vary depending on several parameters such as the patient’s positioning, and thoracic or abdominal pressure variation. These authors even concluded that diaphragm excursion should not be used to assess diaphragm function.
Using diaphragm excursion to predict weaning success should therefore be measured in patients undergoing a spontaneous breathing trial, such as a T-piece trial (disconnecting the patient from the ventilator) or a ZEEP trial (decreasing the pressure support to minimal values with PEEP set at 0 cm H 2 0). In this situation, the excursion measured will hence apprehend the diaphragmatic contraction without the impact of the pressure support generated by the ventilator.
Diaphragm thickening fraction, which is also measured with ultrasound, is another interesting technique as several studies demonstrated its reliability to predict extubation success [ 4 ]. It might even be superior to diaphragm excursion in this indication: two studies have demonstrated a significant correlation between the diaphragmatic tidal thickening fraction and the diaphragmatic pressure–time product in patients receiving noninvasive ventilation after extubation and in healthy subjects and intubated patients with pressure support ventilation [ 4 ].
Moreover, some studies have shown an interesting and feasible method for predicting weaning success using the measurement of the right diaphragm thickening fraction in combination with the rapid shallow breathing index (RSBI). This combination has been shown to improve the precision of successful weaning prediction when compared with RSBI alone [ 5 ].
Therefore, the prediction of weaning success in patients undergoing assisted breathing trials should be evaluated by diaphragmatic thickening fraction as it is less impacted by pressure support variation [ 2 ].
In a nutshell, we think diaphragm excursion measurement is an interesting approach but should be done in patients with spontaneous breathing without pressure support to be able to predict weaning success. Nonetheless, this study has shown promising results regarding the feasibility and reliability of speckle tracking imaging, with high correlation values. Further research on diaphragm function assessment to predict weaning outcome is needed.
Availability of data and materials
Not applicable.
Huang D, Song F, Luo B, Wang S, Qin T, Lin Z, et al. Using automatic speckle tracking imaging to measure diaphragm excursion and predict the outcome of mechanical ventilation weaning. Crit Care. 2023;27:18.
Article PubMed PubMed Central Google Scholar
Zambon M, Greco M, Bocchino S, Cabrini L, Beccaria PF, Zangrillo A. Assessment of diaphragmatic dysfunction in the critically ill patient with ultrasound: a systematic review. Intensive Care Med. 2017;43:29–38.
Article PubMed Google Scholar
Llamas-Álvarez AM, Tenza-Lozano EM, Latour-Pérez J. Diaphragm and lung ultrasound to predict weaning outcome: systematic review and meta-analysis. Chest. 2017;152:1140–50.
Sferrazza Papa GF, Pellegrino GM, Di Marco F, Imeri G, Brochard L, Goligher E, et al. A review of the ultrasound assessment of diaphragmatic function in clinical practice. Respiration. 2016;91:403–11.
Pirompanich P, Romsaiyut S. Use of diaphragm thickening fraction combined with rapid shallow breathing index for predicting success of weaning from mechanical ventilator in medical patients. J Intensive Care. 2018;6:6.
Download references
Author information
Authors and affiliations.
Service de Médecine Intensive-Réanimation, Hôpital de Bicêtre, DMU CORREVE, FHU SEPSIS, Groupe de Recherche CARMAS, Hôpitaux Universitaires Paris-Saclay, AP-HP, 94270, Le Kremlin-Bicêtre, France
Emma Sabourin, Christophe Carpentier, Christopher Lai, Xavier Monnet & Tài Pham
INSERM UMR S_999, Pulmonary Hypertension: Pathophysiology and Novel Therapies, University Paris-Saclay, Hôpital Marie Lannelongue, Le Plessis-Robinson, France
Christopher Lai & Xavier Monnet
INSERM U1018, Equipe d’Epidémiologie Respiratoire Intégrative, CESP, Université Paris-Saclay (UVSQ)—Université Paris-Sud, 94807, Villejuif, France
You can also search for this author in PubMed Google Scholar
Contributions
ES, CC and TP provided concept and design. ES, CC and TP performed drafting of the manuscript. ES, CC, CL, XM and TP performed critical revision of the manuscript for important intellectual content. All authors read and approved the final manuscript.
Corresponding author
Correspondence to Tài Pham .
Ethics declarations
Ethical approval and consent to participate, competing interests.
C.L. received honoraria for lectures from Sedana Medical. XM is a member of the medical advisory board of Pulsion Medical Systems. He received lecture fees from Pulsion Medical Systems and Baxter Healthcare. E.S., C.C and T.P have no competing interests to declare.
Additional information
Publisher's note.
Springer Nature remains neutral with regard to jurisdictional claims in published maps and institutional affiliations.
Rights and permissions
Open Access This article is licensed under a Creative Commons Attribution 4.0 International License, which permits use, sharing, adaptation, distribution and reproduction in any medium or format, as long as you give appropriate credit to the original author(s) and the source, provide a link to the Creative Commons licence, and indicate if changes were made. The images or other third party material in this article are included in the article's Creative Commons licence, unless indicated otherwise in a credit line to the material. If material is not included in the article's Creative Commons licence and your intended use is not permitted by statutory regulation or exceeds the permitted use, you will need to obtain permission directly from the copyright holder. To view a copy of this licence, visit http://creativecommons.org/licenses/by/4.0/ . The Creative Commons Public Domain Dedication waiver ( http://creativecommons.org/publicdomain/zero/1.0/ ) applies to the data made available in this article, unless otherwise stated in a credit line to the data.
Reprints and permissions
About this article
Cite this article.
Sabourin, E., Carpentier, C., Lai, C. et al. “Under pressure”: should we use diaphragm excursion to predict weaning success in patients receiving pressure support ventilation?. Crit Care 27 , 238 (2023). https://doi.org/10.1186/s13054-023-04504-8
Download citation
Received : 16 May 2023
Accepted : 23 May 2023
Published : 15 June 2023
DOI : https://doi.org/10.1186/s13054-023-04504-8
Share this article
Anyone you share the following link with will be able to read this content:
Sorry, a shareable link is not currently available for this article.
Provided by the Springer Nature SharedIt content-sharing initiative
Critical Care
ISSN: 1364-8535
- Submission enquiries: [email protected]
Learn how UpToDate can help you.
Select the option that best describes you
- Medical Professional
- Resident, Fellow, or Student
- Hospital or Institution
- Group Practice
- Patient or Caregiver
- Find in topic
RELATED TOPICS
INTRODUCTION
This topic will discuss the recognition and surgical management of blunt and penetrating injury to the diaphragm. Injuries to associated thoracic and abdominal organs are discussed in separate topic reviews. The general approach to blunt and penetrating chest and abdominal trauma is also discussed elsewhere. (See "Initial evaluation and management of blunt thoracic trauma in adults" and "Initial evaluation and management of penetrating thoracic trauma in adults" and "Initial evaluation and management of blunt abdominal trauma in adults" .)
ANATOMY OF THE DIAPHRAGM
The diaphragm is dome-shaped and attaches to the chest and abdominal walls circumferentially ( figure 1 ). The liver, spleen, transverse colon, stomach, pancreas, adrenal glands, and kidneys contact the undersurface of the diaphragm. Thoracoabdominal structures, including the aorta, inferior vena cava, thoracic duct, esophagus, vagus nerves, and phrenic nerves, traverse the diaphragm through three major apertures (ie, aortic, caval, esophageal) ( figure 2 ).
The diaphragm is composed of two muscle groups, costal and crural, which are compositionally and functionally distinct. Both groups are innervated by the phrenic nerves ( figure 3 and figure 4 ). The costal muscle group that forms the diaphragmatic leaflets is thin, and contraction of its fibers flattens the diaphragm and lowers the ribs. The crural muscle groups are thicker but contribute minimally to the displacement of the diaphragm. The median arcuate ligament anterior to the aortic hiatus is formed by the continuation of the medial tendinous margins of the crura.
- Research Articles
- Open access
- Published: 27 October 2023
Diaphragm excursions as proxy for tidal volume during spontaneous breathing in invasively ventilated ICU patients
- Matthijs L. Janssen 1 , 2 ,
- Annemijn H. Jonkman 1 ,
- Myrte Wennen 1 ,
- Evert-Jan Wils 1 , 2 ,
- Henrik Endeman 1 &
- Leo Heunks 1
Intensive Care Medicine Experimental volume 11 , Article number: 73 ( 2023 ) Cite this article
659 Accesses
11 Altmetric
Metrics details
There is a need to monitor tidal volume in critically ill patients with acute respiratory failure, given its relation with adverse clinical outcome. However, quantification of tidal volume in non-intubated patients is challenging. In this proof-of-concept study, we evaluated whether ultrasound measurements of diaphragm excursion could be a valid surrogate for tidal volume in patients with respiratory failure. Diaphragm excursions and tidal volumes were simultaneously measured in invasively ventilated patients ( N = 21) and healthy volunteers ( N = 20). Linear mixed models were used to estimate the ratio between tidal volume and diaphragm excursion. The tidal volume–diaphragm excursion ratio was 201 mL/cm in ICU patients [95% confidence interval (CI) 161–240 mL/cm], and 361 (294–428) mL/cm in healthy volunteers. An excellent association was shown within participants ( R 2 = 0.96 in ICU patients, R 2 = 0.90 in healthy volunteers). However, the differences between observed tidal volume and tidal volume as predicted by the linear mixed models were considerable: the 95% limits of agreement in Bland–Altman plots were ± 91 mL in ICU patients and ± 396 mL in healthy volunteers. Likewise, the variability in tidal volume estimation between participants was large. This study shows that diaphragm excursions measured with ultrasound correlate with tidal volume, yet quantification of absolute tidal volume from diaphragm excursion is unreliable.
High respiratory effort and tidal volume (TV) have been linked to aggravation of lung injury in patients with acute respiratory failure, also referred to as patient self-inflicted lung injury (P-SILI) [ 1 , 2 ]. Timely identification of patients with respiratory deterioration may be of clinical relevance [ 3 , 4 ]. The ROX-index (SpO 2 /FiO 2 /respiratory rate) has been validated to identify patients on high-flow nasal oxygen at risk for requiring endotracheal intubation [ 5 ]. Yet, upon increased respiratory loading, changes in TV precede increases in respiratory rate [ 6 ]. Indeed, replacing respiratory rate by TV in the ROX-index significantly improved predicting requirement of invasive mechanical ventilation in patients with respiratory failure [ 7 ]. However, TV measurement in non-intubated critically ill patients is challenging given the need for accurate airflow measurements.
Ultrasound assessment of diaphragm excursion is reproducible and fair correlations with TV were reported in non-clinical studies [ 8 , 9 , 10 ]. Therefore, we hypothesized that bedside measurement of diaphragm excursion could be a valid surrogate for TV. Studies evaluating diaphragm motion have been performed earlier in the context of weaning from mechanical ventilation [ 11 ]. The aim of the current proof-of-concept study was to determine the relationship between TV and diaphragm excursions in ICU patients and healthy volunteers. In addition, we investigated correlations between changes in TV and diaphragm excursion within patients.
In this prospective study two groups were studied: healthy volunteers and patients on invasive mechanical ventilation, enrolled between August and December 2022. The ethics board approved the study (MEC-2022-0451) and written informed consent was obtained, through legal representatives whenever necessary. Patients with tracheostomy, Body Mass Index (BMI) > 35 kg/m 2 , exacerbation of obstructive lung disease, large pleural effusions (> 1.5 cm), neuromuscular disease or diaphragm paralysis (defined as known paralysis in medical history or having paradoxal diaphragm movement on ultrasound) were excluded.
Simultaneous measurements of TV and diaphragm excursion were obtained in at least 3 breaths per participant. The right hemi-diaphragm was visualized in semi-recumbent position (30 degrees) using subcostal view in M-mode (Sparq, Philips; 2–4 MHz probe) by a single observer experienced in diaphragm ultrasound, as previously described[ 12 ]. Images were stored for offline analysis (Sante DICOM Viewer). In patients on invasive mechanical ventilation (Servo-U, Getinge, Sweden) measurements were performed during the first few minutes of a spontaneous breathing trial with positive end-expiratory pressure (PEEP) of 5 cmH 2 O and no inspiratory pressure support. Healthy volunteers were breathing through a mouthpiece with flow sensor connected to a signal acquisition system (BIOPAC Systems, USA), while wearing a nose clip to prevent air leakage. They were instructed to perform tidal breathing as well as deep breathing at non-maximal levels to generate a range of TV. To maximize precision of the measurements, both TV and diaphragm excursions were determined offline and thus were not read from the ventilator or ultrasound machine directly during the imaging procedure. Diaphragm excursions were determined by measurement of the amplitude of the M-line, while being blinded for the corresponding TV values. TV were extracted from the integral of the inspiratory flow-time curve as exported from the ventilator monitor in ICU patients or as measured with a dedicated transducer in healthy subjects.
Using intra-class correlation coefficient (ICC) analysis, single observer test–retest reliability was assessed for diaphragm excursion in a random sample of 3 healthy volunteers and 3 patients ( n = 74 breaths) using a two-way mixed model with single measures of agreement. Furthermore, we assessed the stability of the ratio between diaphragm excursion and TV within a subject as a surrogate of measurement reliability, considering that this ratio should not change within the short time interval. To this end, we used 3–5 breaths for all subjects and employed a two-way mixed model with single measures of consistency.
Statistical analysis was performed with R (RStudio, version 4.2.2). A linear mixed model with a random intercept per participant and fixed effect of diaphragm excursion was used to estimate the TV-diaphragm excursion ratio, thereby taking multiple and variable measurements per subject into account. The agreement between the observed TV and the TV as predicted by the linear mixed model was evaluated using a Bland–Altman plot. In addition, to test if the relationship between TV and diaphragm excursion was affected by any participant characteristics, such as age, BMI and chest circumference, these characteristics were individually added to the linear mixed model as fixed effects. For all analyses, a p value < 0.05 was considered statistically significant.
ICU patients ( N = 21) and healthy volunteers (N = 20) (Table 1 ) yielded 139 (median 6, IQR 5–8) and 255 (median 13, IQR 11–14) analyzable breaths, respectively. A good stability of the TV-diaphragm excursion ratio over consecutive breaths within one subject was shown (ICC: 0.86). The mean (± standard deviation) difference between first and second diaphragm excursion measurement was 0.035 ± 0.20 cm. The ICC for intra-observer variability between the first and second measurement of diaphragm excursion was 0.99. The models indicated an excellent association within participants ( R 2 = 0.96 in invasively ventilated patients, R 2 = 0.90 in healthy volunteers), as illustrated in Fig. 1 . The TV-diaphragm excursion ratio was 201 mL/cm in ICU patients (95% confidence interval (CI) 161–240 mL/cm), p < 0.001, and 361 (95% CI 294–428) mL/cm, p < 0.001 in healthy volunteers. The mean (± standard deviation) value of the per-patient intercept of the model was 0 ± 157 mL in ICU patients, and 0 ± 267 mL for healthy volunteers. The variability in TV estimation between participants was large: e.g., a diaphragm excursion of 1.5 cm could correspond to a TV between 250 and 750 mL in ICU patients (Fig. 1 ). Participant characteristics (age, sex, height, Ideal Body Weight, BMI, chest circumference and days on invasive mechanical ventilation) did not affect the relationship between diaphragm excursion and TV (Table 1 ). Bland–Altman plots (Fig. 2 ) showed considerable differences between observed and predicted TV (95% limits of agreement: ± 91 mL in ICU patients and ± 396 mL in healthy volunteers).
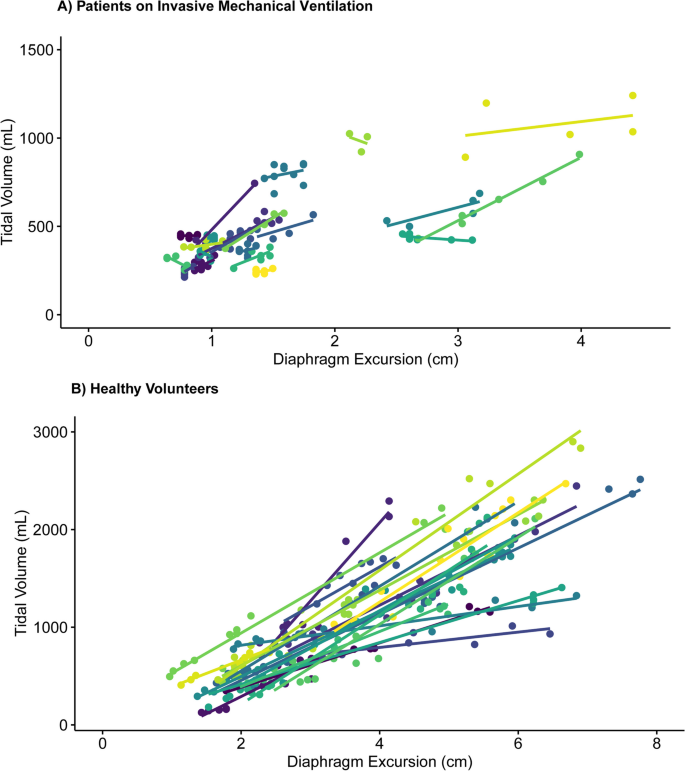
Correlation between Diaphragm Excursion and Tidal volume per participant, separated for ICU patients on invasive mechanical ventilation and healthy volunteers. Every color represents a different participant. A Patients on Invasive Mechanical Ventilation. B Healthy volunteers
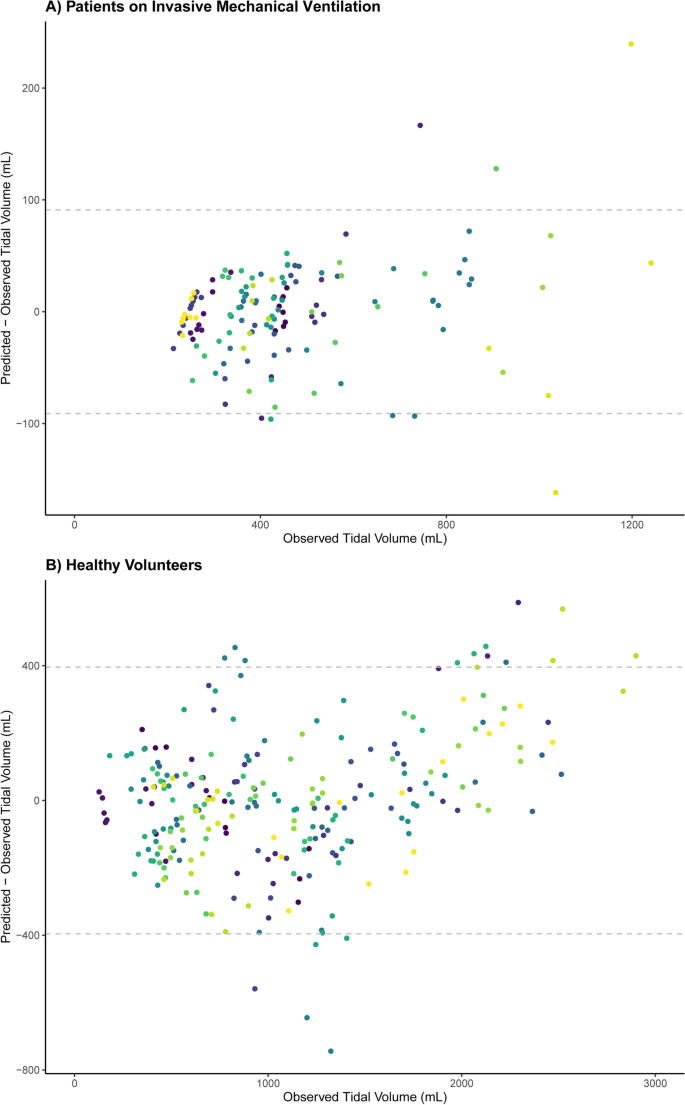
Bland–Altman plot showing the association between observed and predicted Tidal Volumes based on the linear mixed model, separated for ICU patients on invasive mechanical ventilation and healthy volunteers. Every color represents a different participant. The dashed lines indicate 95% limits of agreement: ± 91 mL in ICU patients ( A ) and ± 396 mL in healthy volunteers ( B )
Our study demonstrates a correlation between ultrasound measurement of diaphragm excursion and TV in healthy volunteers, but the large variability in the data in ICU patients indicates a less obvious association. This precludes a reliable estimation of the absolute value of TV from diaphragm excursion measurement in the clinical setting.
The difference in TV-diaphragm excursion ratio between ICU patients and healthy volunteers may be explained by a smaller distribution of TV in ICU patients. Indeed, an additional (sensitivity) analysis of the TV-diaphragm excursion relationship in healthy volunteers when including only breaths in the same TV range as ICU patients (TV ≤ 1250 mL) indicated that the TV-diaphragm excursion ratio is comparable to ICU patients [231 (209–255) mL/cm]. In addition, altered respiratory mechanics and potential effects of PEEP on diaphragm efficiency [ 13 ] have likely played a role. An earlier study showed that the application of PEEP resulted in caudal displacement of the diaphragm and decreased the diaphragm contractile efficiency. Possibly, the decrease in TV-diaphragm excursion ratio in ICU patients compared to healthy volunteers is explained by PEEP.
Our results contrast with earlier studies that observed a fair correlation between TV and diaphragm excursion [ 9 , 10 ]. However, these studies were performed in non-clinical settings, and used simple linear regression analysis without accounting for multiple measurements per participant. Furthermore, their larger TV-diaphragm excursion ratios (555 and 625 mL/cm, respectively) may be explained by recruitment of accessory muscles, since participants in earlier studies were instructed to inhale up to total lung capacity.
There are limitations of our study to acknowledge. First, we did not quantify accessory muscle use, although the association between diaphragm excursion and TV is affected by these muscles. Occult recruitment of accessory muscles may, therefore, have distorted the TV-diaphragm excursion ratio especially in ICU patients. However, we evaluated the potential of diaphragm excursion as bedside tool to monitor TV. Simultaneous evaluation of accessory muscle recruitment might have improved the understanding of the association between diaphragm excursion and TV, but would also complicate its clinical applicability. Second, the average time on invasive mechanical ventilation in the studied ICU patients was rather short. We recognize that the association between diaphragm excursion and TV may differ in patients with prolonged invasive mechanical ventilation due to diaphragm muscle dysfunction [ 14 ]. However, the targeted population to use diaphragm excursion as proxy for TV would concern non-intubated patients rather than those with prolonged invasive mechanical ventilation. Third, we excluded patients with high BMI due to difficulty of imaging the diaphragm, and also patients with exacerbation of obstructive lung disease due to flattening of their diaphragm resulting from pulmonary hyperinflation. This may affect the generalizability of our results as these are common comorbidities in the ICU population. Fourth, images from multiple breaths were obtained once in each participant. The use of a single ultrasonographer may imply that if this method were to be translated to clinical practice more variability from different observers may be introduced. However, the reproducibility of diaphragm excursion measurements via ultrasound has already been substantiated in a large study [ 8 ]. Consequently, we reasoned that imaging performed by multiple observers was deemed unnecessary in this study. Finally, TV was derived from the flow tracings but under different gas conditions (body temperature, pressure, water vapor saturated in ventilated patients and ambient temperature and pressure in healthy subjects); this will not affect the primary conclusion and between-subject variability but may result in a slightly higher absolute ratio (mL/cm) for healthy volunteers compared to ventilated patients.
Adequate diaphragm imaging is pivotal to establish the TV-diaphragm excursion ratio in the critical care setting. M-mode ultrasound measures unidimensional diaphragm movement and requires diaphragm motion perfectly aligned with the M-mode line. Even then, commonly employed one-dimensional measures of diaphragm excursion cannot capture the complete diaphragm motion, which is multidimensional. Our study emphasizes the complexity of the resultant relationship between a single measurement of diaphragm excursion and TV. Hence, a one-dimensional measure is unsuitable to determine absolute values or a safe cutoff for TV. Advanced techniques such as speckle tracking may have superior performance by quantifying diaphragm motion in multiple dimensions [ 15 , 16 ]. Prior studies were often hampered by the application of inspiratory pressure support during ultrasound measurements. However, it should be stressed that such measurements of excursion should be performed in patients without inspiratory ventilator support [ 12 , 17 ] to reliably reflect the patient’s own contribution to generating TV, such as done in our study. The relationship between diaphragm excursion as measured with speckle tracking and TV and its possible role in predicting the need for intubation in non-intubated patients with acute respiratory failure requires further study.
To conclude, in this proof-of-concept study in critically ill patients and healthy volunteers, single measurement of diaphragm excursion is not a clinically feasible surrogate for absolute values of TV. Consecutive measurements of diaphragm excursion may indicate changes in TV within patients with respiratory failure, yet its margin of error is too large to use the measurement for monitoring clinical deterioration. Therefore, diaphragm excursions measured with ultrasound should not be used to identify patients at risk for P-SILI.
Take home message
Monitoring tidal volume in patients with respiratory failure is necessary, but its measurement in non-intubated patients is challenging. This study shows that diaphragm excursions measured with ultrasound correlate with tidal volume, yet quantification of absolute values for tidal volume from diaphragm excursion is unreliable.
Measurements of diaphragm excursions with ultrasound correlate with tidal volume, but should not be used to determine tidal volume.
Availability of data and materials
The data sets used and/or analysed during the current study are available from the corresponding author on reasonable request.
Abbreviations
Intensive care unit
Invasive mechanical ventilation
Patient self-inflicted lung injury
Positive end-expiratory pressure
- Tidal volume
Body Mass Index
Ideal body weight
Grieco DL, Menga LS, Eleuteri D, Antonelli M (2019) Patient self-inflicted lung injury: implications for acute hypoxemic respiratory failure and ARDS patients on non-invasive support. Minerva Anestesiol 85(9):1014–1023
Article PubMed Google Scholar
Yoshida T, Grieco DL, Brochard L, Fujino Y (2020) Patient self-inflicted lung injury and positive end-expiratory pressure for safe spontaneous breathing. Curr Opin Crit Care 26(1):59–65
Brochard L, Slutsky A, Pesenti A (2017) Mechanical ventilation to minimize progression of lung injury in acute respiratory failure. Am J Respir Crit Care Med 195(4):438–442
Kang BJ, Koh Y, Lim CM, Huh JW, Baek S, Han M et al (2015) Failure of high-flow nasal cannula therapy may delay intubation and increase mortality. Intensive Care Med 41(4):623–632
Roca O, Caralt B, Messika J, Samper M, Sztrymf B, Hernandez G et al (2019) An index combining respiratory rate and oxygenation to predict outcome of nasal high-flow therapy. Am J Respir Crit Care Med 199(11):1368–1376
Vaporidi K, Akoumianaki E, Telias I, Goligher EC, Brochard L, Georgopoulos D (2020) Respiratory drive in critically ill patients. Pathophysiology and clinical implications. Am J Respir Crit Care Med 201(1):20–32
Chen D, Heunks L, Pan C, Xie J, Qiu H, Yang Y et al (2022) A novel index to predict the failure of high-flow nasal cannula in patients with acute hypoxemic respiratory failure: a pilot study. Am J Respir Crit Care Med. https://doi.org/10.1164/rccm.202203-0561LE
Article PubMed PubMed Central Google Scholar
Boussuges A, Gole Y, Blanc P (2009) Diaphragmatic motion studied by m-mode ultrasonography: methods, reproducibility, and normal values. Chest 135(2):391–400
Cohen E, Mier A, Heywood P, Murphy K, Boultbee J, Guz A (1994) Excursion-volume relation of the right hemidiaphragm measured by ultrasonography and respiratory airflow measurements. Thorax 49(9):885–889
Article CAS PubMed PubMed Central Google Scholar
Houston JG, Angus RM, Cowan MD, McMillan NC, Thomson NC (1994) Ultrasound assessment of normal hemidiaphragmatic movement: relation to inspiratory volume. Thorax 49(5):500–503
Parada-Gereda HM, Tibaduiza AL, Rico-Mendoza A, Molano-Franco D, Nieto VH, Arias-Ortiz WA et al (2023) Effectiveness of diaphragmatic ultrasound as a predictor of successful weaning from mechanical ventilation: a systematic review and meta-analysis. Crit Care (London, England) 27(1):174
Article Google Scholar
Tuinman PR, Jonkman AH, Dres M, Shi ZH, Goligher EC, Goffi A, et al. Respiratory muscle ultrasonography: methodology, basic and advanced principles and clinical applications in ICU and ED patients-a narrative review. Intensive Care Med. 2020;46(4):594–605.
Jansen D, Jonkman AH, Vries HJ, Wennen M, Elshof J, Hoofs MA et al (2021) Positive end-expiratory pressure affects geometry and function of the human diaphragm. J Appl Physiol (1985) 131(4):1328–1339
Article CAS PubMed Google Scholar
Vassilakopoulos T, Petrof BJ (2004) Ventilator-induced diaphragmatic dysfunction. Am J Respir Crit Care Med 169(3):336–341
Oppersma E, Hatam N, Doorduin J, van der Hoeven JG, Marx G, Goetzenich A et al (2017) Functional assessment of the diaphragm by speckle tracking ultrasound during inspiratory loading. J Appl Physiol (1985) 123(5):1063–1070
Huang D, Song F, Luo B, Wang S, Qin T, Lin Z et al (2023) Using automatic speckle tracking imaging to measure diaphragm excursion and predict the outcome of mechanical ventilation weaning. Critical Care (London, England) 27(1):18
Sabourin E, Carpentier C, Lai C, Monnet X, Pham T (2023) “Under pressure”: should we use diaphragm excursion to predict weaning success in patients receiving pressure support ventilation? Critical Care (London, England) 27(1):238
World Medical Association Declaration of Helsinki (2013) ethical principles for medical research involving human subjects. JAMA 310(20):2191–2194
Download references
Acknowledgements
We thank all study participants for making the study possible.
This study was partly funded by an unrestricted grant by Fisher & Paykel Healthcare (Auckland, New Zealand).
Author information
Authors and affiliations.
Department of Intensive Care, Erasmus Medical Center, Rotterdam, The Netherlands
Matthijs L. Janssen, Annemijn H. Jonkman, Myrte Wennen, Evert-Jan Wils, Henrik Endeman & Leo Heunks
Department of Intensive Care, Franciscus Gasthuis & Vlietland, Rotterdam, The Netherlands
Matthijs L. Janssen & Evert-Jan Wils
You can also search for this author in PubMed Google Scholar
Contributions
MLJ: made substantial contributions to the conception and design of the work, the acquisition, analysis, and interpretation of data; and drafted the work. AHJ: made substantial contributions to the conception and design of the work, analysis, and interpretation of data; and drafted the work. MW: made substantial contributions to the analysis and interpretation of data and substantively revised the manuscript. HE and EJW: made substantial contributions the conception and design of the work, interpretation of data and substantively revised the manuscript. LH: made substantial contributions to the conception and design of the work, interpretation of data, substantively revised the manuscript.
Corresponding author
Correspondence to Leo Heunks .
Ethics declarations
Ethics approval and consent to participate.
The ethics board approved the study (MEC-2022-0451) and informed consent was obtained. The study has been carried out in accordance with the Helsinki declaration for medical research involving humans [ 18 ].
Consent for publication
Not applicable.
Competing interests
AHJ has received personal fees from Liberate Medical (Crestwood, Kentucky). HE has received unrestricted research grants from Fisher & Paykel Healthcare (Auckland, New Zealand), La Roche Ltd. (Bazel, Switzerland) and Ventinova Medical B.V. (Eindhoven, the Netherlands). LH has received speakers fee from Getinge (Sweden), research support from Liberate Medical (Crestwood, Kentucky), ZonMw (Netherlands), and the European Respiratory Society, and personal fees from American Thoracic Society.
Additional information
Publisher's note.
Springer Nature remains neutral with regard to jurisdictional claims in published maps and institutional affiliations.
Rights and permissions
Open Access This article is licensed under a Creative Commons Attribution 4.0 International License, which permits use, sharing, adaptation, distribution and reproduction in any medium or format, as long as you give appropriate credit to the original author(s) and the source, provide a link to the Creative Commons licence, and indicate if changes were made. The images or other third party material in this article are included in the article's Creative Commons licence, unless indicated otherwise in a credit line to the material. If material is not included in the article's Creative Commons licence and your intended use is not permitted by statutory regulation or exceeds the permitted use, you will need to obtain permission directly from the copyright holder. To view a copy of this licence, visit http://creativecommons.org/licenses/by/4.0/ .
Reprints and permissions
About this article
Cite this article.
Janssen, M.L., Jonkman, A.H., Wennen, M. et al. Diaphragm excursions as proxy for tidal volume during spontaneous breathing in invasively ventilated ICU patients. ICMx 11 , 73 (2023). https://doi.org/10.1186/s40635-023-00553-z
Download citation
Received : 15 August 2023
Accepted : 22 September 2023
Published : 27 October 2023
DOI : https://doi.org/10.1186/s40635-023-00553-z
Share this article
Anyone you share the following link with will be able to read this content:
Sorry, a shareable link is not currently available for this article.
Provided by the Springer Nature SharedIt content-sharing initiative
- Respiratory failure
- Diaphragm excursion
- Non-invasive respiratory support
- High-flow nasal oxygen
- Research article
- Open access
- Published: 27 January 2023
Clinical values of diaphragmatic movement in patients with chronic obstructive pulmonary disease
- Taehwa Kim 1 , 2 na1 ,
- Sungchul Huh 3 na1 ,
- Jae Heun Chung 1 , 2 ,
- Yun Seong Kim 1 , 2 ,
- Ra Yu Yun 3 , 4 ,
- Onyu Park 5 &
- Seung Eun Lee ORCID: orcid.org/0000-0002-4266-7722 1 , 2
BMC Pulmonary Medicine volume 23 , Article number: 33 ( 2023 ) Cite this article
2018 Accesses
1 Altmetric
Metrics details
The limitation of activity due to dyspnea in chronic obstructive pulmonary disease (COPD) patients is affected by diaphragmatic dysfunction and reduced lung function. This study aimed to analyze the association between diaphragm function variables and forced expiratory volume in the first second (FEV1) and to estimate the clinical significance of diaphragm function in the correlation between COPD severity and lung function.
This prospective, single-center, cross-sectional observational study enrolled 60 COPD patients in a respiratory outpatient clinic. Data for baseline characteristics and the dyspnea scale were collected. Participants underwent a pulmonary function test (PFT), a 6-minute walk test (6MWT), and diaphragm function by ultrasonography.
The right excursion at forced breathing showed the most significant correlation with FEV1 ( r = 0.370, p = 0.004). The cutoff value was 6.7 cm of the right diaphragmatic excursion at forced breathing to identify the FEV1 above 50% group. In the group with a right diaphragmatic excursion at forced breathing < 6.7 cm, modified Medical Research Council (mMRC), St. George's Respiratory Questionnaire and the total distance of 6MWT showed no difference between groups with FEV1 under and above 50% ( p > 0.05). In the group with ≥ 6.7 cm, mMRC and the total distance of 6MWT showed a significant difference between FEV1 under and above 50% ( p = 0.014, 456.7 ± 69.7 m vs. 513.9 ± 60.3 m, p = 0.018, respectively).
The right diaphragmatic forced excursion was closely related to FEV1, and analysis according to the right diaphragmatic forced excursion-based cut-off value showed a significant difference between both groups. When the diaphragm function was maintained, there was a lot of difference in the 6MWT’s factors according to the FEV1 value. Our data suggest that diaphragmatic function should be performed when interpreting PFT.
Peer Review reports
Introduction
The most common complaint in respiratory diseases regardless of the disease type is dyspnea [ 1 ]. COPD is characterized by worsening dyspnea during movement [ 2 ]. COPD restricts various activities of daily living due to shortness of breath, leading to poor quality of life and increased mortality and morbidity [ 3 ]. There are many causes of dyspnea; however, for patients with stable COPD, a major contributor is the weakening of the respiratory muscles, excluding conditions such as acute infectious diseases [ 4 ].
The diaphragm is the main respiratory muscle, particularly the inspiratory muscles. The weakness of the diaphragm in COPD has been extensively studied. Some studies have reported a significant reduction in diaphragmatic excursion in patients with COPD [ 5 , 6 , – 7 ]. Lung hyperinflation-associated shortening of the diaphragm has traditionally been considered a major cause of diaphragmatic weakness [ 8 ]. Also, there were previous studies about diaphragmatic thickness. Diaphragmatic thickness was a factor related to weaning and prognosis in patients under mechanical ventilation [ 9 , 10 ]. Recently, several studies have reported the clinical value of diaphragm ultrasonography according to COPD severity, and even compared to traditional methods, the diagnostic value of ultrasonography has proven to be reliable and useful [ 11 ]. Ultrasonography is also commonly used in medical facilities because it can be carried out anywhere, has no associated radiation risk, and can be used to adequately visualize the structure of the diaphragm [ 12 ].
Furthermore, 6MWT is an important tool for assessing exercise capacity and functional status in patients with COPD. Diaphragmatic weakness can impair physical performance, especially the 6MWT [ 13 , 14 ]. A previous study reported that pulmonary function was significantly correlated with the 6MWT in patients with severe and very severe COPD [ 15 ]. The relationship between 6MWT and PFT is a matter of connecting and understanding the respiratory muscles. PFT is used to measure the volume and flow rate of the lungs, and 6MWT is an important test for evaluating the exercise capacity and functional status of patients.
When we summarize the above, PFT correlates with 6MWT in COPD patients [ 15 ]. 6MWT can evaluate physical performance of COPD patients. Physical performance can also reflect diaphragmatic weakness [ 13 , 14 ]. Therefore, PFT correlates with 6MWT, 6MWT reflects physical performance, and physical performance was associated with diaphragmatic weakness. This relationship of PFT and diaphragmatic weakness can be expressed as follows for the patient. If the pulmonary function expressed by PFT is good, or if case which the power and strength of the respiratory muscles are good when PFT remains the same, breathing is more stable. Therefore, understanding the physiological principles of the respiratory muscle performance that establish the relationship these and compensate for this is important for managing the patient’s condition. Through this study, a review of the correlation between the PFT reflecting the 6MWT and diaphragm ultrasound features of respiratory muscle may be helpful to understand the physiological principles of patients with COPD.
Thus, this study aimed to analyze diaphragm movement characteristics using ultrasonography in patients with COPD and clarify its association with pulmonary function.
Study design and methods
Study design and participants.
This single-center, prospective, cross-sectional observational study recruited participants from a tertiary hospital outpatient respiratory clinic between April 2020 and April 2021. The inclusion criteria were: 1) patients 18 years old or older diagnosed with COPD by a pulmonologist; COPD diagnostic criterion was a post-bronchodilator FEV1/forced vital capacity (FVC) ratio < 0.70 based on the Global Initiative for Chronic Obstructive Lung Disease (GOLD), 2) patients who could maintain the required posture for diaphragm function measurement by ultrasonography and stable breathing during the examination such as 6MWT. Patients unable to cooperate with the examination and unstable patients requiring immediate medical intervention were excluded. Patients with interstitial lung disease featured on chest computed tomography (CT) that could affect diaphragm movement were also excluded.
Sixty-nine patients were enrolled, six of whom with combined interstitial lung disease on CT were excluded. Two patients were lost to follow-up, and one died before all examinations were completed. Finally, 60 patients completed all examinations for the study protocol and were included in the analysis.
All patients provided informed consent before participating in the study. Each patient’s clinical information was collected from four domains: pulmonary function, exercise capacity, body composition, and diaphragm function. Pulmonary function was evaluated through spirometry, MIP, and maximal expiratory pressure (MEP). Exercise capacity and body composition were assessed using the 6MWT and bioelectrical impedance analysis (BIA). Diaphragm dysfunction is defined as loss of muscle contractility [ 16 ]. To evaluated diaphragm dysfunction, we was assessed using ultrasonography in both the M-mode and B-mode for excursion and thickness, respectively.
Assessments
For patients who had performed a PFT within 1 month of participating in the study, the previous results were used and no retesting was performed. Patients who had no available PFT results within 1 month of participating in this study were reevaluated after enrollment. The Carefusion Vmax 20 (VIASYS Healthcare Inc. Sensormedics; Yorba Linda, CA, USA) was used for PFTs and FEV1, FVC, diffusing capacity of the lungs for CO, and total lung capacity were measured using the body plethysmography test. Regarding spirometry, the patients sat in a small booth and breathed into a mouthpiece. One technical expert from the Department of Respiratory Medicine conducted all the tests to maintain the consistency of the results.
MIP (PONY FX, COSMED Inc.; Rome, Italy) and MEP (PONY FX, COSMED Inc.; Rome, Italy) were measured in the sitting position using a portable mouth pressure meter. Three consecutive MIP and MEP measurements were taken, and the best result was recorded. The PFT was measured in a sitting position. A flanged mouthpiece was applied to the short and rigid tube of the measuring instrument and air leakage was checked around the mouthpiece before testing. The test was performed by an experienced examiner who has conducted the test for more than 8 years. MIP was measured by exhaling as deep as possible and inhaling as hard as possible for at least 1.5 s. MEP was measured by inhaling as deep as possible and exhaling as hard as possible for at least 1.5 s. Both measurements were made three times, and patients recovered to normal breathing patterns with at least a minute of break between measurements. The highest of the three measurements was recorded [ 17 ].
The 6MWT was performed according to the American Thoracic Society standards under the direction of a well-trained respiratory therapist at a 30 m indoor walking course [ 18 ]. Patients were encouraged by the instructor every minute and were allowed to rest or quit the test at any point. We measured the total distance and peripheral saturation with the portable oxygen meter. The patients’ body compositions were estimated indirectly using the BIA from a supine position (InBody S10, InBody, Co. Ltd., Seoul, Korea).
Diaphragm function was assessed using ultrasonography (LOGIQ E9, GE Healthcare; Chicago, IL, USA) obtained from both supine and sitting positions. It is generally accepted that there are positional differences in diaphragm contractility. The effects of gravitational loading on the diaphragm length-tension and body position-mediated changes in intra-abdominal pressure may explain the differences found. Not only that there is also a difference in the excursion between right and left. The excursion of the right diaphragm shows a lower value than that of the left diaphragm because the liver in the abdominal cavity restricts the movement of the right diaphragm. We also measured the diaphragm function in two positions based on this information. The supine position involved lying on the back or with the face upward while the sitting position was semi-seated (45–60 degrees). Both M-mode and B-mode imaging were used to evaluate diaphragmatic excursion and thickness, respectively. The mid-clavicular line and the liver were used as anatomical landmarks on the right side and the spleen on the left side to visualize the diaphragm in the M-mode. B-mode ultrasonography was used to measure the diaphragmatic thickness at the bilateral zone of apposition [ 19 ]. The diaphragm thickness was measured during quiet spontaneous breathing without peak inspiratory or expiratory maneuvers. The diaphragmatic thickness fraction was calculated as the difference between thickness at the end of inspiration and thickness at the end of expiration divided by thickness at the end of expiration x 100. The diaphragmatic excursion was measured as follows. The highest position of the diaphragm movement taken by the M-mode was considered to be the end-expiratory phase, whereas the lowest position was considered as the end-inspiratory phase.
The dyspnea scale used St. George's Respiratory Questionnaire (SGRQ) and the modified Medical Research Council scale (mMRC scale). The SGRQ is a self-administered questionnaire with 76 items [ 20 ]. This can identify the patient’s symptoms and the activities of daily life. mMRC scale is most commonly used in the assessment of dyspnea in chronic respiratory diseases and is a very useful and unrecognized dyspnea scale [ 21 ].
Statistical analysis
The data were analyzed using IBM SPSS (version 27.0; Chicago, IL, USA). The level of significance was set at p < 0.05. Descriptive statistics, including numbers, percentages, means, and standard deviations, were used to summarize each variable (demographics, PFTs, 6MWT, and diaphragmatic ultrasound results). The results were analyzed by independent t-test, cross-analysis, and frequency analysis. The correlation between the variables was analyzed by Pearson’s Correlation Coefficient, which confirmed the linear relationship between two variables using a scatterplot. The cut-off value was calculated using the receiver operating characteristic (ROC) curve analysis. The reference plane was 0.5 or more in the ROC curve, and the p -value < 0.05; hence, this result was adopted. Consequently, the cut-off value was confirmed when sensitivity and specificity were plotted in a line chart, which is the point where the two graphs meet.
Ethics statement
We certify that all applicable institutional and governmental regulations concerning the ethical use of human volunteers were followed throughout this study. The study procedures were reviewed and approved by our Pusan National University Yangsan Hospital Institutional Review Board [IRB No. 05–2020-217].
FEV1 and diaphragm function
We assessed whether diaphragm function was associated with FEV1 (Fig. 1 ). In the total group analysis, both diaphragmatic excursion and thickness were associated with FEV1. However, the diaphragmatic excursion was more associated with FEV1 than thickness. Diaphragmatic excursion during forced breathing and in the supine position had a greater association with FEV1 than breathing at rest and in the sitting position. Additionally, when comparing the right and left under the same conditions, the right was more significant during forced breathing and in the supine position ( r = 0.370, p = 0.004,). Moreover, diaphragmatic thickness at right end-expiration was associated with FEV1. In summary, right ( r = 0.370, p = 0.004) and left ( r = 0.257, p = 0.048) diaphragmatic excursion during forced breathing in the supine position and diaphragmatic thickness at right end-expiration ( r = 0.310, p = 0.016) were significantly associated with FEV1.
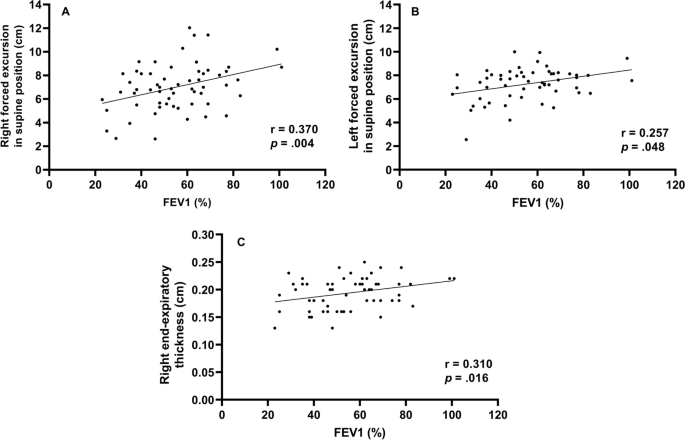
Correlation between forced expiratory volume in 1 s and diaphragm function Right forced excursion, and left forced excursion in the supine position and right end-expiratory thickness were correlated to forced expiratory volume in 1 s
Diaphragmatic function and BMI (body mass index)
To evaluate the function of the diaphragm muscle [ 22 ], the diaphragmatic excursion was measured at rest and during forced expiration (Supplement Table 1 ). In 60 patients, diaphragmatic excursion at rest in the supine position was 3.5 cm ± 1.2 on the right side and 3.5 cm ± 1.2 on the left side. During forced breathing, diaphragmatic excursion in the supine position was 6.9 cm ± 2.0 on the right side and 7.6 cm ± 1.6 on the left side. The total percent body fat was 24.2% ± 6.9. Segmental lean mass analysis was performed by direct segmental multi-frequency BIA. The lean mass was 90.5% ± 9.7 on the right arm, 88.1% ± 9.2 on the left arm, 94.5% ± 5.8 on the trunk, 95.7% ± 131.3 on the right leg, and 9.51% ± 8.8 on the left leg.
Cutoff value-associated characteristics
The ROC curve analysis of the diaphragm function variables was performed to identify the cutoff value for differentiating between FEV1 ≥ 50% and < groups. The cutoff value was ≤ 6.7 cm on the right diaphragmatic excursion at forced breathing with an area under the curve of 0.5 or more and p -value was 0.043. Right diaphragmatic excursion during forced breathing was less than the cut-off value of 6.7 cm for 26 patients and ≥ 6.7 cm for 43 patients (Table 1 ). There were no differences in age, sex, or smoking history between the two groups. The dyspnea scales such as mMRC, SGRQ, and GOLD were not significantly different between both groups. There were no differences in body mass index, percent body fat, or lean mass of the right or left legs between the groups. However, among the pulmonary function indicators, there were significant differences between the two groups. Specifically, FEV1, FVC, and MIP were significantly different (< 6.7 cm group vs. ≥ 6.7 cm group, FEV1: 49.2% ± 16.2 vs. 59.5% ± 17.2, p = 0.021; FVC: 76.2% ± 19.1 vs. 86.0% ± 15.5, p = 0.032; MIP: 67.4 cm H 2 O ± 25.1 vs. 86.5 cm H 2 O ± 28.7, p = 0.010). Concerning the 6MWT, there was a significant difference in SpO2 before 6MWT and the number of interruptions (SpO2 before 6MWT: 94.1% ± 2.7 vs. 95.3% ± 1.6, p = 0.038; number of interruptions: 4 [15.4%] vs. 0 [0%], p = 0.018). The left diaphragmatic excursion during forced breathing was also different between the two groups (6.8 cm ± 1.5 vs. 7.6 cm ± 1.3, p = 0.022) as well as the diaphragmatic thickness during right end-inspiration (0.3 cm ± 0.1 vs. 0.4 cm ± 0.1, p = 0.006). In addition, the ROC ≥ 6.7 cm group left diaphragmatic excursion was also measured with a value greater than that of the ROC < 6.7 cm group.
Subgroup characteristics according to FEV1
To identify the clinical significance of diaphragm function with the relationship between lung function, and COPD severity, the two groups classified as a right diaphragmatic excursion at 6.7 cm of forced breathing were further divided into groups based on FEV1 (< 50% or ≥ 50%) (Table 2 ). There were significant differences in age (65.0 ± 7.8 years vs. 72.7 ± 6.2 years, p = 0.011), the GOLD score ( p < 0.001), FEV1/FVC (40.1% ± 14.7 vs. 55.%4 ± 11.4, p = 0.007), peak expiratory flow rate (183.3 L/min ± 80.4 vs. 275.8 L/min ± 113.8, p = 0.027), SpO2 after the 6MWT (85.9% ± 6.5 vs. 91.5% ± 2.2, p = 0.011), and left diaphragmatic excursion during forced breathing (6.2 cm ± 1.6 vs. 7.4 cm ± 1.0, p = 0.038).
When the group with the right diaphragmatic excursion ≥ 6.7 cm was further divided into subgroups according to FEV1 (< 50% or ≥ 50%) and analyzed, mMRC, GOLD score, FEV1/FVC, MIP, peak expiratory flow rate, 6MWT, SpO2 before and after the 6MWT, and right diaphragmatic thickness at end-expiration subgroups were significantly different between the two groups.
This study contains the following: 1) evidence that FEV1 is significantly correlated with diaphragm movement, 2) cutoff values for diaphragm movement in patients with COPD, and 3) evidence to support the claim that the function of the diaphragm should be considered when interpreting the patient’s condition based on their FEV1.
First, FEV1 was significantly correlated with diaphragm movement. Studies on the relationship between the diaphragm and pulmonary function in patients with COPD are ongoing and have consistently reported that the severity of COPD and diaphragm function are closely related. Some previous studies have evaluated the direct relationship between FEV1 and diaphragm function [ 23 , 24 ].
The results of this study is also consistent with those of previous studies showing that diaphragm movement and FEV1 are related. However, beyond the findings of previous results [ 23 ], in our study, diaphragmatic excursion and thickness were found to be more correlated to FEV1 on the right side than on the left side.
Like the previous study that the thickness of the diaphragm is related to the ventilator weaning mechanical ventilation [ 9 , 10 ], this result has confirmed that the right diaphragm thickness was significantly related not only to the weaning of the ventilator and the prognosis of the patient but also to FEV1.
Second, we provided a cutoff value for a right diaphragmatic forced excursion in patients with COPD. Although there are studies that have presented a reference [ 23 ] value for healthy persons, the significant contribution of this study is the proposed reference value for patients with COPD.
We analyzed the correlation using Pearson’s correlation coefficient and confirmed the factors of diaphragmatic function-related components side (right, left), thickness, and excursion that were most-related to FEV1. Among them, Rt. forced excursion (supine), Lt. forced excursion (supine) and Rt. end-expiratory thickness showed meaningful p -value in association with FEV1. In addition, these three factors were analyzed in the linear relationship with the scattered plot and showed a proportional relationship between FEV1. Finally, when all factors related to the diaphragmatic function were analyzed, the right forced excursion was statistically determined as the most meaningful factor in relation to FEV1. We also obtained the cut-off value of 6.7 cm through the ROC curve.
The range in diaphragmatic excursion values varies considerably depending on the patient’s condition. A previous study has suggested normal values based on sex and the side of the diaphragm using healthy individuals. When breathing deeply, the right diaphragmatic excursion was 7 cm ± 1.1 in men and 5.7 cm ± 1 in women ( p < 0.001) and the left diaphragmatic excursion were 7.5 cm ± 0.9 and 6.4 cm ± 1 in men and women, respectively ( p < 0.01) [ 23 ]. In our study, we also assessed excursion during deep breathing to provide a cut-off value for patients with COPD.
When analyzed by dividing them into two groups based on a cut-off value, the following evaluation factors showed significant differences ( p < 0.05): FEV1, FVC, MIP, left forced excursion, right diaphragmatic thickness during end-inspiration, 6MWT, the SpO2 before and after 6MWT, and interruption of the 6MWT.
These factors can be broadly divided into PFT-related and performance-related factors. As mentioned above, PFT-related factors such as MIP, left diaphragmatic forced excursion and right diaphragmatic thickness during end-inspiration were lower in the < 6.7 cm group. Moreover, the SpO2 level before the 6MWT was lower in the < 6.7 cm group, the overall 6MWT was shorter, and there were many interruptions in the 6MWT. These factors might reflect activity as a performance evaluation factor. Although generalizability is limited given the few patients and the fact that all the participants were outpatients who could walk; these results may reflect an actual patient’s status. However, these findings are intended for patients who can walk, suggesting that the cut-off value of 6.7 cm may be reliable in this population.
Finally, results concerning the degree of pulmonary function and correlations with the diaphragmatic movement were noteworthy. The two groups were analyzed based on the right diaphragmatic forced excursion (6.7 cm) and divided into subgroups based on FEV1 (< 50% vs. ≥ 50%). As a result, in the group that had maintained diaphragm function (≥ 6.7 cm), the MIP, portable peak flow meter, 6MWT, SpO2 before and after the 6MWT, and right diaphragmatic thickness at end-expiration were different between the two FEV1 groups. In summary, the difference between the two FEV1 groups was large when diaphragm function was maintained; when it was not maintained, there were no differences between the two FEV1 groups. Therefore, even in patients who maintained their FEV1 > 50%, when diaphragm function deteriorated, the patient’s 6MWT, SpO2 before and after the 6MWT were less predictable (they either deteriorated or were maintained). The patients whose FEV1 decreased < 50%, if the diaphragm function was maintained, the 6MWT could be better than that in patients with an FEV1 ≥ 50% and a reduced diaphragm function.
In conclusion, when interpreting a patient’s condition based on FEV1, it is important to assess diaphragm function, since the effect of the FEV1 value on the patient depends on how well the diaphragm function has been maintained.
In this study, when the diaphragm function was maintained, there were significant differences in MIP, peak expiratory flow rate, 6MWT, SpO2 before and after the 6MWT, and right diaphragmatic thickness at end-expiration according to FEV1 in patients with COPD. Even if the diaphragm function was not maintained, because there are still differences in the FEV1, it may be beneficial to consider diaphragmatic function measured by right diaphragm excursion as an additional indicator of function beyond the FEV1. Therefore, it can be clinically helpful to check whether the diaphragm is functioning properly when determining a patient’s condition based on FEV1.
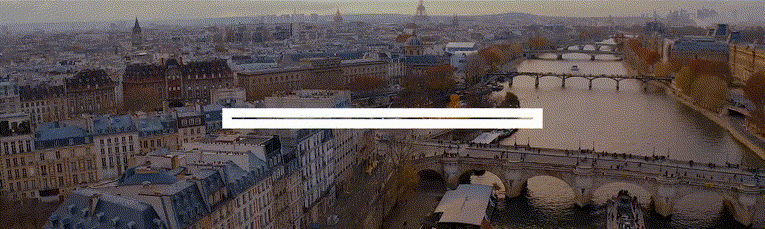
Availability of data and materials
The datasets used and/or analysed during the current study are available from the corresponding author on reasonable request.
Abbreviations
Chronic obstructive pulmonary disease
Pulmonary function test
- 6-minute walk test
Forced expiratory volume in the first second
Maximal inspiratory pressure
International Classification of Diseases 11TH
Forced vital capacity
Global Initiative for Chronic Obstructive Lung Disease
Computed tomography
Maximal expiratory pressure
Bioelectrical impedance analysis
Modified Medical Research Council
Receiver operating characteristic
Body mass index
St. George's Respiratory Questionnaire
Niedermeyer J. Dyspnea in airway and pulmonary diseases. Internist. 2015;56(8):882–9.
Article CAS Google Scholar
Antoniu SA. Descriptors of dyspnea in obstructive lung diseases. Multidisciplinary respiratory medicine. 2010;5(3):216–9.
Article Google Scholar
Lopez AD, Shibuya K, Rao C, Mathers CD, Hansell AL, Held LS, et al. Chronic obstructive pulmonary disease: current burden and future projections. Eur Respir J. 2006;27(2):397–412.
Decramer M. Respiratory muscles in COPD: regulation of trophical status Verhandelingen. Koninklijke Academie voor Geneeskunde van Belgie. 2001;63(6):577–602 discussion −4.
CAS Google Scholar
Corbellini C, Boussuges A, Villafañe JH, Zocchi L. Diaphragmatic mobility loss in subjects with moderate to very severe COPD may improve after in-patient pulmonary rehabilitation. Respir Care. 2018;63(10):1271–80.
Crimi C, Heffler E, Augelletti T, Campisi R, Noto A, Vancheri C, et al. Utility of ultrasound assessment of diaphragmatic function before and after pulmonary rehabilitation in COPD patients. Int J Chronic Obstruct Pulmon Dis. 2018;13:3131–9.
He L, Zhang W, Zhang J, Cao L, Gong L, Ma J, et al. Diaphragmatic motion studied by M-mode ultrasonography in combined pulmonary fibrosis and emphysema. Lung. 2014;192(4):553–61.
Laghi F, Tobin MJ. Disorders of the respiratory muscles. Am J Respir Crit Care Med. 2003;168(1):10–48.
Goligher EC, Fan E, Herridge MS, Murray A, Vorona S, Brace D, et al. Evolution of diaphragm thickness during mechanical ventilation. Impact Inspiratory Effort Am J Respirat Cri Care Med. 2015;192(9):1080–8.
Goligher EC, Dres M, Fan E, Rubenfeld GD, Scales DC, Herridge MS, et al. Mechanical ventilation-induced diaphragm atrophy strongly impacts clinical outcomes. Am J Respir Crit Care Med. 2018;197(2):204–13.
Boussuges A, Rives S, Finance J, Brégeon F. Assessment of diaphragmatic function by ultrasonography: current approach and perspectives. World J Clin Cases. 2020;8(12):2408–24.
Sarwal A, Walker FO, Cartwright MS. Neuromuscular ultrasound for evaluation of the diaphragm. Muscle Nerve. 2013;47(3):319–29.
Criner G. 6-minute walk testing in COPD: is it reproducible? Eur Respir J. 2011;38(2):244–5.
Hernandes NA, Wouters EF, Meijer K, Annegarn J, Pitta F, Spruit MA. Reproducibility of 6-minute walking test in patients with COPD. Eur Respir J. 2011;38(2):261–7.
Chen H, Liang BM, Tang YJ, Xu ZB, Wang K, Yi Q, et al. Relationship between 6-minute walk test and pulmonary function test in stable chronic obstructive pulmonary disease with different severities. Chin Med J. 2012;125(17):3053–8.
Google Scholar
Minami T, Manzoor K, McCool FD. Assessing diaphragm function in Chest Wall and neuromuscular diseases. Clin Chest Med. 2018;39(2):335–44.
ATS/ERS Statement on Respiratory Muscle Testing. Am J Respir Crit Care Med. 2002;166(4):518–624.
ATS statement: guidelines for the six-minute walk test. Am J Respir Crit Care Med. 2002;166(1):111–7.
Matamis D, Soilemezi E, Tsagourias M, Akoumianaki E, Dimassi S, Boroli F, et al. Sonographic evaluation of the diaphragm in critically ill patients. Technique and clinical applications. Intensive Care Med. 2013;39(5):801–10.
Jones PW, Quirk FH, Baveystock CM. The St George's respiratory questionnaire. Respir Med. 1991;85 Suppl B(25-31):discussion 3-7.
Launois C, Barbe C, Bertin E, Nardi J, Perotin JM, Dury S, et al. The modified Medical Research Council scale for the assessment of dyspnea in daily living in obesity: a pilot study. BMC pulmonary medicine. 2012;12:61.
Dhungana A, Khilnani G, Hadda V, Guleria R. Reproducibility of diaphragm thickness measurements by ultrasonography in patients on mechanical ventilation. World J Critical Care Med. 2017;6(4):185–9.
Boussuges A, Gole Y, Blanc P. Diaphragmatic motion studied by m-mode ultrasonography: methods, reproducibility, and normal values. Chest. 2009;135(2):391–400.
Rocha FR, Brüggemann AK, Francisco DS, Medeiros CS, Rosal D, Paulin E. Diaphragmatic mobility: relationship with lung function, respiratory muscle strength, dyspnea, and physical activity in daily life in patients with COPD. Jornal brasileiro de pneumologia : publicacao oficial da Sociedade Brasileira de Pneumologia e Tisilogia. 2017;43(1):32–7.
Download references
Acknowledgements
Abstract has been published/presented in the Korean tuberculosis and respiratory society, the Korean tuberculosis and respiratory society fall academic presentation | 129 volume 0342 ~ 343, total 2 PAGES, 2021
https://journal.kstudy.com/ISS_Detail.asp?key=3921544&tname=kiss2002&code=YqldZWtoSqVtJTNEOTEnMSUmN/B%20Z%20xLJTNEVHJpZSUmNbNj2bRU4XB/JTNEMA ==
This study was supported by the Research Institute for Convergence of Biomedical Science and Technology (30–2020-003), Pusan National University Yangsan Hospital. The funding body played no role in the design of the study and collection, analysis, and interpretation of data and in writing the manuscript.
Author information
Taehwa Kim and Sungchul Huh contributed equally to this work.
Authors and Affiliations
Division of Respiratory, Allergy and Critical Care Medicine, Department of Internal Medicine, Pusan National University Yangsan Hospital and Pusan National University School of Medicine, Geumo-ro 20, Beomeo-ri, Yangsan-si, Gyeongsangnam-do, 50612, Republic of Korea
Taehwa Kim, Jae Heun Chung, Yun Seong Kim & Seung Eun Lee
BioMedical Research Institute for Convergence of Biomedical Science and Technology, Pusan National University Yangsan Hospital, Yangsan, South Korea
Department of Rehabilitation Medicine, Rehabilitation Hospital, Pusan National University Yangsan, Yangsan, South Korea
Sungchul Huh & Ra Yu Yun
Pusan National University School of Medicine, Yangsan, South Korea
College of Nursing, Pusan National University, Pusan National University Yangsan Hospital, Yangsan, South Korea
You can also search for this author in PubMed Google Scholar
Contributions
Conceptualization: TK, SEL. Data acquisition and analysis: TK, OP, RYY, SH, JHC, SEL. Data interpretation: TK, RYY, SH, JHC, SEL. Validation: TK, JHC. Writing – original draft: SH, TK. Writing – review: SEL, JHC, YSK. The author(s) read and approved the final manuscript.
Corresponding author
Correspondence to Seung Eun Lee .
Ethics declarations
Ethics approval and consent to participate.
The authors are accountable for all aspects of the work in ensuring that questions related to the accuracy or integrity of any part of the work are appropriately investigated and resolved. The study was conducted in accordance with the Declaration of Helsinki (as revised in 2013) [ 17 ]. The study was approved by Pusan National University Yangsan Hospital (PNUYH) Institutional Review Board (IRB No. 05–2020-217) and individual consent for this retrospective analysis was waived.
Consent for publication
Not applicable.
Competing interests
The authors have no conflicts of interest or funding sources to declare.
Additional information
Publisher’s note.
Springer Nature remains neutral with regard to jurisdictional claims in published maps and institutional affiliations.
Supplementary Information
Additional file 1., rights and permissions.
Open Access This article is licensed under a Creative Commons Attribution 4.0 International License, which permits use, sharing, adaptation, distribution and reproduction in any medium or format, as long as you give appropriate credit to the original author(s) and the source, provide a link to the Creative Commons licence, and indicate if changes were made. The images or other third party material in this article are included in the article's Creative Commons licence, unless indicated otherwise in a credit line to the material. If material is not included in the article's Creative Commons licence and your intended use is not permitted by statutory regulation or exceeds the permitted use, you will need to obtain permission directly from the copyright holder. To view a copy of this licence, visit http://creativecommons.org/licenses/by/4.0/ . The Creative Commons Public Domain Dedication waiver ( http://creativecommons.org/publicdomain/zero/1.0/ ) applies to the data made available in this article, unless otherwise stated in a credit line to the data.
Reprints and permissions
About this article
Cite this article.
Kim, T., Huh, S., Chung, J.H. et al. Clinical values of diaphragmatic movement in patients with chronic obstructive pulmonary disease. BMC Pulm Med 23 , 33 (2023). https://doi.org/10.1186/s12890-022-02220-7
Download citation
Received : 25 April 2022
Accepted : 02 November 2022
Published : 27 January 2023
DOI : https://doi.org/10.1186/s12890-022-02220-7
Share this article
Anyone you share the following link with will be able to read this content:
Sorry, a shareable link is not currently available for this article.
Provided by the Springer Nature SharedIt content-sharing initiative
- Cut-off value
BMC Pulmonary Medicine
ISSN: 1471-2466
- Submission enquiries: [email protected]
- General enquiries: [email protected]

Current time by city
For example, New York
Current time by country
For example, Japan
Time difference
For example, London
For example, Dubai
Coordinates
For example, Hong Kong
For example, Delhi
For example, Sydney
Geographic coordinates of Elektrostal, Moscow Oblast, Russia
City coordinates
Coordinates of Elektrostal in decimal degrees
Coordinates of elektrostal in degrees and decimal minutes, utm coordinates of elektrostal, geographic coordinate systems.
WGS 84 coordinate reference system is the latest revision of the World Geodetic System, which is used in mapping and navigation, including GPS satellite navigation system (the Global Positioning System).
Geographic coordinates (latitude and longitude) define a position on the Earth’s surface. Coordinates are angular units. The canonical form of latitude and longitude representation uses degrees (°), minutes (′), and seconds (″). GPS systems widely use coordinates in degrees and decimal minutes, or in decimal degrees.
Latitude varies from −90° to 90°. The latitude of the Equator is 0°; the latitude of the South Pole is −90°; the latitude of the North Pole is 90°. Positive latitude values correspond to the geographic locations north of the Equator (abbrev. N). Negative latitude values correspond to the geographic locations south of the Equator (abbrev. S).
Longitude is counted from the prime meridian ( IERS Reference Meridian for WGS 84) and varies from −180° to 180°. Positive longitude values correspond to the geographic locations east of the prime meridian (abbrev. E). Negative longitude values correspond to the geographic locations west of the prime meridian (abbrev. W).
UTM or Universal Transverse Mercator coordinate system divides the Earth’s surface into 60 longitudinal zones. The coordinates of a location within each zone are defined as a planar coordinate pair related to the intersection of the equator and the zone’s central meridian, and measured in meters.
Elevation above sea level is a measure of a geographic location’s height. We are using the global digital elevation model GTOPO30 .
Elektrostal , Moscow Oblast, Russia

An official website of the United States government
The .gov means it’s official. Federal government websites often end in .gov or .mil. Before sharing sensitive information, make sure you’re on a federal government site.
The site is secure. The https:// ensures that you are connecting to the official website and that any information you provide is encrypted and transmitted securely.
- Publications
- Account settings
Preview improvements coming to the PMC website in October 2024. Learn More or Try it out now .
- Advanced Search
- Journal List
- Minim Invasive Surg
- v.2016; 2016

Comparison of Diaphragmatic Breathing Exercise, Volume and Flow Incentive Spirometry, on Diaphragm Excursion and Pulmonary Function in Patients Undergoing Laparoscopic Surgery: A Randomized Controlled Trial
Gopala krishna alaparthi.
1 Department of Physiotherapy, Kasturba Medical College, Manipal University, Bejai, Mangalore 575004, India
Alfred Joseph Augustine
2 Department of Surgery, Kasturba Medical College, Manipal University, Mangalore 575004, India
3 Department of Pulmonary Medicine, Kasturba Medical College, Manipal University, Mangalore 575004, India
Ajith Mahale
4 Department of Radiodiagnosis, Kasturba Medical College, Manipal University, Mangalore 575004, India
Objective. To evaluate the effects of diaphragmatic breathing exercises and flow and volume-oriented incentive spirometry on pulmonary function and diaphragm excursion in patients undergoing laparoscopic abdominal surgery. Methodology. We selected 260 patients posted for laparoscopic abdominal surgery and they were block randomization as follows: 65 patients performed diaphragmatic breathing exercises, 65 patients performed flow incentive spirometry, 65 patients performed volume incentive spirometry, and 65 patients participated as a control group. All of them underwent evaluation of pulmonary function with measurement of Forced Vital Capacity (FVC), Forced Expiratory Volume in the first second (FEV 1 ), Peak Expiratory Flow Rate (PEFR), and diaphragm excursion measurement by ultrasonography before the operation and on the first and second postoperative days. With the level of significance set at p < 0.05. Results. Pulmonary function and diaphragm excursion showed a significant decrease on the first postoperative day in all four groups ( p < 0.001) but was evident more in the control group than in the experimental groups. On the second postoperative day pulmonary function (Forced Vital Capacity) and diaphragm excursion were found to be better preserved in volume incentive spirometry and diaphragmatic breathing exercise group than in the flow incentive spirometry group and the control group. Pulmonary function (Forced Vital Capacity) and diaphragm excursion showed statistically significant differences between volume incentive spirometry and diaphragmatic breathing exercise group ( p < 0.05) as compared to that flow incentive spirometry group and the control group. Conclusion . Volume incentive spirometry and diaphragmatic breathing exercise can be recommended as an intervention for all patients pre- and postoperatively, over flow-oriented incentive spirometry for the generation and sustenance of pulmonary function and diaphragm excursion in the management of laparoscopic abdominal surgery.
1. Introduction
Chest physiotherapy is a common practice in patients undergoing cardiothoracic and abdominal surgery [ 1 ]. Abdominal surgery that was previously performed via a large incision is now more commonly performed laparoscopically [ 2 ]. The laparoscopic surgeries involve structures such as the gall bladder, colon, small intestine, stomach, liver, and pancreas [ 1 ].
In laparoscopy, intraoperative pulmonary changes are due to decreased pulmonary compliance secondary to upward movement of the diaphragm during insufflation and to changes in carbon dioxide (CO 2 ) homeostasis secondary to absorption of insufflated CO 2 from peritoneum [ 3 ]. General anesthesia and surgery related pain may lead to changes in the ventilation pattern resulting in the patient taking shallow breaths which reduce the ability to clear sputum from the chest [ 4 – 6 ].
Studies have reported altered pulmonary function after both conventional and laparoscopic abdominal surgeries [ 7 – 12 ]. Postoperative pulmonary dysfunction in laparoscopic surgery is approximately 20% to 25% depending upon the type of surgery [ 7 – 9 ]. Pulmonary dysfunction leads to pulmonary complications which includes atelectasis, pneumonia, tracheobronchial infection, and respiratory failure. These may have an adverse effect on the length of hospital stay [ 4 ].
Reduction of pulmonary function, Forced Vital Capacity (FVC), and Forced Expiratory Vital Capacity (FEV 1 ) have been reported on the basis of functional alterations [ 13 ]. Pathogenesis of postoperative pulmonary dysfunction has been attributed to diaphragmatic function impairment [ 14 ].
Chest physiotherapy has been employed as an alternative intervention to reduce occurrence of pulmonary function loss and its complications. Postoperative chest physiotherapy started being implemented in the beginning of the 20th century. It includes breathing exercises, percussion, vibration, splinted huffing/coughing, positioning, and mobilization [ 15 ].
Diaphragmatic breathing exercises are used in order to augment diaphragmatic descent while inhalation and diaphragmatic ascent while expiration. The beneficial effects of diaphragmatic breathing are as follows: inflation of the alveoli, reversing postoperative hypoxemia, improvement of ventilation and oxygenation, decreasing the work of breathing, and increasing the degree of excursion of the diaphragm [ 16 , 17 ].
Mechanical breathing device such as the incentive spirometry (IS) has been introduced into clinical practice [ 13 ]. Incentive spirometry encourages the patient to take long, slow deep breath mimicking natural sighing and also provides a visual positive feedback. Incentive spirometers are available either by volume of inspiration (volume-oriented) or flow rate (flow-oriented) [ 4 – 6 , 18 – 20 ].
The flow-oriented incentive spirometer (Triflow device) consists of three chambers in series, each of which contains a ball. When the patient's effort generates a subatmosphere pressure above the ball, it rises in the chamber. An inspiratory flow of 600 mL/s is required to raise the first ball, an inspiratory flow of 900 mL/s is required to elevate the first and second balls, and a flow of 1200 mL/s is required to elevate all three balls. The volume-oriented incentive spirometer is a compact device of 4000 mL capacity and has a one-way valve to prevent exhalation into the unit. A sliding pointer indicates the prescribed inspiratory volume and an inspiratory flow guide coaches the subject to inhale slowly [ 18 – 20 ].
Studies suggest a physiologically significant difference in the effect of the flow- and volume-oriented incentive spirometer. Flow-oriented devices (Triflow device) enforce more work of breathing and increase muscular activity of the upper chest. Volume-oriented devices (Coach 2 device) enforce less work of breathing and improve diaphragmatic activity [ 6 , 18 – 21 ].
Earlier studies show that the volumetric incentive spirometer is better in case of cardiac and thoracic surgeries because it provides the appropriate feedback for a slow sustained inspiration and volume [ 18 ]. Studies show that slow sustained inspirations are much more effective to promote lung expansion rather than fast inspirations [ 18 ]. Studies also show that diaphragmatic breathing exercise encourages more diaphragmatic movement [ 17 , 18 ].
Gastaldi et al. studied thirty-six subjects, in order to assess the effect of respiratory kinesiotherapy on respiratory muscle strength and pulmonary function following laparoscopic cholecystectomy. Subjects were randomly sorted into two groups: the exercise and the control. Three breathing exercises were performed by seventeen subjects while other nineteen served as a control group. All the subjects were assessed for Maximal Inspiratory Pressure (MIP) and Maximal Expiratory Pressure (MEP), PEF, and spirometry (FVC, FEV 1 , and FEV 1 /FVC). Both groups registered a decrease in all variables on the first day after surgery. On the second postoperative day, the exercise group showed decreased values for all variables. The values then normalized. However, values of all variables for the control group begin to normalize only on the fifth postoperative day [ 22 ].
El-Marakby et al. carried out a study on two experimental groups of patients in order to evaluate the effects of aerobic exercise training and incentive spirometry in controlling pulmonary complications following laparoscopic cholecystectomy. One group was given aerobic walking raining and incentive spirometry as well as traditional physical therapy (Group A); the other (Group B) was given traditional physical therapy. Results indicated a significant reduction in heart rate, SaO 2 , and inspiratory capacity for both groups. The researchers concluded that aerobic exercise and incentive spirometry were beneficial in reducing the postoperative pulmonary complications after laparoscopic cholecystectomy [ 23 ].
Kundra et al. carried out a comparative study on the effect of preoperative and postoperative incentive spirometry on the pulmonary function of fifty patients who had undergone laparoscopic cholecystectomy. The study group had to carry out incentive spirometry fifteen times before surgery, every four hours, for one week. However, the control group underwent incentive spirometry only during the postoperative period. Pulmonary function was recorded before surgery and 6, 24, and 48 hours postoperatively and at the time of discharge. Result showed that pulmonary function improvement was seen after preoperative incentive Spirometry. The authors concluded that pulmonary function is well-preserved with preoperative than postoperative incentive spirometry [ 24 ].
Fagevik Olsén et al. reviewed forty-four studies in order to evaluate the effects of chest physiotherapy interventions in laparoscopic and open abdominal surgery. But the results showed that breathing exercises were efficacious in preventing postoperative pulmonary complications in patients undergoing open surgery. The review also showed that laparoscopic procedures impair respiratory function to a considerably lower degree than open surgery. One study in the review showed that routine treatment is not called for in upper gastrointestinal features such as, for instance, fundoplication and vertical banded gastroplasty [ 1 ].
Cattano et al. studied forty-one morbidly obese to assess use of incentive spirometry preoperatively which could help patients to preserve their pulmonary function (inspiratory capacity) better in the postoperative period following laparoscopic bariatric surgery. Subjects were randomly sorted into two groups (the exercise and the control group). The exercise group used the incentive spirometer for ten breaths, five times per day. The control group used incentive spirometer three breaths, once per day. Pulmonary function (inspiratory capacity) was recorded at the day of surgery and postoperative day 1. The author concluded that preoperative use of the incentive spirometer does not lead to significant improvement of pulmonary function (inspiratory capacity) [ 25 ].
Various chest physiotherapy techniques are used clinically as part of the routine prophylactic and therapeutic regimen in postoperative respiratory care. However, the efficacy of flow and volume incentive spirometry and diaphragmatic breathing exercise is still controversial [ 6 , 17 ].
There are no retrievable studies that have been done on the clinical efficacy of diaphragmatic breathing exercise and flow and volume incentive spirometry after laparoscopic abdominal surgery. With this background the present study aim is to compare the effect of diaphragmatic breathing exercise, flow and volume incentive spirometry, on pulmonary function and diaphragm excursion, following laparoscopic surgery.
2. Material and Method
2.1. inclusion criteria.
Inclusion criteria involved subjects of either gender in the age group of 18 to 80 years who were posted for laparoscopic abdominal surgery.
2.2. Exclusion Criteria
Exclusion criteria were as follows:
- Patients who had undergone open abdominal surgery and laparoscopic obstetrics and gynecological surgery.
- Patients with unstable hemodynamic parameters (arterial pressure <100 mmHg systolic and <60 mmHg for diastolic and mean arterial pressure (MAP) <80 mmHg).
- Patients with postoperative complications requiring mechanical ventilation.
- Uncooperative patients or patients unable to understand or to use the device properly.
- Patients with inadequate inspiration characterized by vital capacity <10 mL/kg.
2.3. Equipment Used
Equipment used was as follows:
- Ultrasonography machine (Voluson730).
- Pulmonary function test machine (EasyOne Plus Portable Diagnostic Spirometer Machine, ndd Medical Technologies, Inc. Massachusetts, USA).
- Flow-oriented incentive spirometry machine (Triflow device, IGNA Medical Devices, Mumbai).
- Volume-oriented incentive spirometry machine (Coach 2 device, Smiths Medical International Ltd., USA).
2.4. Procedure
The study was carried out in Kasturba Medical College Hospitals Mangalore over a period of four years starting from January 2011 to December 2014. The study was approved by the Institutional Ethics Committee of Kasturba Medical College Mangalore. Eligible patients were selected based on the inclusion and exclusion criteria. The purpose of study was made clear to each patient and a written informed consent was obtained prior to involving them in the study.
The patients were divided into four groups:
- Flow-oriented incentive spirometry group (Triflow device).
- Volume-oriented incentive spirometry group (Coach 2 device).
- Diaphragmatic breathing exercise group.
- Control group.
The patients were allocated to groups by block randomization done by primary investigator. The entire sample was divided into 13 blocks with 20 patients in each, 5 belonging to each group. Group information was concealed in a sealed opaque envelope and revealed to the patients only after they were recruited into the treatment group or the control group done by primary investigator.
Following the allocation to groups, the patients in the treatment group were visited one day prior to the surgery; preoperative information was offered and, based upon his/her group, flow-oriented incentive spirometry, volume-oriented incentive spirometry, or diaphragmatic breathing exercise was taught to each patient. Other therapies like airway clearance techniques, thoracic expansion exercise, and mobilization were also taught to every patient in all treatment groups (see Steps 1 – 5 ). Patients in the control group were not given any treatment or taught any exercises. The treatment protocol for postoperative laparoscopic abdominal surgery is as follows.
The first step is diaphragmatic breathing exercise, flow or volume incentive spirometry (3 sets, 5 repetitions of deep breaths).
The second step is airway clearance techniques (huffing or coughing).
The third step is circulation (foot and ankle pumping, hip and knee bending 10 times each hour).
The fourth step is thoracic expansion exercise (position patient in long sitting in bed/high sitting over the side of the bed).
The fifth step is mobilization:
- Sitting out of the bed in a chair (one hour twice daily).
- Walking (three times per day).
- Stair climbing done before the patient was discharged from the hospital.
An experienced radiologist carried out ultrasonography for diaphragm excursion on the preoperative as well as the 1st and 2nd postoperative day, for all groups.
Pulmonary function tests (PFT) measured the following variables: Forced Vital Capacity (FVC), Forced Expiratory Volume in the first second (FEV 1 ), Peak Expiratory Flow Rate (PEFR). These were taken on the preoperative day and 1st and the 2nd postoperative day, for all groups. These measurements were taken by the primary investigator ( Figure 1 , flowchart).

Consort flow diagram of the study.
3. Description of Outcome Measures
3.1. diaphragm excursion.
The patient lays in the supine position and diaphragm movements were recorded in the B-Mode. The probe was positioned between the midclavicular and anterior axillary lines, in the subcostal area, so that the ultrasound beam entered the posterior third of the right hemidiaphragm perpendicularly. The procedure began at the end of normal expiration with the subjects being instructed to inhale as deeply as possible. A fixed point at the edge of the image on the screen and the diaphragm margin at maximal inspiration and again at maximal expiration served as reference points between which measurements were made, with the average of three values being taken for both maximal inspiration and maximal expiration [ 26 , 27 ].
3.2. Pulmonary Function Test
Pulmonary function test procedures (EasyOne Plus Portable Diagnostic Spirometer Machine) were carried out according to the American Thoracic Society/European Respiratory Society guidelines [ 28 ]. The following variables have been recorded: Forced Vital Capacity (FVC), Forced Expiratory Volume in the first second (FEV 1 ), and Peak Expiratory Flow Rate (PEFR) the best value of 3 acceptable tests [ 29 ].
3.3. Treatment Procedures
3.3.1. methods to perform flow-oriented and volume-oriented incentive spirometry.
The patient was placed in a semirecumbent position (45°), with a pillow under the knees. The patient was instructed to inhale with a slow and deep sustained breath, holding it for a minimum of 5 seconds and then to exhale passively in order to avoid any forceful expiration. First, the patient was given demonstration and then asked to perform in order to ensure that she/he understood the process [ 15 , 17 ]. The patient was instructed to hold the spirometer upright and to perform flow-oriented incentive spirometry by inhaling slowly and thereby raising the ball, followed by volume incentive spirometry in order to raise the piston or plate in the chamber to the set target [ 19 , 20 ].
The patient was instructed to perform 3 sets of 5 repeated deep breaths. This had to be performed by the patient every waking hour. The therapist administered the exercise four times a day and the patient was instructed to perform the same for the rest of the day [ 19 ]. The patient was asked to keep a record of the exercise performed by entering in a log book which was provided beforehand.
3.3.2. Method to Perform Diaphragmatic Breathing Exercise
The patient assumed a semi-Fowler's position (back and head are fully supported and abdominal wall is relaxed) and performed diaphragmatic breathing. The therapist placed his hands just below the anterior costal margin, on the rectus abdominis, while the patient was instructed to inhale slowly and deeply through the nose, from functional residual capacity to total lung capacity with a three-second inspiratory hold. The patient was then instructed to relax the shoulders, keep the upper chest quiet in order that the abdomen be raised a little. The Patient was then instructed to exhale slowly through the mouth [ 16 , 28 ].
The Patient was made to experience a slight rise and subsequent fall of the abdomen during inspiration and expiration, by placing his or her own hand below the anterior costal margin. The Patient was instructed to perform 3 sets of 5 deep breaths with the therapist administering them four times a day and the patient being instructed to perform the same once every waking hour for the rest of the day. In between the repetitions of the diaphragmatic breathing exercise, the patient was told to breathe normally [ 16 , 28 ]. The patient was asked to keep a record of the exercise performed by entering in a log book which was provided beforehand.
3.4. Data Analysis
3.4.1. sample size.
The sample size was calculated based on the values obtained from pulmonary function test in a pilot study (20 subjects, 5 in each group) [ 30 , 31 ]. The following formula was used for calculating the same:
where n is the number of subjects in each group and Zα and Zβ are constants and they are substituted. Selected power for the study was 90% and D is effect size which is the absolute value of the difference in means and represents what is considered a clinically meaningful or practically important difference in means.
D is taken from the pilot study which used the same variable, which compared pulmonary function test in subjects, and S is the standard deviation of the means. The sample size is 65 in each group (total 260 subjects).
Data was analyzed using SPSS package version 21. ANOVA and post hoc analysis (Bonferroni's t -test) were carried out to verify the within-groups differences. Between-groups differences were compared using two-factor ANOVA.
We selected 274 patients posted for laparoscopic abdominal surgery, of which 260 were included in the study. Fourteen patients were excluded because they were converted to an open surgical procedure. There were 195 patients in the intervention groups and 65 in the control group.
Baseline demographic characteristics of the participants such as age, height, weight, BMI, risk factors, and duration of surgery are presented in Table 1 . There were no statistically significant differences between the groups. Data about Patients who underwent different types of laparoscopic abdominal surgery are summarized in Table 1 . Of the 260 patients included, 140 patients underwent cholecystectomy, 53 hernioplasty, 43 appendectomy, 11 umbilical hernia repair, 8 laparoscopic diagnostic, 3 bariatric surgery, and 2 hemicolectomy.
Demographic characteristics of subjects undergoing laparoscopic abdominal surgery.
Forced Vital Capacity (FVC) was compared within the intervention groups and the control group before and after operation, and the same is summarized in Table 2 . There was a statistically significant decrease in Forced Vital Capacity (FVC) in the 1st and 2nd post-op day when compared with the preoperative period in all groups.
Comparison of Forced Vital Capacity (FVC) before and after the laparoscopic abdominal surgery in the intervention groups and control group.
% change. ∗∗ Highly significant at p < 0.001 level.
Forced Expiratory Volume in one second (FEV 1 ) was compared within the intervention groups and the control group before and after the operation and is summarized in Table 3 . There was a statistically significant decrease in Forced Expiratory Volume at the end of the first second (FEV 1 ) on the 1st and 2nd postoperative day when compared with the preoperative period in all groups.
Comparison of Forced Expiratory Volume in one second (FEV 1 ) before and after the laparoscopic abdominal surgery in the intervention groups and control group.
Peak Expiratory Flow Rates (PEFR) were compared with the intervention groups and control group before and after operation and are summarized in Table 4 . In all groups there was a statistically significant decrease in Peak Expiratory Flow Rate (PEFR) on the 1st and 2nd postoperative day compared to the preoperative period.
Comparison of Peak Expiratory Flow Rate (PEFR) before and after the laparoscopic abdominal surgery in the intervention groups and the control group.
Diaphragm excursions were compared within intervention groups and the control group before and after operation and are summarized in Table 5 . There was a statistically significant decrease in diaphragm excursion in the 1st and 2nd postoperative period when compared with the preoperative period in all groups except in diaphragmatic breathing exercise group and volume incentive spirometry group which almost came back to normal.
Comparison of diaphragm excursion before and after the laparoscopic abdominal surgery in the intervention groups and the control group.
% change. # Not significant at p > 0.05. ∗∗ Highly significant at p < 0.001 level.
Forced Vital Capacity (FVC), Forced Expiratory Volume in one second (FEV 1 ), Peak Expiratory Flow Rate, and diaphragm excursion were compared between the intervention groups and the control group during the preoperative and 2nd postoperative day and are summarized in Table 6 .
Showing difference between preoperative and postoperative 2nd day between intervention groups and control group of Forced Vital Capacity, Forced Expiratory Volume in one second, Peak Expiratory Flow Rate, and diaphragm excursion.
# Not significant at p > 0.05. ∗ Significant at p < 0.05 level. ∗∗ Highly significant at p < 0.001 level.
There was a statistically significant difference between intervention groups (diaphragmatic breathing exercise group and volume incentive spirometry group) and control group in terms of Forced Vital Capacity (FVC) and diaphragm excursion ( p < 0.001), the said variables being significantly lower in the control group than in the diaphragmatic breathing exercise group and volume incentive spirometry group.
5. Discussion
The main purpose of this study was to compare diaphragmatic breathing exercise, flow- and volume incentive spirometry, on pulmonary function and diaphragmatic excursion in patients undergoing laparoscopic abdominal surgery. To the best of our knowledge, this study is the first to compare the effects of diaphragmatic breathing exercise with two different kinds of incentive spirometry and also against a control group. There were 65 patients included in each group and the four groups were homogenous in terms of all demographic parameters. In our study we found that diaphragmatic breathing exercise and volume incentive spirometry improve lung function and diaphragm excursion in patients undergoing laparoscopic abdominal surgery.
In our study pulmonary function (FVC, FEV 1 , and PEFR) and diaphragm excursion showed a decrease on the 1st postoperative day when compared to the preoperative values in all four groups with an average decrease of 27% in Forced Vital Capacity, 28% in Forced Expiratory Volume in one second, 37% in Peak Expiratory Flow Rate, and 28% in diaphragm excursion. The present study finding of reduction in pulmonary function during postoperative day is similar to those reported in a previous study [ 3 , 21 – 24 ].
Our results are in accordance with Schauer et al. who found 30% to 38% reduction in postoperative pulmonary function (FVC, FEV 1 , and FEF25%–75%) in laparoscopic cholecystectomy [ 9 ]. Karayiannakis et al. found 22% of FVC and 19% of FEV 1 reduction after laparoscopic cholecystectomy [ 32 ]. Ramos et al. found 20% to 30% reduction in postoperative pulmonary function (FVC and FEV 1 ) in laparoscopic cholecystectomy [ 33 ]. Ravimohan et al. found 21% to 31% reduction in postoperative day pulmonary function variables (FVC, FEV 1 , and FEF25%–75%) in laparoscopic cholecystectomy [ 7 ].
Possible reasons for decrease in pulmonary function and diaphragm excursion during the postoperative period in laparoscopic abdominal surgery are as follows. During the postoperative period, patients exhibit shallow breathing without the intermittent sigh or breaths which are inspired approximately ten times an hour. Patients will breathe shallowly which leads to a decrease in ventilation to dependent lung regions [ 7 , 32 , 33 ]. In the present study, reduced pulmonary function (FVC, FEV 1 , and PEFR) and diaphragm excursion in postoperative laparoscopic abdominal surgery subjects might be due to postoperative pain, location of surgical ports, along with anaesthetic, analgesic usage [ 7 , 34 ].
The effects of general anaesthesia on distribution of ventilation and chest wall and lung mechanics lead to ventilation-perfusion mismatch, increased dead space, shunt, and hypoxemia [ 9 , 35 , 36 ]. Narcotic/opioid analgesics and other drugs affect the central regulation of breathing, changing the neural drive of the upper airway and chest wall muscles, which lead to hypoventilation, a diminished sensitivity of the respiratory center to carbon dioxide stimulation, an increase of obstructive breathlessness, the suppression of the cough reflex, and irregular mucus production [ 37 ].
The location of surgical ports involves trauma near the diaphragm and chest wall/ribs, leading to postoperative incisional pain and reflex inhibition of the phrenic nerve and diaphragmatic reflex paresis resulting in functional disruption of respiratory muscle movement. In addition, when patients remain lying down for long periods during the postoperative period their abdominal content limits diaphragmatic movement [ 34 ].
Several studies found that diaphragmatic dysfunction is due to gas insufflation in the abdominal cavity which might also be responsible for the increase of resistance and reduced diaphragmatic excursion, leading to reduced lung volume [ 38 ]. All these factors lead to a change in postoperative lung function usually resulting in development of a restrictive pattern and decreased diaphragm excursion in laparoscopic abdominal surgery.
Our results are in accordance with Ford et al., who showed that reduction in inspiratory muscle activity, mainly the diaphragm, was the main determinant of impaired pulmonary function. Diaphragm dysfunction may be due to reflex inhibition of efferent phrenic activity [ 39 ]. Several studies suggested that laparoscopic abdominal surgery causes reflex inhibition of the phrenic nerve which might lead to shallow breaths and reduced pulmonary ventilation [ 34 ]. Erice et al. explained reduced pulmonary ventilation mainly due to decreased inspiratory muscle activity [ 40 ]. Lunardi et al. showed a decrease of 27% in the respiratory muscular activity of patients who underwent laparoscopy abdominal surgery [ 41 ].
Possible reasons for improved pulmonary function and diaphragm excursion in the diaphragmatic breathing exercise group are as follows. The present study showed that the diaphragmatic breathing exercise group was able to improve pulmonary mechanics thus leading to a beneficial effect on pulmonary function (FVC) and diaphragm excursion. Diaphragmatic breathing exercise improves diaphragmatic descent and diaphragmatic ascent during inspiration and expiration, respectively. Slower deep inspiration ensures more even distribution of air throughout the lung, particularly to the dependent lung [ 16 ]. The physiological effects of diaphragmatic breathing exercise are that breathing through full vital capacity and holding for 3–5 seconds ensure full inflation of the lungs thus opening up alveoli which have low volume and stimulating the production of surfactant. Diaphragmatic breathing exercise will also decrease activity of accessory muscles, ensure that breathing patterns are as close to normal as possible, and also reduce the work of breathing [ 16 , 31 ].
Our results are in accordance with the findings of Tahir et al. who showed that diaphragmatic breathing exercise will improve basal ventilation [ 42 ]. Weber and Prayar and Menkes and Britt found that diaphragmatic breathing exercise will improve tidal volume and also facilitate secretion removal [ 43 , 44 ]. Blaney and Sawyer observed that tactile stimulation over the subject's lower costal margin as well as verbal instruction served to significantly increase diaphragmatic movement during diaphragmatic breathing exercises [ 45 ]. Manzano et al. found that diaphragmatic breathing exercise was able to improve pulmonary mechanics and lead to beneficial effect on Forced Vital Capacity (FVC) [ 46 ]. Grams et al. evaluated the efficacy of diaphragmatic breathing exercise for the prevention of postoperative pulmonary complications and for the recovery of pulmonary mechanics and found that diaphragmatic breathing exercise appeared to be more effective [ 17 ].
Possible reasons for improved pulmonary function and diaphragmatic excursion in the volume incentive spirometry group are as follows. The present study showed that the volume incentive spirometry group also had improved pulmonary mechanics that led to a beneficial effect on pulmonary function (FVC) and diaphragm excursion. After laparoscopic abdominal surgery, it may be hard to take a deep breath and if patients do not breathe deeply it may lead to postoperative pulmonary complications. The volume incentive spirometer is a mechanical device used to take slow, deep long breaths that encourage patients to breathe to total lung capacity, to sustain that inflation and open up collapsed alveoli [ 18 ].
The volume incentive spirometer will be more “physiological” because the training volume is constant until it reaches the maximum inspiratory capacity (level preset by physiotherapist). It provides a low level of resistance training while minimizing the potential fatigue to the diaphragm [ 19 ]. Our study results are in accordance with Paisani et al. who showed that when volume incentive spirometry was performed with low inspiratory flow it promoted diaphragmatic excursion and improved the expansion of the basal area of chest wall [ 21 ]. Minschaert et al. observed that patients treated with incentive spirometry would have early recovery of the pulmonary volume [ 47 ]. Kundra et al. found that the use of incentive spirometry in the preoperative period leads to greater improvement in the lung functions than if given in the postoperative period. So use of the volume incentive spirometer will result in active recruitment of the diaphragm and other inspiration muscles which may lead to improved pulmonary function and diaphragm excursion [ 24 ].
Limitation of the Study . There was no blinding in the study procedure; the same investigator who randomized the patients into the experimental groups and the control group measured the outcome variables (pulmonary function test) and the same investigator taught the exercises to all experimental groups. Diaphragm excursion measurement was not done by the same radiologist throughout the study and the finding would have been confounded by the expertise of professional. Type of anaesthesia, analgesia, and postoperative pain was not recorded which could affect the findings. There was no follow-up in the study as all patients were discharged on the 2nd postoperative day. As a result we are unaware which group values returned to normal. Patient adherence to the intervention programs was recorded by providing a log book to each subject, in which they had to make an entry the very time they did the prescribed technique but there is no way to verify the authenticity of these entries.
6. Suggestions for the Future Research
Future research could be directed at long-term follow-up to see which group sustains improvement for a long duration and the functional aspect of recovery. Future studies can be carried out to compare the effect of the techniques on patients who have undergone upper and lower abdominal laparoscopic surgeries, using a larger sample size. Effect of combining therapy like incentive spirometer and diaphragmatic breathing exercise can be studied on laparoscopic abdominal surgery patients. Future research can be done by assessing and using respiratory muscle strength and patient comfort with different technique as an outcome in laparoscopic abdominal surgery. Similar studies can be conducted on patients following open abdominal surgeries and cardiac and thoracic surgeries.
7. Clinical Implication
Based on the results of the study we strongly recommend the following:
- Volume-oriented incentive spirometry and diaphragmatic breathing exercise can be recommended for all patients preoperatively and postoperatively over flow-oriented incentive spirometry as an intervention for the generation and sustenance of pulmonary function and diaphragm excursion in the management of laparoscopic abdominal surgery.
8. Conclusion
- (i) From our study we conclude that in laparoscopic abdominal surgery patients there is a significant decrease in pulmonary function (FVC, FEV 1 , and PEFR) and diaphragm excursion in all four groups on the 1st postoperative day when compared with the preoperative day.
- (ii) A greater improvement in pulmonary function and diaphragm excursion between the first and second postoperative day was seen in all experimental groups when compared to the control group.
- (iii) From our study we conclude that pulmonary function and diaphragm excursion was better preserved in the diaphragmatic breathing exercise group and volume incentive spirometry group when compared with the flow incentive spirometry group and the control group.
Acknowledgments
The authors are extremely grateful to Dr. Anil Sharma, Senior Consultant Surgeon, Max Super Specialty Hospital, New Delhi, for all timely help, for valuable expert advice and suggestion, and for being the source of inspiration.
Competing Interests
The authors declare that there are no competing interests.
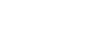
- Yekaterinburg
- Novosibirsk
- Vladivostok
- Tours to Russia
- Practicalities
- Russia in Lists
Rusmania • Deep into Russia
Moscow metro excursion
Plan your next trip to russia, ready-to-book tours.
Your holiday in Russia starts here. Choose and book your tour to Russia.
REQUEST A CUSTOMISED TRIP
Looking for something unique? Create the trip of your dreams with the help of our experts.
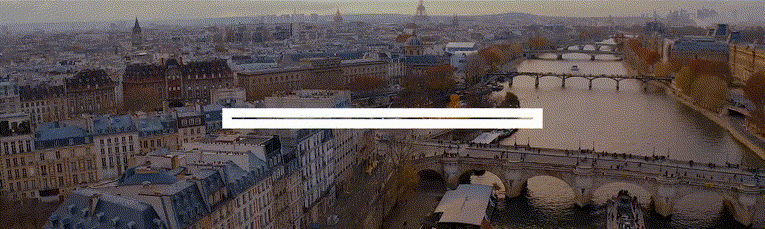
IMAGES
VIDEO
COMMENTS
- Diaphragmatic excursion is affected by the abdominal contents and pressure, which limit diaphragm displacement. 22 Open in a separate window. Open in a separate window. Figure 1. ... In C, schematic representation of the measurement of diaphragmatic excursion: on the left, placement of the probe in the subcostal region to display the ...
Diaphragmatic excursion. Diaphragmatic excursion is the movement of the thoracic diaphragm during breathing. Normal diaphragmatic excursion should be 3-5 cm, but can be increased in well-conditioned persons to 7-8 cm. This measures the contraction of the diaphragm. It is performed by asking the patient to exhale and hold it.
Patients with history of diaphragmatic pathology or recent thoracic or upper abdominal surgery were not included. Ultrasound examination of the diaphragm ... Diaphragmatic excursion is positively correlated with lung inspiratory volumes and can accurately reflect the muscle strength and function , . Furthermore, diaphragmatic excursion is an ...
The mean diaphragmatic excursions of the two hemidiaphragms have been determined for men and women (Table (Table1). 1). Furthermore, in 1995, Houston et al have reported that in healthy volunteers, the right-to-left ratio of hemidiaphragmatic excursion during deep inspiration was in the range of 0.5-1.6. Consequently, this ratio has been ...
Diaphragm excursion was measured using an ultrasound imaging device (FAMUBO-Z; Seiko, Japan) with a convex probe, and maximum diaphragm excursion (DE-max) was calculated. The oblique scanning under the rib arch method was used for measurement [9, 17]. In B-mode, the probe was scanned obliquely along the rib margin under the rib arch in the long ...
The diaphragm, the major respiratory muscle, is responsible for approximately 60-80% of the workload 15 with an excursion of 1-2 cm, while during the forced breathing its amplitude is up to 7-11 cm. 4 MV has been proved to induce several diaphragmatic abnormalities, leading to atrophy and contractile dysfunction of diaphragm (VIDD) 16 ...
phragmatic excursion may be detected by percus- ... inspiration. The most characteristic physical sign of diaphragmatic dysfunction is abdominal para-dox, which is the paradoxical inward motion of ...
Normal diaphragm excursion is between 3 and 5 cm. An abnormal sniff test is the paradoxic motion of a hemidiaphragm upward by greater than 2 cm which can indicate diaphragm paralysis ... Abdominal approaches to diaphragm plication may be performed laparoscopically or open. The position of the liver makes transabdominal diaphragm plication more ...
a median of the abdominal perimeter of 78 cm. The thickness of the diaphragm was 3.4 mm on inspiration and 2.6 mm on expiration, with a mean diaphragmatic excursion of 15 mm. ... Diaphragmatic excursion measured with ultrasound is increasin-gly useful in the thoracoabdominal assessment of patients. Perhaps
Using diaphragm excursion to predict weaning success should therefore be measured in patients undergoing a spontaneous breathing trial, such as a T-piece trial (disconnecting the patient from the ventilator) or a ZEEP trial (decreasing the pressure support to minimal values with PEEP set at 0 cm H 2 0). In this situation, the excursion measured will hence apprehend the diaphragmatic ...
The diaphragm (dia: across, phragm: fence) is the musculotendinous boundary between the negative-pressure thoracic cavity and positive-pressure abdominal cavity. The diaphragm plays a significant role in respiratory mechanics, and injury to the diaphragm impairs ventilation and oxygen delivery. The normal mechanics of respiration are discussed ...
In case of a unilateral weakening of the hemidiaphragm, the diaphragmatic excursion will be reduced or absent. Sometimes, it can show a paradoxical movement of the diaphragm where the diaphragm moves into thoracic cavity during inspiration while the other normal working diaphragm moves downward toward the abdominal cavity.
The diaphragm is the dome-shaped structure that separates the thoracic and abdominal cavities. It is the principal muscle of respiration, is innervated by the phrenic nerves that arise from the ...
abdominal surgery 65 patients for each pattern of breathing, and diaphragm excursion measurement by ultrasonography before the operation and on the first and second postoperative days. Pulmonary ...
Diaphragm excursions and tidal volumes were simultaneously measured in invasively ventilated patients (N = 21) and healthy volunteers (N = 20). Linear mixed models were used to estimate the ratio between tidal volume and diaphragm excursion. The tidal volume-diaphragm excursion ratio was 201 mL/cm in ICU patients [95% confidence interval (CI ...
Keywords: Diaphragmatic excursion, Expiratory abdominal muscles, Expiratory muscle thickness pattern, External oblique, Internal oblique, Rectus abdominis, Transversus abdominis. Indian Journal of Critical Care Medicine (2022): 10.5005/jp-journals-10071-24125 IntroductIon Expiratory muscles consist of the rib cage muscles (internal
Diaphragmatic function and BMI (body mass index) To evaluate the function of the diaphragm muscle [], the diaphragmatic excursion was measured at rest and during forced expiration (Supplement Table 1).In 60 patients, diaphragmatic excursion at rest in the supine position was 3.5 cm ± 1.2 on the right side and 3.5 cm ± 1.2 on the left side.
The maximum diaphragmatic excursion can be measured as the difference between the position of the diaphragm at functional residual capacity (FRC) and total lung capacity (TLC), while tidal excursion is the difference between the positional FRC and the end-inspiratory position during resting breathing. ... in the presence of marked abdominal ...
Complex experimental studies of hydrodynamically excited vibrations of fuel-element bundles have been performed on full-scale models of VVER-440 fuel assemblies. Pressure pulsations were used as the main hydrodynamic characteristic of turbulent coolant flow according to the effect of its load on the fuel-element surfaces in the flow. Realizations of the random hydrodynamic loads causing ...
In 1954, Elemash began to produce fuel assemblies, including for the first nuclear power plant in the world, located in Obninsk. In 1959, the facility produced the fuel for the Soviet Union's first icebreaker. Its fuel assembly production became serial in 1965 and automated in 1982. 1. Today, Elemash is one of the largest TVEL nuclear fuel ...
Geographic coordinates of Elektrostal, Moscow Oblast, Russia in WGS 84 coordinate system which is a standard in cartography, geodesy, and navigation, including Global Positioning System (GPS). Latitude of Elektrostal, longitude of Elektrostal, elevation above sea level of Elektrostal.
Several studies found that diaphragmatic dysfunction is due to gas insufflation in the abdominal cavity which might also be responsible for the increase of resistance and reduced diaphragmatic excursion, leading to reduced lung volume . All these factors lead to a change in postoperative lung function usually resulting in development of a ...
Choose district. and we'll show you all the information you need