Thank you for visiting nature.com. You are using a browser version with limited support for CSS. To obtain the best experience, we recommend you use a more up to date browser (or turn off compatibility mode in Internet Explorer). In the meantime, to ensure continued support, we are displaying the site without styles and JavaScript.
- View all journals
- My Account Login
- Explore content
- About the journal
- Publish with us
- Sign up for alerts
- Open access
- Published: 19 May 2021
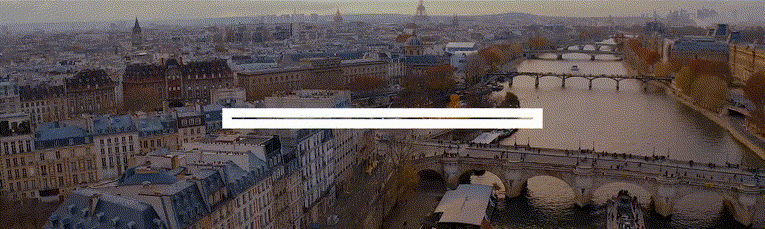
The kaolinite shuttle links the Great Oxidation and Lomagundi events
- Weiduo Hao ORCID: orcid.org/0000-0002-0486-424X 1 ,
- Kaarel Mänd ORCID: orcid.org/0000-0003-1575-3710 1 , 2 ,
- Yuhao Li 1 ,
- Daniel S. Alessi ORCID: orcid.org/0000-0002-8360-8251 1 ,
- Peeter Somelar 2 ,
- Mathieu Moussavou 3 ,
- Alexander E. Romashkin 4 ,
- Aivo Lepland 2 , 5 , 6 ,
- Kalle Kirsimäe 2 ,
- Noah J. Planavsky ORCID: orcid.org/0000-0001-5849-8508 7 &
- Kurt O. Konhauser 1
Nature Communications volume 12 , Article number: 2944 ( 2021 ) Cite this article
4545 Accesses
18 Citations
6 Altmetric
Metrics details
- Element cycles
- Geochemistry
The ~2.22–2.06 Ga Lomagundi Event was the longest positive carbon isotope excursion in Earth’s history and is commonly interpreted to reflect perturbations in continental weathering and the phosphorous cycle. Previous models have focused on mechanisms of increasing phosphorous solubilization during weathering without focusing on transport to the oceans and its dispersion in seawater. Building from new experimental results, here we report kaolinite readily absorbs phosphorous under acidic freshwater conditions, but quantitatively releases phosphorous under seawater conditions where it becomes bioavailable to phytoplankton. The strong likelihood of high weathering intensities and associated high kaolinite content in post-Great-Oxidation-Event paleosols suggests there would have been enhanced phosphorus shuttling from the continents into marine environments. A kaolinite phosphorous shuttle introduces the potential for nonlinearity in the fluxes of phosphorous to the oceans with increases in chemical weathering intensity.
Similar content being viewed by others

Rates of seafloor and continental weathering govern Phanerozoic marine phosphate levels
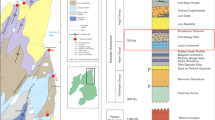
Transient fertilization of a post-Sturtian Snowball ocean margin with dissolved phosphate by clay minerals
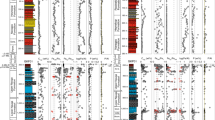
Earth’s Great Oxidation Event facilitated by the rise of sedimentary phosphorus recycling
Introduction.
The LE is the most pronounced and long-lived positive carbon isotope excursion in Earth history. Marine carbonate rocks deposited during the LE, which lasted at least 160 million years, are characterized by δ 13 C carb values >10 (refs. 1 , 2 , 3 , 4 ). The most widely accepted explanation for the LE relies on significant perturbation in the balance of different C burial fluxes—since organic carbon (C org ) is depleted in 13 C, an increase in C org burial compared to carbonate burial will result in 13 C-enrichment of the dissolved marine carbonate pool 2 . Although it is likely that at least some of the carbonate δ 13 C values reflect local, instead of global, processes (e.g., ref. 5 ), current explanations for the anomalously positive values require high rates of marine primary productivity. There is increasing consensus that P is likely to have been the ultimate limiting nutrient through Earth’s history 6 , 7 . Accordingly, explanations for the LE must center around the factors which can induce >100 myr perturbations to the global P cycle.
Oxidation of a reduced mineral enriched upper continental crust during the LE provides one possible mechanism to drive extended changes in the phosphorous cycle 8 , 9 . The proliferation of aerobic chemolithoautotrophy would have facilitated the rapid biological weathering of crustal sulfide minerals, leading to an extended episode of acid rock drainage, and solubilization of nutrient-bearing minerals 10 (e.g., apatite in rocks and soils, the weathering of which is highly pH-dependent 11 ). Similarly, extensive oxidation of siderite in aerially exposed sediments (e.g., shales, marls) during the LE would have led to high acid fluxes into soils and potentially promoted high atmospheric CO 2 levels, increasing weathering regimes more broadly 9 . Elevated siderite and pyrite contents in the weatherable shell during the LE were simply a consequence of higher fluxes of reduced S and Fe to marine sediments during the Archean (e.g., refs. 12 , 13 , 14 ). Upon weathering, P appears to have been mostly leached away and carried into the oceans, instead of retained in the paleosol (for instance, Hao et al. 15 report a peak in paleosol P loss at ~2.3–2.4 Ga). Kinetic and thermodynamic models further indicate that the weathering flux of P increased through the Archean, possibly reaching modern levels by the end of the eon 15 .
This driver of the LE assumes that higher acid fluxes will lead to greater P fluxes to the biosphere 8 , 10 . However, in the modern, typically <10% of total P is transported via rivers as dissolved anions (for detailed discussion see supplementary note 1). Most is transported in the particulate phase 16 , including P adsorbed onto Fe(III)-Al(III)-oxyhydroxides or clay minerals, or organically complexed 17 (see Table S1 ). A large portion of adsorbed P is then sedimented in estuaries and marginal marine environments with the flocculation of particulate phases where they remain inaccessible to plankton until remobilization within the sediment pile 18 . In the absence of plants, the only organic compounds delivered to the oceans would have been from degraded continental microbial mats 19 , which are unlikely to have had as large of an effect on the P cycle. Further, during the LE, ferric oxyhydroxides may have been solubilized in the generally sulfide and siderite-rich Paleoproterozoic soils, in a process similar to what is observed in some pyrite-rich black shale weathering environments today 20 . Building from this framework, it seems reasonable that clay minerals were an important vector by which P was delivered to the oceans at that time.
Different terrestrial weathering regimes will lead to the formation of widely varying secondary mineral assemblages—potentially leading to major changes in P sorption and transport to the oceans. In highly weathered soils today, kaolinite [Al 2 Si 2 O 5 (OH) 4 )], and its hydrated form halloysite [Al 2 Si 2 O 5 (OH) 4 ·2H 2 O], are often the only remaining clay mineral phases aside from Fe(III)- and Al(III)-oxyhydroxides and quartz 21 . With higher weathering intensities due to a stronger acid flux from pyrite and siderite oxidation, as well as higher CO 2 levels, kaolinite formation would have been widespread. This link is well developed in modern settings, with extensive kaolinite formation in soils developed on organic and pyrite-rich lithologies such as black shales 22 , 23 . Despite still constituting an important part of the clay fraction, clay minerals more characteristic of moderate terrestrial weathering conditions (e.g., smectites) are more rapidly dissolved in acidic environments 21 , 24 , 25 , 26 .
Conceptually, increased fluxes of kaolinite generation post-GOE are also expected due to an increase in continental area at 2.4 Ga 27 , 28 , 29 , and intensified continental weathering in the warm climate aftermath of Huronian global glaciation 30 , 31 , 32 . While goethite and hematite formation under an oxygenated atmosphere may have driven increased Al substitution into these phases at the expense of kaolinite formation 33 , 34 , we consider it unlikely to have substantially decreased kaolinite fluxes, as ferric hydroxide solubility increases dramatically with lower pH and Fe 3+ becomes the dominant pyrite oxidant in high acid production drainage environments 9 . Thus, in this work, we show the evidence of kaolinite generation in shales and paleosols post-GOE, and the capacity of kaolinite shuttling P from land to the oceans. The subsequent bacteria growth experiments demonstrate that the delivery of P by kaolinite leads to the augmentation of coastal primary productivity.
Kaolinite in post-GOE shales and paleosols
Consistent with the above view that an increase in kaolinite formation in the aftermath of the GOE, kaolinite is found in several LE successions. For example, the LST-12 drill core exposing the upper strata of the Francevillian Group in Gabon hosts organic-rich shales with sedimentary kaolinite alongside mixed-layer illite-smectite 35 (supplementary note 2 , and Figs. S1 – S3 ). Despite the greenschist facies metamorphic overprint 36 , tentative traces of preserved kaolinite are also found in X-ray diffraction spectra in two shale intervals of drill core OPH, which intersects the Zaonega Formation in Russia (supplementary note 2 and Fig. S1 ). There is also enhanced formation of kaolinite in paleosols formed around, and following, the GOE indicating intensive acidic continental weathering at that time 37 , 38 , 39 , 40 , 41 , 42 , 43 , 44 , 45 , 46 . However, it is important to note that it is not possible to estimate exactly how common kaolinite is from the rock record given that it is often transformed during burial metamorphism 47 . Nonetheless, clues about the extent of kaolinite formation and weathering intensity can be gleamed from the major elemental composition of paleosols. We examine Al-richness (compared to Ca, Na, Mg, and K) in a record of definitive, relatively well-preserved paleosols through the late Archean and early Proterozoic ( n = 10; see supplementary note 3 and Supplementary Data 1 ), since kaolinite is the main Al-bearing phase in highly weathered soils. Some Archean paleosols, such as Mt. Roe 45 (Fig. 1a ), display strong Al enrichment, suggesting strong weathering and local kaolinite production either under a high-CO 2 atmosphere during long period of weathering 39 , or in the presence of transient oxygenated conditions 45 . Notably, we observe that Al-richness peaks in soils formed between 2.4 Ga and 2.0 Ga (GOE and LE), compared to paleosols older or younger than this time bracket (Fig. 1a, b ), suggesting that kaolinite was highly abundant in contemporary terrestrial weathering environments. This trend is not likely an artifact of the differing latitude at which these paleosols formed given that calculated paleolatitudes do not correspond to the above trend (Supplementary Data 2 ).
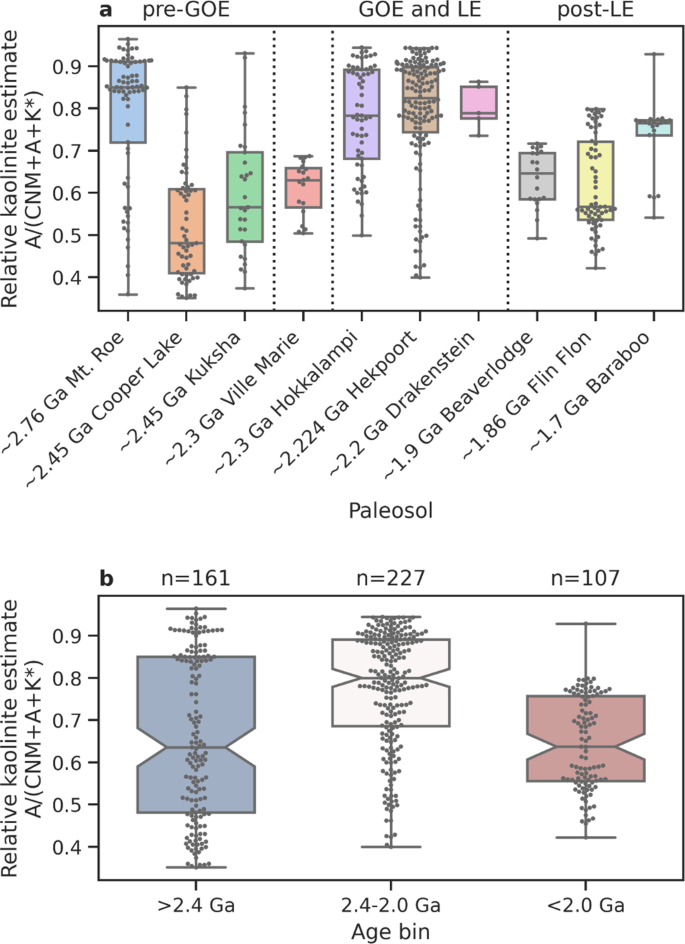
Relative kaolinite contents are approximated by molar Al-richness, as compared to Ca, Na, Mg, and K (CNM = CaO* + Na 2 O + MgO*; A = Al 2 O 3 ; K* = K 2 O*; asterisks denote data corrected for non-silicate contribution and K-metasomatism; Methods). a Al-richness in individual paleosols. The Ville Marie paleosol is poorly dated and may have formed either prior to or after the Great Oxidation Event (GOE), while its profile is possibly truncated with only the saprolite part preserved. Boxes show the mean value (horizontal line) and 25%/75% quantiles (edges), whiskers show the full range. Superimposed dots denote individual samples. b Al-richness in paleosols within the pre-GOE (>2.4 Ga), GOE and LJE (2.4–2.0 Ga), and post-LJE (>2.4 Ga) age bins.
The shuttling of P by kaolinite from rivers to the oceans
We used static and dynamic adsorption experiments to demonstrate that kaolinite will more effectively shuttle P to the oceans than other typical clay minerals or oxides/hydroxides. In the static experiments we simulated freshwater (pH 4 and ionic strength 0.01 M) to seawater-like (pH 8 and ionic strength 0.56 M) aqueous conditions, and also tested P adsorption to kaolinite at various initial phosphate concentrations to bracket modern riverine concentrations (Table S1 ). We stress that these conditions are meant to be representative of fresh and marine waters – they are not based on empirical- or model-based reconstruction of past pH values. For all initial P concentrations <400 ppb (12.9 μM), the difference between the equilibrium adsorption experiments of simulated freshwater and seawater was negligible (Fig. 2 ). By contrast, at initial P concentrations >400 ppb, there was ~100–200 ppb (3.2–6.4 μM) more P adsorbed in the freshwater environment than seawater. Surprisingly, ionic strength variations were found to be insignificant in modulating P adsorption compared to pH (similar trendlines for IS = 0.01 M and 0.56 M at the pH 8 conditions; Fig. 2 ). However, the surface charge of kaolinite is pH-dependent 48 , 49 . Surface complexation modeling based on acid-base titrations of kaolinite shows that kaolinite surface functional groups are highly protonated at pH < 4.7, lending the clay a net positive charge (supplementary note 4), which can electrostatically attract negatively charged P species (dominantly H 2 PO 4 − at pH 2–7). A bidentate mononuclear surface complex of P onto positively charged sites is the dominant adsorption mechanism on kaolinite, explaining the pH-dependence of P adsorption (supplementary note 4).
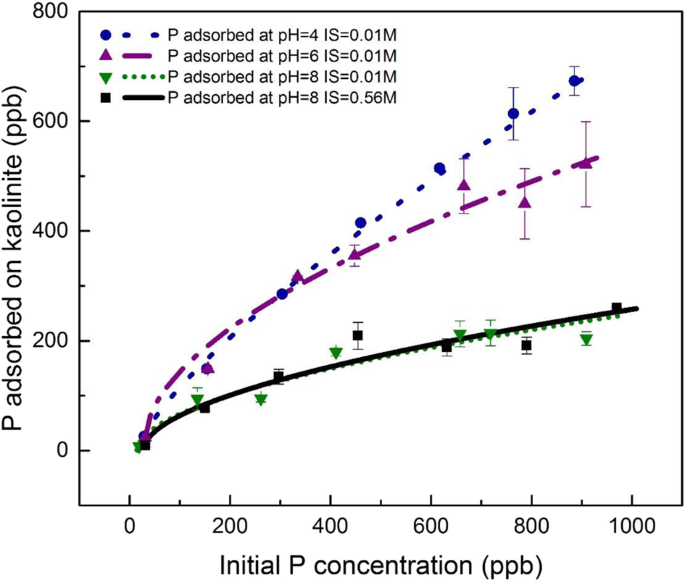
Black squares and continuous line represent P adsorption at pH 8, IS = 0.56 M conditions; green downward-facing triangles and dotted line represent P adsorption at pH 8, IS = 0.01 M conditions; purple upward-facing triangles and dashed-dotted line show P adsorption at pH 6, IS = 0.01 M conditions; blue circles and dashed line are P adsorption at pH 4, IS = 0.01 M conditions. Error bars represent the ±1 standard deviation of replicates.
To compare the results to clays more typical of moderate weathering regimes, we also examined the P adsorption capacities of illite and montmorillonite in both freshwater and marine conditions (data shown in supplementary note 5). We show that even under lower degrees of weathering characterized by montmorillonite and illite production, the transport of clays from rivers to the oceans can act as a P source simply due to variations in aqueous conditions. Importantly, however, the magnitude of P release is depressed when compared to kaolinite, suggesting that the kaolinite shuttle is more conducive to transporting P from land to the sea.
Dynamic adsorption experiments were performed to directly observe P behavior on kaolinite surfaces under simultaneously changing pH and ionic strength conditions (Fig. 3 ). At a P concentration of ~155 ppb (5 μM), 95% of the P was adsorbed onto kaolinite surfaces at shale weathering conditions (pH 4). After switching to marine conditions (pH of 8), the proportion of adsorbed P steadily declined throughout our experimental period (3 days). In total, 20% of pre-adsorbed P was released into the aqueous environment. As a comparison, we also analyzed P sorption to Fe(III)-oxides and Al(III)-hydroxide, such as hematite (Fe 2 O 3 ) and gibbsite (Al[OH] 3 ), respectively, as both minerals represent stable mineral oxide phases under extreme weathering environments (e.g., laterites 23 , 50 ). For both hematite and gibbsite, we show 100% adsorption of P at freshwater conditions but negligible release at seawater pH and ionic strength (Fig. 3 ).
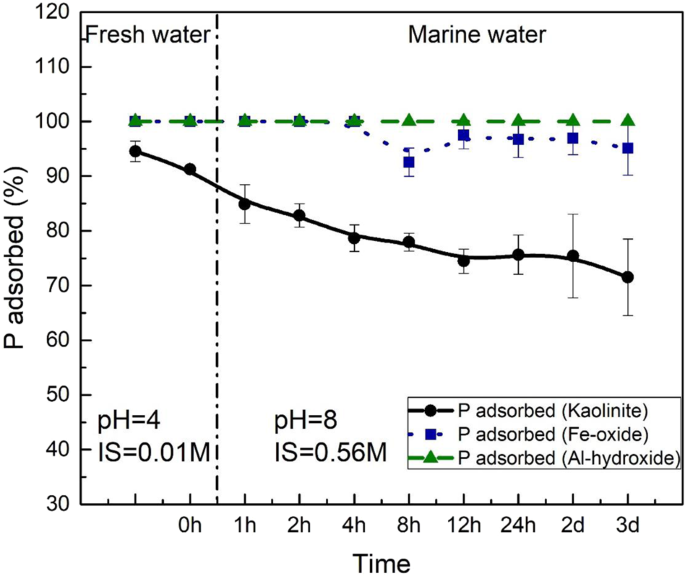
Freshwater conditions were simulated at pH 4 and ionic strength = 0.01 M, while marine conditions refer to pH 8 and ionic strength = 0.56 M. The black circles and continuous line represent P desorption from kaolinite surfaces; blue squares and dotted line show the P desorption from Fe-oxide; green triangles and dashed line show P desorption from Al-hydroxide. Error bars represent the ±1 standard deviation of replicates.
Augmentation of nearshore primary bioproductivity
To confirm that the desorbed P in our experiments will be truly bioavailable, we also explored the impact of P bearing kaolinite input to the growth of the marine cyanobacterium Synechococcus sp. PCC 7002 in continuous culture; this species comprises a significant fraction of the modern marine phytoplankton 51 . Without clay input, cell density reached a plateau at the 2nd day and then decreased to 5 × 10 6 cells/mL (55% of initial density) at the 14th day, while with 1 g/L of P-bearing clay input, the cell number reached 8 × 10 6 cells/mL (97% of initial density) at the end of the growth period. A 2 g/L P-bearing kaolinite input led to a bacterial cell density of 1.2 × 10 7 (139% of initial density), more than twice that of the blank (Fig. 4 ). In summary, the high adsorption capacity of kaolinite under freshwater conditions and its low capacity under marine conditions results in enhanced P bioavailability and cyanobacterial productivity in post-GOE nearshore environments.
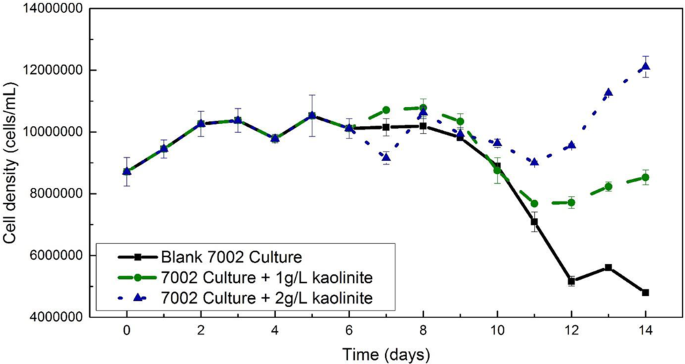
The original culture was divided into three 150 mL sub-cultures after day 6. Black squares and straight line represent the control culture incubated in modified A + growth media with only 5 µM potassium phosphate. The green circles and dashed line show the growth of a culture with addition of 150 mg of P-bearing kaolinite after day 6. The blue triangles and dotted line represent the culture in which 300 mg of P-bearing kaolinite was added after day 6. Error bars represent the ±0.5 standard deviation of replicates.
It should still be noted that kaolinite shuttling would not have been the sole process providing bioavailable P to marine primary producers after the GOE. For example, although P release from Fe(III)- and Al(III)-hydroxides is not as efficient as from kaolinite, bound P can be released from sediments to the water column over longer periods during early diagenesis 52 . Additionally, since Paleoproterozoic oceans were likely redox-stratified, some ferric oxyhydroxides would have been reductively dissolved below the redoxcline, and the released P made bioavailable through upwelling 53 . Smectite (montmorillonite) and illite, though showing a relatively lower P shuttling capacity compared to kaolinite (supplementary note 5), may also have contributed to nutrient shuttling during the Lomagundi Event, as, again, suggested by the preservation of smectite-rich clay minerals in Francevillian black shales 35 , 54 and major elemental compositions close to average smectite composition in contemporary paleosols (Fig. S3 ). It is also worth noting that any changes in terrestrial P inputs are going to be modulated by the marine P cycle. For instance, coupled P and Fe enrichment in the Francevillian basin, which was likely influenced by upwelling (e.g., refs. 55 , 56 ), is likely a signal of Fe control on marine P cycling. Nonetheless, we highlight that the kaolinite shuttle added upon, and compounded, these prior P-fertilization mechanisms to significantly increase the supply of bioavailable P to nearshore ecosystems.
It is also possible that the kaolinite shuttle was turned on during other extreme global carbon cycle and biogeochemical perturbations in Earth’s past marked by anomalously high primary productivity (e.g., the Permian Triassic biotic crisis; Paleocene-Eocene Thermal Maximum (PETM)). For example, the PETM is characterized by a rise of surface temperature (5–8 °C) and extensive continental weathering (e.g., ref. 57 ). Consistent with our view, there are spikes in kaolinite formation during the PETM in several regions 58 , 59 . A compilation of marine sediments from the P-E boundary demonstrates a dramatic increase in kaolinite generation (approaching 80% of kaolinite in clay phases) during the PETM period (supplementary note 6, Supplementary Data 3 , and Fig. S11 ). The shuttle of kaolinite from land to the oceans would have increased marine bio-productivity, induced the storage of CO 2 into 13 C-depleted organic carbon, and subsequently recover the post-PETM δ 13 C carb to Paleocene values (see ref. 57 ).
The kaolinite shuttle provides a novel mechanism to tie together known threads of Earth’s biogeochemical environment following the initial rise of atmospheric oxygen – increased acid weathering and enhanced primary productivity. A critical aspect of the model is that the response of the P cycle to weathering intensity is non-linear. Phosphorous fluxes became greatly enhanced when intense terrestrial weathering conditions that produced abundant kaolinite became spatially widespread. Strong nonlinearity in the extent of P shuttling could help explain why the Lomagundi stands out as a unique event in Earth’s history. Reconstructing mineralogical trends in deep time events and from Lomagundi-aged sediments is difficult given clay transformation with burial, but more recent “weathering events” (e.g., the PETM) bolster the case that there is a link between terrestrial weathering regimes, nutrient fluxes, intervals of anoxia, extinction, and ecosystem transitions.
The paleosol chemical database of eight relatively complete paleosols ranging in age from 2.76 Ga to 1.70 Ga was compiled from published paleosol studies and used to assess trends in weathering intensity through the time period. Al, Ca, Na, Mg, and K were plotted on a CNM-A-K ternary plot, modified from the CN-A-K plot of Nesbitt and Young 30 . The addition of M (Mg) was to separate Al-rich kaolinite/gibbsite – proxies for acid weathering – from Al and Mg-rich chlorite, which is a common diagenetic-metamorphic mineral. The paleosol database was scrubbed of samples representing cover rocks and soils developed on chemical sediments. Ca and Mg were corrected for carbonate and apatite content, and K for K-metasomatism. For the detailed methodology, see supplementary note 3. The mineral composition (Fig. S1 ) of mudstones in drill core LST-12 (Francevillian Group, Gabon) and OPH (Zaonega Formation, Russia) was determined by X-ray diffractometry using a Bruker D8 Advance diffractometer with Cu K α radiation and a LynxEye positive sensitive detector. The measured patterns were interpreted and modeled using the Rietveld algorithm-based program Topaz. The relative error of quantification is better than 10% for major phases (>5 wt%) and better than 20% for minor phases (<5 wt%). For the geological setting, location and description of the successions see ref. 60 for core LST-12, and refs. 61 , 62 , 63 for the OPH core.
Phosphate equilibrium adsorption experiments onto three clay minerals (kaolinite, illite, and montmorillonite) were performed at the following phosphate concentrations: 1, 5, 10, 15, 20, 25, and 30 μM. A 5-mM Na 2 HPO 4 stock solution was prepared by dissolving Na 2 HPO 4 salt (ACS certified, Fisher Scientific) into MQ water. Solutions of different phosphate concentration were prepared by diluting the stock solution with a 0.01-M NaCl or 0.56-M NaCl solution to a total volume of 50 ml. Then, 5 ml of solution was pipetted to analyze the initial phosphate concentration. After that, 45 mg of clay was added into solution to make a 1-g/L solid suspension. After agitation, the solution pH of each experiment was adjusted to 4, 6, and 8 separately using 0.1 M HCl and 0.1 M NaOH. The solution pH was consistently maintained during the adsorption period. Once equilibrium was reached, 5 ml of clay suspension was pipetted and filtered through a 0.2-µm filter. The filtrate and the initial 5 ml solution were acidified for ICP-MS analysis. All experiments were performed in duplicate.
Phosphate dynamic adsorption experiments were performed in a 500-ml beaker. For simulation of freshwater conditions, 400 ml of 0.01 M NaCl solution was added to a glass beaker. Then, 0.4 mL of 5 mM Na 2 HPO 4 stock solution was added to make a 5-μM phosphate solution. A magnetic stir bar was added to keep the solution mixing during the whole experimental period. Once well mixed, a 5-ml aliquot was taken for initial phosphate concentration analysis. After, the solution pH was adjusted to 4 with small aliquots of 0.1 M HCl and 0.1 M NaOH, mimicking freshwater conditions, and 0.395 g of kaolinite was added into the beaker to make a 1 g/L clay suspension. The adsorption of phosphate onto clays under the freshwater condition lasted 24 h and during this process, the pH was maintained at 4 using 0.1 M HCl and 0.1 M NaOH solution. After 24 h, a 5-ml aliquot was syringed out and filtered through a 0.2 µm filter for ICP-MS analysis. Then, the solution pH was adjusted to 8 by a 0.1-M NaOH solution and 12.87 g of NaCl was added into the beaker at the same time to adjust the ionic strength to 0.56 M, representing marine water conditions. Once the pH stabilized, a 5-ml aliquot was collected for phosphate concentration analysis at t = 0. The whole marine condition adsorption process took 2 days and the time intervals of sample collection were t = 0, t = 1 h, t = 2 h, t = 4 h, t = 8 h, t = 12 h, t = 1 d, and t = 2 d. During the experiment, the reaction beaker was covered by parafilm to prevent possible solution evaporation. Same procedure was applied to Al-hydroxide and Fe-oxide. The phosphate concentration in these samples was analyzed by ICP-MS. Detailed experimental information could also be found in Hai et al. 64 .
The cyanobacterium Synechococcus sp. PCC7002 was used as an analog strain in the growth experiment to analyze its response to the addition of P-bearing kaolinite clay. This axenic strain was incubated in a modified A + growth media with a limited phosphate concentration of 5 µM. All cultures were grown on a shaker in a designated growth chamber that was set at 30 °C with constant halogen lighting. A sterile 1-L Erlenmeyer flask with 550 mL of modified A+ growth media was initially inoculated from an axenic PCC 7002 culture growing on an agar plate. The growth of the microbial culture was monitored by optical density at 750 nm (OD 750 nm) on a daily basis after inoculation. The growth curve started at day 0 when the OD 750 nm absorbance was above 0.15. A triplicate of 1 mL cultures was extracted sterilely from the flask each day for Chlorophyll-a concentration analysis as an indicator of cell growth from day 0. When it was certain that the culture reached the stationary phase, at which time the Chlorophyll-a concentration stopped increasing at ~0.680 µg/mL, the culture was further divided into three 150 mL sub-cultures in 500 mL Erlenmeyer flasks. Immediately after sub-culturing, 150 mg and 300 mg of P-bearing kaolinite were added into two of the three sub-cultures to make a 1 g/L and 2 g/L kaolinite suspension, respectively. The last sub-culture was the control where no clay was added. All three cultures were continuously examined for cell growth by Chlorophyll-a concentration until day 14, when there was a clear cell density difference among three sub-cultures (Fig. 4 ). Both optical density at 750 nm and chlorophyll-a concentration was measured on a Beckman Coulter DU®520 UV/VIS spectrophotometer. The chlorophyll-a concentration was lastly converted to cell density using a correlation that was established from a standard growth curve, which was systematically quantified by both chlorophyll-a concentration and cell density that was measured on an Attune NXT acoustic focusing flow cytometer (Fig. 4 ).
Data availability
The authors declare that the main data supporting the findings of this study are available in the following Data Repository link: https://doi.org/10.17632/dmhfrympsr.1 .
Code availability
Code available at https://doi.org/10.5281/zenodo.4641971 .
Bekker, A., Karhu, J. A., Eriksson, K. A. & Kaufman, A. J. Chemostratigraphy of Paleoproterozoic carbonate successions of the Wyoming Craton: tectonic forcing of biogeochemical change? Precambrian Res. 120 , 279–325 (2003).
Article ADS CAS Google Scholar
Karhu, J. A. & Holland, H. D. Carbon isotopes and the rise of atmospheric oxygen. Geology 24 , 867–870 (1996).
Schidlowski, M., Eichmann, R. & Junge, C. E. Carbon isotope geochemistry of Precambrian Lomagundi carbonate province, Rhodesia. Geochim. Cosmochim. Acta 40 , 449–455 (1976).
Melezhik, V. A., Fallick, A. E., Medvedev, P. V. & Makarikhin, V. V. Extreme C-13Ccarb enrichment in ca, 2.0 Ga magnesite-stromatolite-dolomite-‘red beds’ association in a global context: a case for the world-wide signal enhanced by a local environment. Earth Sci. Rev. 48 , 71–120 (1999).
Geyman, E. C. & Maloof, A. C. A diurnal carbon engine explains 13C-enriched carbonates without increasing the global production of oxygen. Proc. Natl Acad. Sci. USA 116 , 24433–24439 (2019).
Article ADS CAS PubMed PubMed Central Google Scholar
Reinhard, C. T. et al. Evolution of the global phosphorus cycle. Nature 541 , 386–389 (2017).
Article ADS CAS PubMed Google Scholar
Laakso, T. A. & Schrag, D. P. Limitations on limitation. Glob. Biogeochem. Cycles 32 , 486–496 (2018).
Bekker, A. & Holland, H. D. Oxygen overshoot and recovery during the early Paleoproterozoic. Earth Planet. Sci. Lett. 317 , 295–304 (2012).
Bachan, A. & Kump, L. R. The rise of oxygen and siderite oxidation during the Lomagundi Event. Proc. Natl Acad. Sci. USA 112 , 6562–6567 (2015).
Konhauser, K. O. et al. Aerobic bacterial pyrite oxidation and acid rock drainage during the Great Oxidation Event. Nature 478 , 369–373 (2011).
Smith, E. A., Mayfield, C. I. & Wong, P. T. S. Physical and chemical characterization of selected natural apatites in synthetic and natural aqueous solutions. Water Air Soil Pollut. 8 , 401–415 (1977).
Article CAS Google Scholar
Rosas, J. C. & Korenaga, J. Rapid crustal growth and efficient crustal recycling in the early Earth: implications for Hadean and Archean geodynamics. Earth Planet. Sci. Lett. 494 , 42–49 (2018).
Kump, L. R. & Seyfried, W. E. Hydrothermal Fe fluxes during the Precambrian: effect of low oceanic sulfate concentrations and low hydrostatic pressure on the composition of black smokers. Earth Planet. Sci. Lett. 235 , 654–662 (2005).
Kump, L. R. & Barley, M. E. Increased subaerial volcanism and the rise of atmospheric oxygen 2.5 billion years ago. Nature 448 , 1033–1036 (2007).
Hao, J., Knoll, A. H., Huang, F., Hazen, R. M. & Daniel, I. Cycling phosphorus on the Archean Earth: Part I. Continental weathering and riverine transport of phosphorus. Geochim. Cosmochim. Acta 273 , 70–84 (2020).
Froelich, P. N. Kinetic control of dissolved phosphate in natural rivers and estuaries: a primer on the phosphate buffer mechanism 1. Limnol. Oceanogr. 33 , 649–668 (1988).
ADS CAS Google Scholar
Gérard, F. Clay minerals, iron/aluminum oxides, and their contribution to phosphate sorption in soils - A myth revisited. Geoderma 262 , 213–226 (2016).
Conley, D. J., Smith, W. M., Cornwell, J. C. & Fisher, T. R. Transformation of particle-bound phosphorus at the land-sea interface. Estuar. Coast. Shelf Sci. 40 , 161–176 (1995).
Lalonde, S. V. & Konhauser, K. O. Benthic perspective on Earth’s oldest evidence for oxygenic photosynthesis. Proc. Natl Acad. Sci. USA 112 , 995–1000 (2015).
Joeckel, R. M., Ang Clement, B. J. & VanFleet Bates, L. R. Sulfate-mineral crusts from pyrite weathering and acid rock drainage in the Dakota Formation and Graneros Shale, Jefferson County, Nebraska. Chem. Geol. 215 , 433–452 (2005).
Velde, B. B. & Meunier, A. The Origin Of Clay Minerals In Soils And Weathered Rocks . p. 219–226 (Springer-Verlag, 2008).
Fischer, C., Schmidt, C., Bauer, A., Gaupp, R. & Heide, K. Mineralogical and geochemical alteration of low-grade metamorphic black slates due to oxidative weathering. Geochemistry 69 , 127–142 (2009).
Jin, L. et al. Evolution of porosity and geochemistry in Marcellus Formation black shale during weathering. Chem. Geol. 356 , 50–63 (2013).
Bibi, I., Singh, B. & Silvester, E. Dissolution of illite in saline-acidic solutions at 25 °C. Geochim. Cosmochim. Acta 75 , 3237–3249 (2011).
Bibi, I., Singh, B. & Silvester, E. Dissolution kinetics of soil clays in sulfuric acid solutions: Ionic strength and temperature effects. Appl. Geochem. 51 , 170–183 (2014).
Hao, W. et al. Effect of acidic conditions on surface properties and metal binding capacity of clay minerals. ACS Earth Space Chem. 3 , 2421–2429 (2019).
Lee, C.-T. A. et al. Two-step rise of atmospheric oxygen linked to the growth of continents. Nat. Geosci. 9 , 417–424 (2016).
Korenega, J., Planavsky, N. J. & Evans, D. A. D. Global water cycle and the coevolution of the Earth’s interior and surface environment. Philos. Trans. R. Soc. Ser. A 375 , 20150393 (2017).
Guo, M. & Korenaga, J. Argon constraints on the early growth of felsic continental crust. Sci. Adv. 6 , eaaz6234 (2020).
Nesbitt, H. W. & Young, G. Early Proterozoic climates and plate motions inferred from major element chemistry of lutites. Nature 299 , 715 (1982).
Papineau, D., Mojzsis, S. J. & Schmitt, A. K. Multiple sulfur isotopes from Paleoproterozoic Huronian interglacial sediments and the rise of atmospheric oxygen. Earth Planet. Sci. Lett. 255 , 188–212 (2007).
Tang, H. & Chen, Y. Global glaciations and atmospheric change at ca. 2.3 Ga. Geosci. Front. 4 , 583–596 (2013).
Trolard, F. & Tardy, Y. The stabilities of gibbsite, boehmite, aluminous goethites and aluminous hematites in bauxites, ferricretes and laterites as a function of water activity, temperature and particle size. Geochim. Cosmochim. Acta 51 , 945–957 (1987).
Trolard, F. & Tardy, Y. A model of Fe 3+ -kaolinite, Al 3+ -goethite, Al 3+ -hematite equilibria in laterites. Clay Miner. 24 , 1–21 (1989).
Ossa, F. O. et al. Exceptional preservation of expandable clay minerals in the ca. 2.1 Ga black shales of the Francevillian basin, Gabon and its implication for atmospheric oxygen accumulation. Chem. Geol. 362 , 181–192 (2013).
Melezhik, V. A., Fallick, A. E., Filippov, M. M. & Larsen, O. Karelian shungite - an indication of 2.0-Ga-old metamorphosed oil-shale and generation of petroleum: geology, lithology and geochemistry. Earth Sci. Rev. 47 , 1–40 (1999).
Bandopadhyay, P., Eriksson, P. & Roberts, R. A vertic paleosol at the Archean-Proterozoic contact from the Singhbhum-Orissa craton, eastern India. Precambrian Res. 177 , 277–290 (2010).
de Wall, H., Pandit, M. K. & Chauhan, N. K. Paleosol occurrences along the Archean–Proterozoic contact in the Aravalli craton, NW India. Precambrian Res. 216 , 120–131 (2012).
Soomer, S. et al. High-CO 2 , acidic and oxygen-starved weathering at the Fennoscandian Shield at the Archean-Proterozoic transition. Precambrian Res. 327 , 68–80 (2019).
Retallack, G. J. & Krinsley, D. H. Metamorphic alteration of a Precambrian (2.2 Ga) paleosol from South Africa revealed by backscattered electron imaging. Precambrian Res. 63 , 27–41 (1993).
Article ADS Google Scholar
Gutzmer, J. & Beukes, N. J. Earliest laterites and possible evidence for terrestrial vegetation in the Early Proterozoic. Geology 26 , 263–266 (1998).
Gall, Q. The Proterozoic Thelon paleosol, Northwest Territories, Canada. Precambrian Res. 68 , 115–137 (1994).
Rye, R. & Holland, H. D. Paleosols and the evolution of atmospheric oxygen: a critical review. Am. J. Sci. 298 , 621–672 (1998).
Nedachi, Y., Nedachi, M., Bennett, G. & Ohmoto, H. Geochemistry and mineralogy of the 2.45 Ga Pronto paleosols, Ontario, Canada. Chem. Geol. 214 , 21–44 (2005).
Teitler, Y. et al. Ubiquitous occurrence of basaltic-derived paleosols in the Late Archean Fortescue Group, Western Australia. Precambrian Res. 267 , 1–27 (2015).
Marmo, J. S. The Lower Proterozoic Hokkalampi Paleosol In North Karelia, Eastern Finland . p. 41–66 (Springer, 1992).
Fedo, C. M., Wayne Nesbitt, H. & Young, G. M. Unraveling the effects of potassium metasomatism in sedimentary rocks and paleosols, with implications for paleoweathering conditions and provenance. Geology 23 , 921–924 (1995).
Hao, W., Flynn, S. L., Alessi, D. S. & Konhauser, K. O. Change of the point of zero net proton charge (pH PZNPC ) of clay minerals with ionic strength. Chem. Geol. 493 , 458–467 (2018).
Hao, W. et al. The impact of ionic strength on the proton reactivity of clay minerals. Chem. Geol. 529 , 119294 (2019).
Teitler, Y. et al. Petrology and geochemistry of scandium in New Caledonian Ni-Co laterites. J. Geochem. Explor. 196 , 131–155 (2019).
Flombaum, P. et al. Present and future global distributions of the marine Cyanobacteria Prochlorococcus and Synechococcus . Proc. Natl Acad. Sci. USA 110 , 9824–9829 (2013).
Sundby, B., Gobeil, C., Silverberg, N. & Mucci, A. The phosphorus cycle in coastal marine sediments. Hydrobiologia 37 , 1129–1145 (1993).
Google Scholar
Konhauser, K. O. et al. Phytoplankton contributions to the trace-element composition of Precambrian banded iron formations. Geol. Soc. Am. Bull. 130 , 941–951 (2018).
Albani, A. et al. Large colonial organisms with coordinated growth in oxygenated environments 2.1 Gyr ago. Nature 466 , 100–104 (2010).
Article ADS PubMed CAS Google Scholar
Gauthier-Lafaye, F. & Weber, F. Natural nuclear fission reactors: time constraints for occurrence, and their relation to uranium and manganese deposits and to the evolution of the atmosphere. Precambrian Res. 120 , 81–100 (2003).
Mayika, K. B. et al. The Paleoproterozoic Francevillian succession of Gabon and the Lomagundi-Jatuli event. Geology 48 , 1099–1104 (2020).
McInerney, F. A. & Wing, S. L. The Paleocene-Eocene Thermal Maximum: a perturbation of carbon cycle, climate, and biosphere with implications for the future. Annu. Rev. Earth Planet. Sci. 39 , 489–516 (2011).
Dypvik, H. et al. The Paleocene–Eocene thermal maximum (PETM) in Svalbard - clay mineral and geochemical signals. Palaeogeogr. Palaeoclimatol. Palaeoecol. 302 , 156–169 (2011).
Article Google Scholar
Pujalte, V., Baceta, J. & Schmitz, B. A massive input of coarse-grained siliciclastics in the Pyrenean Basin during the PETM: the missing ingredient in a coeval abrupt change in hydrological regime. Clim. Past 11 , 1653–1672 (2015).
Ossa, F. O. et al. Two-step deoxygenation at the end of the Paleoproterozoic Lomagundi Event. Earth Planet. Sci. Lett. 486 , 70–83 (2018).
Melezhik, V. A., Fallick, A. E., Brasier, A. T. & Lepland, A. Carbonate deposition in the Palaeoproterozoic Onega basin from Fennoscandia: a spotlight on the transition from the Lomagundi-Jatuli to Shunga events. Earth Sci. Rev. 147 , 65–98 (2015).
Blättler, C. et al. Two-billion-year-old evaporites capture Earth’s great oxidation. Science 360 , 320–323 (2018).
Article PubMed CAS Google Scholar
Kreitsmann, T. et al. Hydrothermal dedolomitisation of carbonate rocks of the Paleoproterozoic Zaonega Formation, NW Russia—Implications for the preservation of primary C isotope signals. Chem. Geol. 512 , 43–57 (2019).
Hao, W. et al. Clay minerals as a source of cadmium to estuaries. Sci. Rep. 10 , 10417 (2020).
Download references
Acknowledgements
K.M. was supported by the Ministry of Education and Research of Estonia (Kristjan Jaak Scholarship program). K.M. and K.K. received further support from the Estonian Research Council grant PRG447 and P.S. from PUT1511. N.J.P acknowledges support from NASA Astrobiology Institute under Cooperative Agreement No. NNA15BB03A issued through the Science Mission Directorate. This work was supported by National Science and Engineering Research Council of Canada (NSERC) Discovery Grants to K.O.K. (RGPIN-165831) and D.S.A. (RGPIN-04134).
Author information
Authors and affiliations.
Department of Earth and Atmospheric Sciences, University of Alberta, Edmonton, Canada
Weiduo Hao, Kaarel Mänd, Yuhao Li, Daniel S. Alessi & Kurt O. Konhauser
Department of Geology, University of Tartu, Tartu, Estonia
Kaarel Mänd, Peeter Somelar, Aivo Lepland & Kalle Kirsimäe
Department of Geology, University of Science and Technology of Masuku, Franceville, Gabon
Mathieu Moussavou
Institute of Geology, Karelian Science Centre, Petrozavodsk, Russia
Alexander E. Romashkin
CAGE—Centre for Arctic Gas Hydrate, Environment and Climate, Department of Geosciences, UiT The Arctic University of Norway, Tromsø, Norway
Aivo Lepland
Geological Survey of Norway (NGU), Trondheim, Norway
The Department of Earth and Planetary Sciences, Yale University, New Haven, CT, USA
Noah J. Planavsky
You can also search for this author in PubMed Google Scholar
Contributions
K.O.K. and W.H. conceived the study; W.H. performed all the P equilibrium adsorption experiments, pH edges, and dynamic adsorption experiments; W.H. and D.S.A. performed the surface complexation modeling of the adsorption data. W.H. compiled the database for P distribution in suspended sediments of modern river; K.M., P.S., M.M., A.E.R., A.L., and K.K. provided information for the Francevillian Group and the Zaonega Formation, and the database for paleosol. Y.L. conducted the bacteria growth curve experiments. W.H., K.M. K.O.K., and N.J.P. wrote the manuscript with input from all co-authors.
Corresponding author
Correspondence to Weiduo Hao .
Ethics declarations
Competing interests.
The authors declare no competing interests.
Additional information
Peer review information Nature Communications thanks Frantz Ossa Ossa and Yoram Teitler for their contribution to the peer review of this work.
Publisher’s note Springer Nature remains neutral with regard to jurisdictional claims in published maps and institutional affiliations.
Supplementary information
Supplementary information, description of additional supplementary files, supplementary data 1, supplementary data 2, supplementary data 3, rights and permissions.
Open Access This article is licensed under a Creative Commons Attribution 4.0 International License, which permits use, sharing, adaptation, distribution and reproduction in any medium or format, as long as you give appropriate credit to the original author(s) and the source, provide a link to the Creative Commons license, and indicate if changes were made. The images or other third party material in this article are included in the article’s Creative Commons license, unless indicated otherwise in a credit line to the material. If material is not included in the article’s Creative Commons license and your intended use is not permitted by statutory regulation or exceeds the permitted use, you will need to obtain permission directly from the copyright holder. To view a copy of this license, visit http://creativecommons.org/licenses/by/4.0/ .
Reprints and permissions
About this article
Cite this article.
Hao, W., Mänd, K., Li, Y. et al. The kaolinite shuttle links the Great Oxidation and Lomagundi events. Nat Commun 12 , 2944 (2021). https://doi.org/10.1038/s41467-021-23304-8
Download citation
Received : 28 October 2020
Accepted : 19 April 2021
Published : 19 May 2021
DOI : https://doi.org/10.1038/s41467-021-23304-8
Share this article
Anyone you share the following link with will be able to read this content:
Sorry, a shareable link is not currently available for this article.
Provided by the Springer Nature SharedIt content-sharing initiative
This article is cited by
- Ernest Chi Fru
- Jalila Al Bahri
- Timothy W. Lyons
Nature Communications (2023)
By submitting a comment you agree to abide by our Terms and Community Guidelines . If you find something abusive or that does not comply with our terms or guidelines please flag it as inappropriate.
The Termination and Aftermath of the Lomagundi-Jatuli Carbon Isotope Excursions in the Paleoproterozoic Hutuo Group, North China
Doi: 10.1007/s12583-015-0654-4.
- Zhenbing She 1, 2 , , ,
- Fanyan Yang 3 ,
- Wei Liu 4 ,
- Luhua Xie 5, 6 ,
- Yusheng Wan 7 ,
- Chao Li 1 ,
- Dominic Papineau 8, 9
State Key Laboratory of Biogeology and Environmental Geology, China University of Geosciences, Wuhan 430074, China
School of Earth Sciences, China University of Geosciences, Wuhan 430074, China
Ningxia Geology And Mineral Resources Centre Lab, Yinchuan 750021, China
Tianjin Research Institute for Water Transport Engineering, M. O. T., Tianjin 300456, China
State Key Laboratory of Isotope Geochemistry, Guangzhou Institute of Geochemistry, Chinese Academy of Sciences, Guangzhou 510640, China
Key Laboratory of Marginal Sea Geology, Guangzhou Institute of Geochemistry, Chinese Academy of Sciences, Guangzhou 510640, China
Beijing SHRIMP Center, Institute of Geology, Chinese Academy of Geological Sciences, Beijing 100037, China
London Centre for Nanotechnology, University College London, London
Department of Earth Sciences, University College London, 17-19 Gower Street, London, United Kingdom
- Corresponding author: Zhenbing She, [email protected]
- Accepted Date: 17 Sep 2015
- Publish Date: 01 Apr 2016
- Paleoproterozoic ,
- Lomagundi-Jatuli event ,
- Hutuo Group ,
- carbon isotope ,
Proportional views
通讯作者: 陈斌, [email protected].
沈阳化工大学材料科学与工程学院 沈阳 110142
Figures( 7 ) / Tables( 1 )
Article Metrics
- PDF Downloads( 411 )
- Abstract views( 816 )
- HTML views( 187 )
Copyright © 2013-2020 Journal of Earth Science 鄂ICP备15021562号-2
Tel: +86-27-67885075 Fax: +86-27-67885075 E-mail: [email protected]
Address: Editorial Office of Journal, China University of Geosciences, Yujiashan, Wuhan, Hubei 430074, P. R. China
Export File
- Figure 1. (a) Tectonic divisions of the North China Craton highlighting exposed Archean and Paleoproterozoic rocks (modified after Zhao et al., 1999 ). (b) Simplified geological map of the Wutai area (modified after Bai, 1986 ).
- Figure 2. Generalized stratigraphic column of the Hutuo Group (modified after Bai, 1986 ) with correlation to Paleoproterozoic successions in N Henan (Lai et al., 2011) and E Liaoning provinces ( Tang et al., 2011 ). Previously published age dates and C isotope data are shown.
- Figure 3. Sedimentary structures. (a) Herringbone cross-bedding, Wenshan Fm. (b) Ripple marks, Wenshan Fm. (c) Disturbed beds (black arrows), rounded intraclasts and flat pebbles (white arrows), Hebiancun Fm. (d) Convolute bedding, Qingshicun Fm. (e) Digitate stromatolite, Jianancun Fm. (f) Stromatolite bioherms, Jianancun Fm. (g) Stromatolite bioherms, Dashiling Fm. (h) Purple-colored wavy-Conical stromatolites, Wenshan Fm. (i) Oblique section of tightly-packed stromatolites, Daguandong Fm. (j) Cherty dolostone with alternating purplish chert layers (ch) and white dolomicrite layers (dol) indicating silicification during very early diagenetic stage, Hebiancun Fm. Hammers are ~40 cm long, and marker pen is 14 cm long.
- Figure 4. Petrographic features of the Hutuo carbonates. (a) Slab of an orange-colored massive dolostone micro-drilled for C isotope analysis, arrow showing the drill pit; (b) pure microcrystalline dolostone with crystals generally smaller than 15 µm; (c) primary laminae in stromatolitic dolostone; (d) magnified view of (c) showing dolomite microcrystals and clots of carbonaceous material; (e) alternated dark organic-rich and transparent dolomite laminae in a stromatolite; (f) magnified view of (e) showing the dark organic-rich layers and clots. Note also the disseminated hematite (Hem) particles and local occurrence of quartz (Qtz).
- Figure 5. Stratigraphic variation of δ 13 C, δ 18 O and Mn/Sr ratios of the Hutuo Group. Three stages of the δ 13 C curve (A, B and C) are shown in detail in Fig. 6 .
- Figure 6. δ 13 C curves of the lower, middle and upper part of the Hutuo carbonates.
- Figure 7. Cross-plots illustrating post-sedimentary alteration of the carbonates.

- Previous Article
- Next Article
- Introduction
- Acknowledgements
Extending the record of the Lomagundi–Jatuli carbon isotope excursion in the Labrador Trough, Canada
Corresponding author: Malcolm Hodgskiss (email: [email protected] ).
- Open the PDF for in another window
- View This Citation
- Add to Citation Manager
- Permissions
- Search Site
Malcolm S.W. Hodgskiss , Kelsey G. Lamothe , Galen P. Halverson , Erik A. Sperling; Extending the record of the Lomagundi–Jatuli carbon isotope excursion in the Labrador Trough, Canada. Canadian Journal of Earth Sciences 2020;; 57 (9): 1089–1102. doi: https://doi.org/10.1139/cjes-2019-0198
Download citation file:
- Ris (Zotero)
The Labrador Trough in northern Québec and Labrador is a 900 km long Rhyacian–Orosirian orogenic belt containing mixed sedimentary–volcanic successions. Despite having been studied intensively since the 1940s, relatively few chemostratigraphic studies have been conducted. To improve our understanding of the Labrador Trough in the context of Earth history, and better constrain the local record of the Lomagundi–Jatuli carbon isotope excursion, high-resolution sampling and carbon isotope analyses of the Le Fer and Denault formations were conducted. Carbonate carbon isotopes (δ 13 C) in the Le Fer Formation record a large range in values from −4.4‰ to +6.9‰. This large range is likely attributable to a combination of post-depositional alteration and variable abundance of authigenic carbonate minerals; elemental ratios suggest that the most 13 C-enriched samples reflect the composition of the water column at the time of deposition. Cumulatively, these data suggest that the Lomagundi–Jatuli Excursion was ongoing during deposition of the Le Fer Formation, approximately 2 km higher in the stratigraphy than previously recognised. However, the possibility of a post-Lomagundi–Jatuli Excursion carbon isotope event cannot conclusively be ruled out. The directly overlying Denault Formation records a range in δ 13 C values, from −0.5‰ to +4.3‰, suggesting that it was deposited after the conclusion of the Lomagundi–Jatuli Excursion and that the contact between the Le Fer and Denault formations occurred sometime during the transition out of the Lomagundi–Jatuli Excursion, ca. 2106 to 2057 Ma.
Email alerts
- chemical composition
- chemostratigraphy
- Eastern Canada
- isotope ratios
- Labrador Trough
- lithostratigraphy
- Paleoproterozoic
- Precambrian
- Proterozoic
- Saguenay County Quebec
- Schefferville Quebec
- stable isotopes
- upper Precambrian
- Elizabeth Lake
- Denault Formation
- Marion Lake
- Le Fer Formation
- Kuujjuaq Quebec
- Logamundi-Jatuli carbon isotope excursion
- Lac Maquart
- N58°00'00" - N58°00'00", W68°19'60" - W68°19'60"
- N54°30'00" - N55°30'00", W68°00'00" - W66°00'00"

Citing articles via
Affiliations.
- Current Issue
- Online ISSN 1480-3313
- ISSN 0008-4077
- Copyright © 2024 Canadian Science Publishing
- How to Subscribe
- Privacy Policy
- For Librarians
- For Industry
- For Society Members
- For Authors
- Terms & Conditions
- 1750 Tysons Boulevard, Suite 1500
- McLean, VA 22102
- Telephone: 1-800-341-1851
- Copyright © 2024 GeoScienceWorld
This Feature Is Available To Subscribers Only
Sign In or Create an Account
Electrostal History and Art Museum
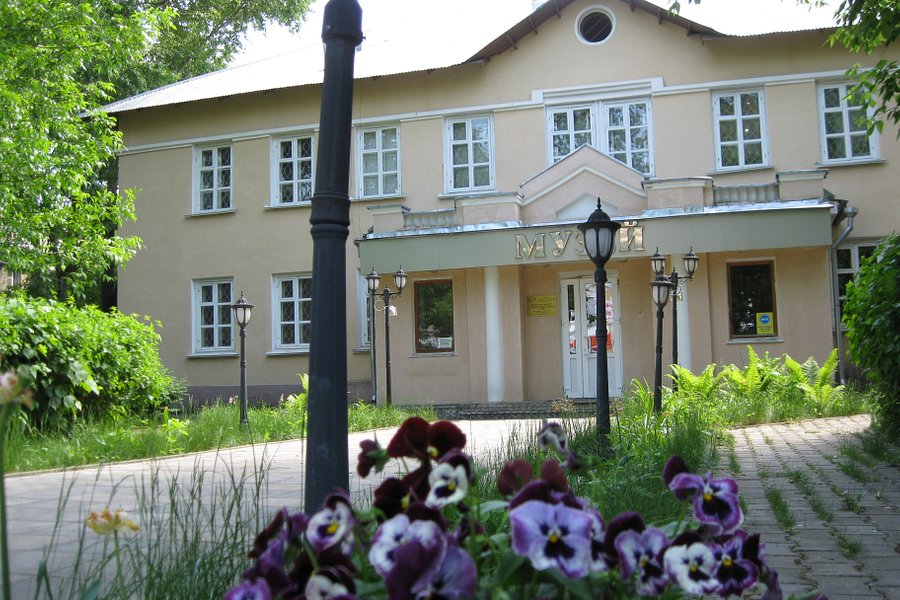
Most Recent: Reviews ordered by most recent publish date in descending order.
Detailed Reviews: Reviews ordered by recency and descriptiveness of user-identified themes such as wait time, length of visit, general tips, and location information.
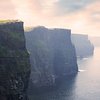
Electrostal History and Art Museum - All You Need to Know BEFORE You Go (2024)
- (0.19 mi) Elektrostal Hotel
- (1.21 mi) Yakor Hotel
- (1.27 mi) Mini Hotel Banifatsiy
- (1.18 mi) Elemash
- (1.36 mi) Hotel Djaz
- (0.07 mi) Prima Bolshogo
- (0.13 mi) Makecoffee
- (0.25 mi) Amsterdam Moments
- (0.25 mi) Pechka
- (0.26 mi) Mazhor

Turn Your Curiosity Into Discovery
Latest facts.
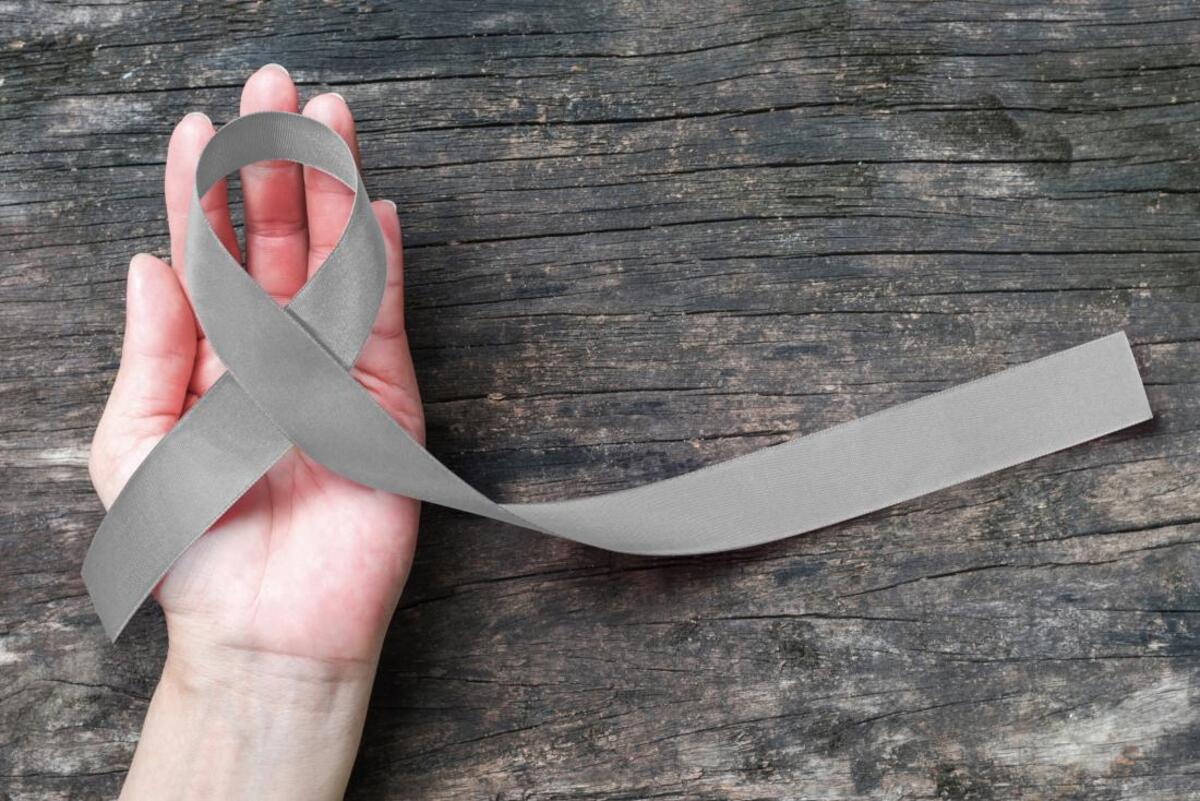
13 Facts About Brain Tumor Awareness Month US May
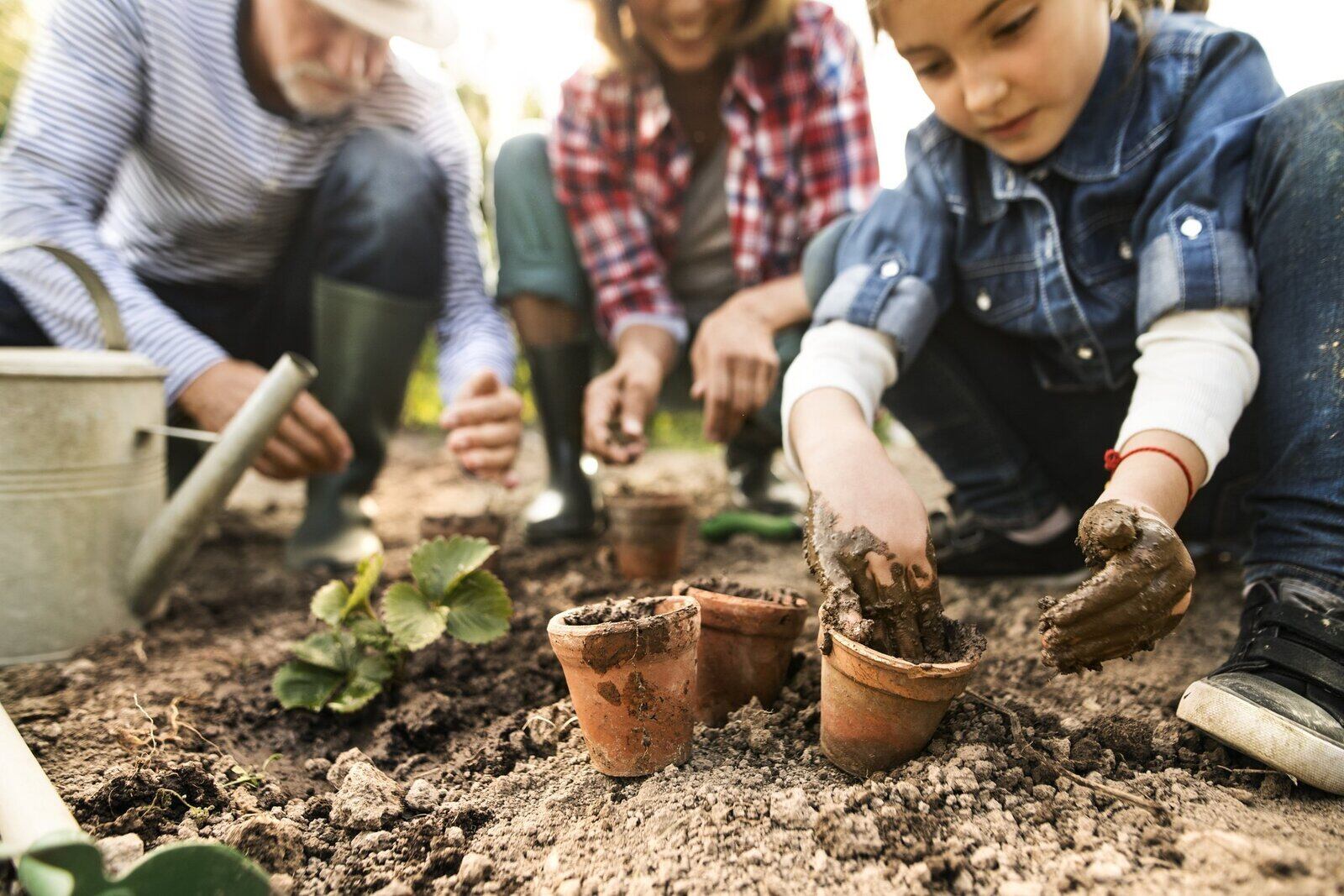
13 Facts About Community Garden Week Apr 1st To Apr 7th
40 facts about elektrostal.
Written by Lanette Mayes
Modified & Updated: 02 Mar 2024

Reviewed by Jessica Corbett
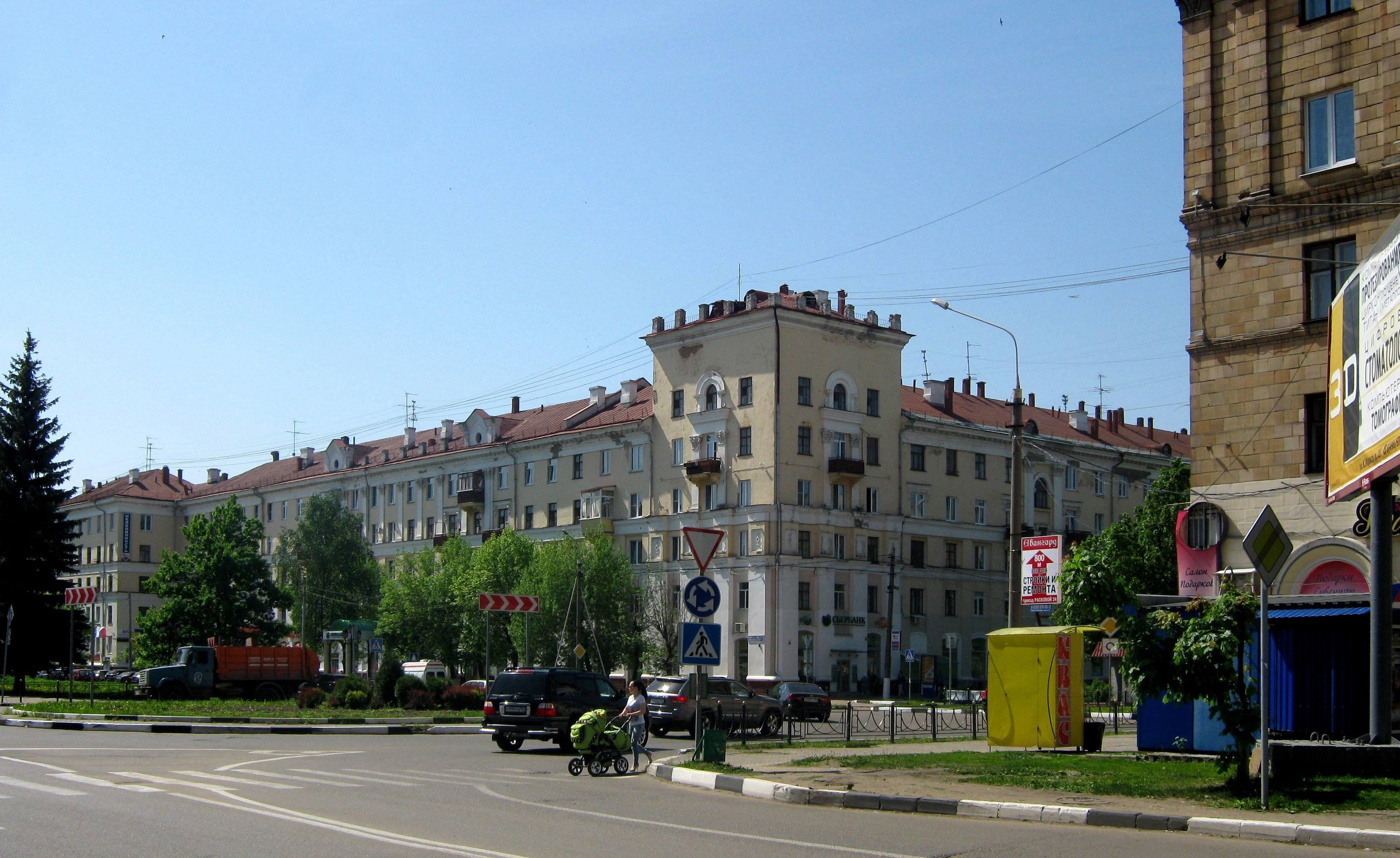
Elektrostal is a vibrant city located in the Moscow Oblast region of Russia. With a rich history, stunning architecture, and a thriving community, Elektrostal is a city that has much to offer. Whether you are a history buff, nature enthusiast, or simply curious about different cultures, Elektrostal is sure to captivate you.
This article will provide you with 40 fascinating facts about Elektrostal, giving you a better understanding of why this city is worth exploring. From its origins as an industrial hub to its modern-day charm, we will delve into the various aspects that make Elektrostal a unique and must-visit destination.
So, join us as we uncover the hidden treasures of Elektrostal and discover what makes this city a true gem in the heart of Russia.
Key Takeaways:
- Elektrostal, known as the “Motor City of Russia,” is a vibrant and growing city with a rich industrial history, offering diverse cultural experiences and a strong commitment to environmental sustainability.
- With its convenient location near Moscow, Elektrostal provides a picturesque landscape, vibrant nightlife, and a range of recreational activities, making it an ideal destination for residents and visitors alike.
Known as the “Motor City of Russia.”
Elektrostal, a city located in the Moscow Oblast region of Russia, earned the nickname “Motor City” due to its significant involvement in the automotive industry.
Home to the Elektrostal Metallurgical Plant.
Elektrostal is renowned for its metallurgical plant, which has been producing high-quality steel and alloys since its establishment in 1916.
Boasts a rich industrial heritage.
Elektrostal has a long history of industrial development, contributing to the growth and progress of the region.
Founded in 1916.
The city of Elektrostal was founded in 1916 as a result of the construction of the Elektrostal Metallurgical Plant.
Located approximately 50 kilometers east of Moscow.
Elektrostal is situated in close proximity to the Russian capital, making it easily accessible for both residents and visitors.
Known for its vibrant cultural scene.
Elektrostal is home to several cultural institutions, including museums, theaters, and art galleries that showcase the city’s rich artistic heritage.
A popular destination for nature lovers.
Surrounded by picturesque landscapes and forests, Elektrostal offers ample opportunities for outdoor activities such as hiking, camping, and birdwatching.
Hosts the annual Elektrostal City Day celebrations.
Every year, Elektrostal organizes festive events and activities to celebrate its founding, bringing together residents and visitors in a spirit of unity and joy.
Has a population of approximately 160,000 people.
Elektrostal is home to a diverse and vibrant community of around 160,000 residents, contributing to its dynamic atmosphere.
Boasts excellent education facilities.
The city is known for its well-established educational institutions, providing quality education to students of all ages.
A center for scientific research and innovation.
Elektrostal serves as an important hub for scientific research, particularly in the fields of metallurgy, materials science, and engineering.
Surrounded by picturesque lakes.
The city is blessed with numerous beautiful lakes, offering scenic views and recreational opportunities for locals and visitors alike.
Well-connected transportation system.
Elektrostal benefits from an efficient transportation network, including highways, railways, and public transportation options, ensuring convenient travel within and beyond the city.
Famous for its traditional Russian cuisine.
Food enthusiasts can indulge in authentic Russian dishes at numerous restaurants and cafes scattered throughout Elektrostal.
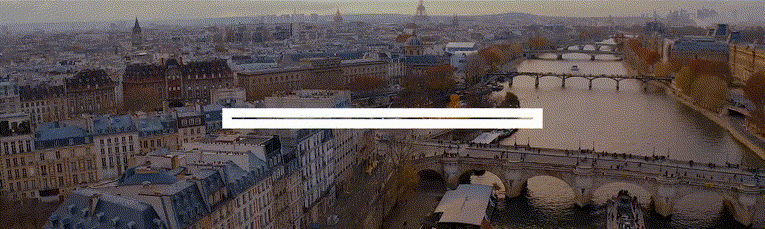
Home to notable architectural landmarks.
Elektrostal boasts impressive architecture, including the Church of the Transfiguration of the Lord and the Elektrostal Palace of Culture.
Offers a wide range of recreational facilities.
Residents and visitors can enjoy various recreational activities, such as sports complexes, swimming pools, and fitness centers, enhancing the overall quality of life.
Provides a high standard of healthcare.
Elektrostal is equipped with modern medical facilities, ensuring residents have access to quality healthcare services.
Home to the Elektrostal History Museum.
The Elektrostal History Museum showcases the city’s fascinating past through exhibitions and displays.
A hub for sports enthusiasts.
Elektrostal is passionate about sports, with numerous stadiums, arenas, and sports clubs offering opportunities for athletes and spectators.
Celebrates diverse cultural festivals.
Throughout the year, Elektrostal hosts a variety of cultural festivals, celebrating different ethnicities, traditions, and art forms.
Electric power played a significant role in its early development.
Elektrostal owes its name and initial growth to the establishment of electric power stations and the utilization of electricity in the industrial sector.
Boasts a thriving economy.
The city’s strong industrial base, coupled with its strategic location near Moscow, has contributed to Elektrostal’s prosperous economic status.
Houses the Elektrostal Drama Theater.
The Elektrostal Drama Theater is a cultural centerpiece, attracting theater enthusiasts from far and wide.
Popular destination for winter sports.
Elektrostal’s proximity to ski resorts and winter sport facilities makes it a favorite destination for skiing, snowboarding, and other winter activities.
Promotes environmental sustainability.
Elektrostal prioritizes environmental protection and sustainability, implementing initiatives to reduce pollution and preserve natural resources.
Home to renowned educational institutions.
Elektrostal is known for its prestigious schools and universities, offering a wide range of academic programs to students.
Committed to cultural preservation.
The city values its cultural heritage and takes active steps to preserve and promote traditional customs, crafts, and arts.
Hosts an annual International Film Festival.
The Elektrostal International Film Festival attracts filmmakers and cinema enthusiasts from around the world, showcasing a diverse range of films.
Encourages entrepreneurship and innovation.
Elektrostal supports aspiring entrepreneurs and fosters a culture of innovation, providing opportunities for startups and business development.
Offers a range of housing options.
Elektrostal provides diverse housing options, including apartments, houses, and residential complexes, catering to different lifestyles and budgets.
Home to notable sports teams.
Elektrostal is proud of its sports legacy, with several successful sports teams competing at regional and national levels.
Boasts a vibrant nightlife scene.
Residents and visitors can enjoy a lively nightlife in Elektrostal, with numerous bars, clubs, and entertainment venues.
Promotes cultural exchange and international relations.
Elektrostal actively engages in international partnerships, cultural exchanges, and diplomatic collaborations to foster global connections.
Surrounded by beautiful nature reserves.
Nearby nature reserves, such as the Barybino Forest and Luchinskoye Lake, offer opportunities for nature enthusiasts to explore and appreciate the region’s biodiversity.
Commemorates historical events.
The city pays tribute to significant historical events through memorials, monuments, and exhibitions, ensuring the preservation of collective memory.
Promotes sports and youth development.
Elektrostal invests in sports infrastructure and programs to encourage youth participation, health, and physical fitness.
Hosts annual cultural and artistic festivals.
Throughout the year, Elektrostal celebrates its cultural diversity through festivals dedicated to music, dance, art, and theater.
Provides a picturesque landscape for photography enthusiasts.
The city’s scenic beauty, architectural landmarks, and natural surroundings make it a paradise for photographers.
Connects to Moscow via a direct train line.
The convenient train connection between Elektrostal and Moscow makes commuting between the two cities effortless.
A city with a bright future.
Elektrostal continues to grow and develop, aiming to become a model city in terms of infrastructure, sustainability, and quality of life for its residents.
In conclusion, Elektrostal is a fascinating city with a rich history and a vibrant present. From its origins as a center of steel production to its modern-day status as a hub for education and industry, Elektrostal has plenty to offer both residents and visitors. With its beautiful parks, cultural attractions, and proximity to Moscow, there is no shortage of things to see and do in this dynamic city. Whether you’re interested in exploring its historical landmarks, enjoying outdoor activities, or immersing yourself in the local culture, Elektrostal has something for everyone. So, next time you find yourself in the Moscow region, don’t miss the opportunity to discover the hidden gems of Elektrostal.
Q: What is the population of Elektrostal?
A: As of the latest data, the population of Elektrostal is approximately XXXX.
Q: How far is Elektrostal from Moscow?
A: Elektrostal is located approximately XX kilometers away from Moscow.
Q: Are there any famous landmarks in Elektrostal?
A: Yes, Elektrostal is home to several notable landmarks, including XXXX and XXXX.
Q: What industries are prominent in Elektrostal?
A: Elektrostal is known for its steel production industry and is also a center for engineering and manufacturing.
Q: Are there any universities or educational institutions in Elektrostal?
A: Yes, Elektrostal is home to XXXX University and several other educational institutions.
Q: What are some popular outdoor activities in Elektrostal?
A: Elektrostal offers several outdoor activities, such as hiking, cycling, and picnicking in its beautiful parks.
Q: Is Elektrostal well-connected in terms of transportation?
A: Yes, Elektrostal has good transportation links, including trains and buses, making it easily accessible from nearby cities.
Q: Are there any annual events or festivals in Elektrostal?
A: Yes, Elektrostal hosts various events and festivals throughout the year, including XXXX and XXXX.
Was this page helpful?
Our commitment to delivering trustworthy and engaging content is at the heart of what we do. Each fact on our site is contributed by real users like you, bringing a wealth of diverse insights and information. To ensure the highest standards of accuracy and reliability, our dedicated editors meticulously review each submission. This process guarantees that the facts we share are not only fascinating but also credible. Trust in our commitment to quality and authenticity as you explore and learn with us.
Share this Fact:
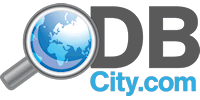
- Bahasa Indonesia
- Eastern Europe
- Moscow Oblast
Elektrostal
Elektrostal Localisation : Country Russia , Oblast Moscow Oblast . Available Information : Geographical coordinates , Population, Area, Altitude, Weather and Hotel . Nearby cities and villages : Noginsk , Pavlovsky Posad and Staraya Kupavna .
Information
Find all the information of Elektrostal or click on the section of your choice in the left menu.
- Update data
Elektrostal Demography
Information on the people and the population of Elektrostal.
Elektrostal Geography
Geographic Information regarding City of Elektrostal .
Elektrostal Distance
Distance (in kilometers) between Elektrostal and the biggest cities of Russia.
Elektrostal Map
Locate simply the city of Elektrostal through the card, map and satellite image of the city.
Elektrostal Nearby cities and villages
Elektrostal weather.
Weather forecast for the next coming days and current time of Elektrostal.
Elektrostal Sunrise and sunset
Find below the times of sunrise and sunset calculated 7 days to Elektrostal.
Elektrostal Hotel
Our team has selected for you a list of hotel in Elektrostal classified by value for money. Book your hotel room at the best price.
Elektrostal Nearby
Below is a list of activities and point of interest in Elektrostal and its surroundings.
Elektrostal Page
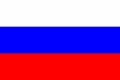
- Information /Russian-Federation--Moscow-Oblast--Elektrostal#info
- Demography /Russian-Federation--Moscow-Oblast--Elektrostal#demo
- Geography /Russian-Federation--Moscow-Oblast--Elektrostal#geo
- Distance /Russian-Federation--Moscow-Oblast--Elektrostal#dist1
- Map /Russian-Federation--Moscow-Oblast--Elektrostal#map
- Nearby cities and villages /Russian-Federation--Moscow-Oblast--Elektrostal#dist2
- Weather /Russian-Federation--Moscow-Oblast--Elektrostal#weather
- Sunrise and sunset /Russian-Federation--Moscow-Oblast--Elektrostal#sun
- Hotel /Russian-Federation--Moscow-Oblast--Elektrostal#hotel
- Nearby /Russian-Federation--Moscow-Oblast--Elektrostal#around
- Page /Russian-Federation--Moscow-Oblast--Elektrostal#page
- Terms of Use
- Copyright © 2024 DB-City - All rights reserved
- Change Ad Consent Do not sell my data
Lomagundi Carbon Isotope Excursion
- Living reference work entry
- Later version available View entry history
- First Online: 01 January 2015
- Cite this living reference work entry
- Andrey Bekker 8
775 Accesses
6 Citations
7 Altmetric
Lomagundi–Jatuli carbon isotope excursion ; Paleoproterozoic carbon isotope excursion
The Lomagundi carbon isotope excursion (LCIE), sensu stricto (s.s.), refers to highly positive carbon isotope values (on the average +8 ‰, but values as high as +16 ‰ are not uncommon with the highest values observed at +28 ‰) in Paleoproterozoic sedimentary carbonates deposited between ~2.22 and 2.06 Ga (Karhu and Holland 1996 ; Bekker et al. 2003 ), likely reflecting seawater composition. The beginning and the end of the excursion have not so far been recognized in the sedimentary record, and there is also uncertainty about the exact age of these transitions. Positive (at ~2.32 Ga and between ~2.45 and ~2.32 Ga; Bekker et al. 2001 ; Rasmussen et al. 2013 ) and negative (between ~2.45 and 2.32 Ga; Bekker et al. 2005 ) carbon isotope excursions have been found leading to the LCIE s.s. The older positive carbon isotope excursions, in particular the ~2.32 Ga one, are considered to be an...
This is a preview of subscription content, log in via an institution to check access.
Access this chapter
Institutional subscriptions
References and Further Reading
Baker AJ, Fallick AE (1989a) Evidence from the Lewisian limestone for isotopically heavy carbon in two-thousand-million-year-old sea water. Nature 337:352–354
Article ADS Google Scholar
Baker AJ, Fallick AE (1989b) Heavy carbon in two-billion-year-old marbles from Lofoten-Vesterålen, Norway: implications for the Precambrian carbon cycle. Geochim Cosmochim Acta 53:1111–1115
Bekker A, Holland HD (2012) Oxygen overshoot and recovery during the early Paleoproterozoic. Earth Planet Sci Lett 317–318:295–304
Article Google Scholar
Bekker A, Karhu JA, Kaufman AJ (2006) Carbon isotope record for the onset of the Lomagundi carbon isotope excursion in the Great Lakes area, North America. Precambrian Res 148:145–180
Bekker A, Kaufman AJ, Karhu JA, Eriksson KA (2005) Evidence for Paleoproterozoic cap carbonates in North America. Precambrian Res 137:167–206
Bekker A, Holland HD, Wang P-L, Rumble D III, Stein HJ, Hannah JL, Coetzee LL, Beukes NJ (2004) Dating the rise of atmospheric oxygen. Nature 427:117–120
Bekker A, Karhu JA, Eriksson KA, Kaufman AJ (2003) Chemostratigraphy of Paleoproterozoic carbonate successions of the Wyoming Craton: tectonic forcing of biogeochemical change? Precambrian Res 120:279–325
Bekker A, Kaufman AJ, Karhu JA, Beukes NJ, Swart QD, Coetzee LL, Eriksson KA (2001) Chemostratigraphy of the Paleoproterozoic Duitschland Formation, South Africa: implications for coupled climate change and carbon cycling. Am J Sci 301:261–285
Crne AE, Melezhik VA, Lepland A, Fallick AE, Prave AR, Brasier AT (2014) Petrography and geochemistry of carbonate rocks of the Paleoproterozoic Zaonega Formation, Russia: documentation of 13C-depleted non-primary calcite. Precambrian Res 240:79–93
Galimov EM, Kuznetsova NG, Prokhorov VS (1968) On the problem of the Earth’s ancient atmosphere composition in connection with results of isotope analysis of carbon from the Precambrian carbonates. Geochemistry 11:1376–1381 (in Russian)
Google Scholar
Galimov EM, Migdisov AA, Ronov AB (1975) Variation in the isotopic composition of carbonate and organic carbon in sedimentary rocks during Earth’s history. Geochem Int 12(2):1–19
Karhu JA, Holland HD (1996) Carbon isotopes and the rise of atmospheric oxygen. Geology 24(10):867–870
Karhu JA (1993) Paleoproterozoic evolution of the carbon isotope ratios of sedimentary carbonates in the Fennoscandian shield, Espoo. Geol Surv Finland Bull 371:87
Konhauser KO, Lalonde SV, Planavsky NJ, Pecoits E, Lyons TW, Mojzsis SJ, Rouxel OJ, Barley ME, Rosìere C, Fralick PW, Kump LR, Bekker A (2011) Aerobic pyrite oxidation and acid rock drainage during the great oxidation event. Nature 478:369–373
Kump LR, Junium C, Arthur MA, Brasier A, Fallick A, Melezhik V, Lepland A, Črne AE, Luo G (2011) Isotopic evidence for massive oxidation of organic matter following the great oxidation event. Science 334:1694–1696
Martin AP, Condon DJ, Prave AR, Melezhik VA, Lepland A, Fallick AE (2013) Dating the termination of the Palaeoproterozoic Lomagundi-Jatuli carbon isotope event in the North Transfennoscandian Greenstone Belt. Precambrian Res 224:160–168
Melezhik VA, Huhma H, Condon DJ, Fallick AE, Whitehouse MJ (2007) Temporal constraints on the Paleoproterozoic Lomagundi-Jatuli carbon isotope event. Geology 35:655–658
Partin CA, Bekker A, Planavsky NJ, Scott CT, Gill BG, Li C, Podkovyrov V, Maslov A, Konhauser KO, Lalonde SV, Love GD, Poulton SW, Lyons TW (2013) Large-scale fluctuations in Precambrian atmospheric and oceanic oxygen levels from the record of U in shales. EPSL 369–370:284–293
Pavlov AA, Hurtgen MT, Kasting JF, Arthur MA (2003) Methane-rich Proterozoic atmosphere? Geology 31:87–90
Planavsky NJ, Bekker A, Hofmann A, Owens J, Lyons TW (2012) Sulfur record of rising and falling marine oxygen and sulfate levels during the Lomagundi Event. PNAS 109(45):18300–18305
Rasmussen B, Bekker A, Fletcher IR (2013) Correlation of Paleoproterozoic glaciations based on U-Pb zircon ages for tuff beds in the Transvaal and Huronian Supergroups. EPSL 382:173–180
Schidlowski M, Eichmann R, Junge CE (1975) Precambrian sedimentary carbonates: carbon and oxygen isotope geochemistry and implications for the terrestrial oxygen budget. Precambrian Res 2:1–69
Schidlowski M, Eichmann R, Junge CE (1976) Carbon isotope geochemistry of the Precambrian Lomagundi carbonate province, Rhodesia. Geochim Cosmochim Acta 40:449–455
Scott C, Wing BA, Bekker A, Planavsky NJ, Medvedev P, Bates SM, Yun M, Lyons TW (2014) Pyrite multiple-sulfur isotope evidence for rapid expansion and contraction of the early Paleoproterozoic seawater sulfate reservoir. Earth Planet Sci Lett 389:95–104
Sheldon ND (2013) Causes and consequences of low atmospheric pCO 2 in the Late Mesoproterozoic. Chem Geol 362:224–231
Download references
Author information
Authors and affiliations.
Department of Earth Sciences, University of California, Riverside, 408 Geology Building, Riverside, CA, 92521, USA
Andrey Bekker
You can also search for this author in PubMed Google Scholar
Corresponding author
Correspondence to Andrey Bekker .
Editor information
Editors and affiliations.
Centro de Biología Molecular, Universidad Autónoma de Madrid, Madrid, Spain
Ricardo Amils
Labo. d'Astrophysique de Bordeaux (LAB), Observatoire Aquitain des Sciences de l'Univers (OASU), Floirac CX, France
Muriel Gargaud
Centro de Astrobiología (CAB), Inst. Nacional de Técnica Aeroespacial, Torrejón de Ardoz, Madrid, Spain
José Cernicharo Quintanilla
Geophysical Lab., Carnegie Institution of Washington, Washington, District of Columbia, USA
Henderson James Cleaves
Astronomy Department, University of Massachusetts, Amherst, Massachusetts, USA
William M. Irvine
Dépt. Sc. de la Terre et de l’Atmosphère, Université du Québec, Montréal, Montreal, Québec, Canada
Daniele Pinti
Centre National d'Etudes Spatiale (CNES) DPI/E2U, Paris CX, France
Michel Viso
Rights and permissions
Reprints and permissions
Copyright information
© 2014 Springer-Verlag Berlin Heidelberg
About this entry
Cite this entry.
Bekker, A. (2014). Lomagundi Carbon Isotope Excursion. In: Amils, R., et al. Encyclopedia of Astrobiology. Springer, Berlin, Heidelberg. https://doi.org/10.1007/978-3-642-27833-4_5127-1
Download citation
DOI : https://doi.org/10.1007/978-3-642-27833-4_5127-1
Received : 24 July 2014
Accepted : 23 September 2014
Published : 30 April 2015
Publisher Name : Springer, Berlin, Heidelberg
Online ISBN : 978-3-642-27833-4
eBook Packages : Springer Reference Physics and Astronomy Reference Module Physical and Materials Science Reference Module Chemistry, Materials and Physics
- Publish with us
Policies and ethics
Chapter history
DOI: https://doi.org/10.1007/978-3-642-27833-4_5127-2
DOI: https://doi.org/10.1007/978-3-642-27833-4_5127-1
- Find a journal
- Track your research
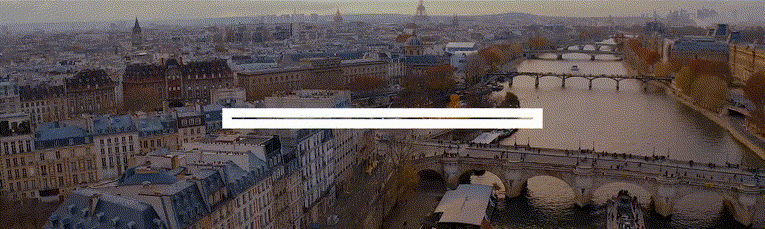
IMAGES
VIDEO
COMMENTS
The early to mid-Paleoproterozoic Lomagundi-Jatuli Excursion (LJE) is ostensibly the largest magnitude (approximately +5 to +30‰), longest duration (ca. 130-250 million years) positive carbon isotope excursion measured in carbonate rocks in Earth history. The LJE has been attributed to large nutrient fluxes, an increase in the size of the biosphere, a reorganization of the global carbon ...
The Paleoproterozoic Lomagundi-Jatuli Event (LJE) is generally considered the largest, in both amplitude and duration, positive carbonate C-isotope ( δ 13 C carb) excursion in Earth history.Conventional thinking is that it represents a global perturbation of the carbon cycle between 2.3-2.1 Ga linked directly with, and in part causing, the postulated rise in atmospheric oxygen during ...
The approximately 2,220-2,060 million years old Lomagundi-Jatuli Event was the longest positive carbon isotope excursion in Earth history and is traditionally interpreted to reflect an ...
The ~2.22-2.06 Ga Lomagundi Event was the longest positive carbon isotope excursion in Earth's history and is commonly interpreted to reflect perturbations in continental weathering and the ...
The Lomagundi-Jatuli Event (LJE) is one of the largest and earliest positive carbon isotope excursions preserving δ13Ccarb values between +5 and +16‰ in Paleoproterozoic carbonates worldwide. However, the duration, amplitude and patterns of these excursions remain poorly constrained. The 2.14-1.83 Ga Hutuo Group in the North China Craton is a >10 km thick volcano- sedimentary sequence ...
Records of the Lomagundi-Jatuli Event (LJE) are well preserved globally, but high δ 13 C carb carbonates have not been identified in the North China Craton (NCC). Our results on ~3-4 km thick carbonates from the newly confirmed Palaeoproterozoic successions in Fanhe Basin in the northeastern NCC show that the ~2.20-2.06 Ga carbonates have positive carbon isotope excursion and those ...
The directly overlying Denault Formation records a range in δ 13 C values, from −0.5‰ to +4.3‰, suggesting that it was deposited after the conclusion of the Lomagundi-Jatuli Excursion and that the contact between the Le Fer and Denault formations occurred sometime during the transition out of the Lomagundi-Jatuli Excursion, ca. 2106 ...
The Palaeoproterozoic Lomagundi-Jatuli Event is one of the largest magnitude and earliest known positive carbonate carbon isotope excursions, preserving δ 13 C values between + 5 and + 16‰ and even higher. It is recorded in sedimentary rocks on all continents bar Antarctica and spans stratigraphic thicknesses ranging from several to many tens of metres.
The Orosirian Period (2050-1800 Ma) of the Paleoproterozoic Era represents an interval between the Great Oxidation Event and Lomagundi-Jatuli Excursion, and the apparent environmental stability ...
The Paleoproterozoic Lomagundi-Jatuli Event (LJE) is generally considered the largest, in both amplitude and duration, positive carbonate C-isotope (δ 13 C carb) excursion in Earth history.Conventional thinking is that it represents a global perturbation of the carbon cycle between 2.3-2.1 Ga linked directly with, and in part causing, the postulated rise in atmospheric oxygen during the ...
The Lomagundi carbon isotope excursion (LCIE), sensu stricto (s.s.), refers to highly positive carbon isotope values (on the average +8 ‰, but values as high as +16 ‰ are not uncommon with the highest values observed at +28 ‰) in Paleoproterozoic sedimentary carbonates deposited between ∼2.22 and 2.06 Ga (Karhu and Holland 1996; Bekker et al. 2003), likely reflecting seawater composition.
The Termination and Aftermath of the Lomagundi-Jatuli Carbon Isotope Excursions 299 Figure 1. (a) Tectonic divisions of the North China Craton highlighting exposed Archean and Paleoproterozoic rocks (modified after Zhao et al., 1999). (b) Simplified geological map of the Wutai area (modified after Bai, 1986).
The Lomagundi-Jatuli Event (LJE) is one of the largest and earliest positive carbon isotope excursions preserving δ 13 C carb values between +5 and +16‰ in Paleoproterozoic carbonates worldwide. However, the duration, amplitude and patterns of these excursions remain poorly constrained. The 2.14-1.83 Ga Hutuo Group in the North China Craton is a > 10 km thick volcano-sedimentary sequence ...
The early to mid-Paleoproterozoic Lomagundi-Jatuli Excursion (LJE) is ostensibly the largest magnitude (approximately +5 to +30‰), longest duration (ca. 130-250 million years) positive carbon isotope excursion measured in carbonate rocks in Earth history. The LJE has been attributed to large nutrient fluxes, an increase in the size of the ...
The directly overlying Denault Formation records a range in δ 13 C values, from −0.5‰ to +4.3‰, suggesting that it was deposited after the conclusion of the Lomagundi-Jatuli Excursion and that the contact between the Le Fer and Denault formations occurred sometime during the transition out of the Lomagundi-Jatuli Excursion, ca. 2106 ...
The Lomagundi-Jatuli Event (LJE) refers to the significant positive carbon isotope excursion in seawater constituents that occurred immediately after the increase in atmospheric oxygen content during the Paleoproterozoic (2.22-2.06 Ga).
Art MuseumsHistory Museums. Write a review. Full view. All photos (22) Suggest edits to improve what we show. Improve this listing. The area. Nikolaeva ul., d. 30A, Elektrostal 144003 Russia. Reach out directly.
40 Facts About Elektrostal. Elektrostal is a vibrant city located in the Moscow Oblast region of Russia. With a rich history, stunning architecture, and a thriving community, Elektrostal is a city that has much to offer. Whether you are a history buff, nature enthusiast, or simply curious about different cultures, Elektrostal is sure to ...
The early to mid-Paleoproterozoic Lomagundi-Jatuli Excursion (LJE) is ostensibly the largest magnitude (approximately +5 to +30‰), longest duration (ca. 130-250 million years) positive carbon isotope excursion measured in carbonate rocks in Earth history. The LJE has been attributed to large nutrient fluxes, an increase in the size of the biosphere, a reorganization of the global carbon ...
Residents of a Moscow region town impacted by power outages have taken to the streets, demanding that local authorities restore heat to their homes as subzero temperatures grip the region, Russian ...
Elektrostal Geography. Geographic Information regarding City of Elektrostal. Elektrostal Geographical coordinates. Latitude: 55.8, Longitude: 38.45. 55° 48′ 0″ North, 38° 27′ 0″ East. Elektrostal Area. 4,951 hectares. 49.51 km² (19.12 sq mi) Elektrostal Altitude.
The Lomagundi carbon isotope excursion (LCIE), sensu stricto (s.s.), refers to highly positive carbon isotope values (on the average +8‰, but values as high as +16‰ are not uncommon with the highest values observed at +28‰) in Paleoproterozoic sedimentary carbonates deposited between ~2.22 and 2.06 Ga (Karhu and Holland 1996; Bekker et al. 2003), likely reflecting open-marine seawater ...
The Lomagundi carbon isotope excursion (LCIE), sensu stricto (s.s.), refers to highly positive carbon isotope values (on the average +8 ‰, but values as high as +16 ‰ are not uncommon with the highest values observed at +28 ‰) in Paleoproterozoic sedimentary carbonates deposited between ~2.22 and 2.06 Ga (Karhu and Holland 1996; Bekker et al. 2003), likely reflecting seawater composition.