Gamma rays: Everything you need to know about these powerful packets of energy
Gamma rays can only be detected by sensors made of dense metals and takes over six feet (1.8 meters) of concrete to block.
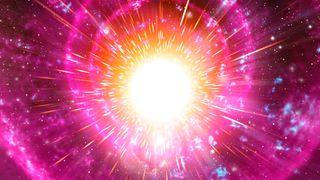
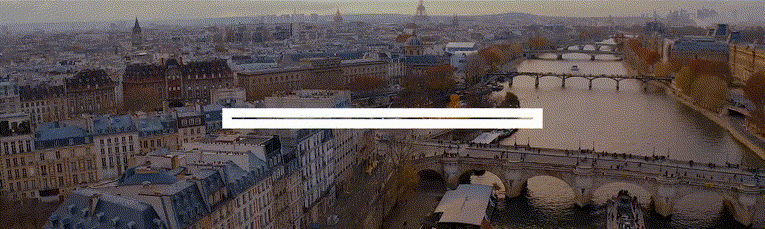
How were gamma rays discovered?
How to stop gamma rays, are gamma rays dangerous, gamma-ray astronomy, gamma-ray bursts, additional resources.
Gamma rays are high-energy photons produced by some of the most violent events in the universe.
Photons of light are massless particles that are essentially packets of energy. Because of a quantum-mechanical phenomenon known as wave-particle duality , particles can behave like waves, and photons are no different. Photons have wavelengths, and the amplitude of their wavelength determines where they sit on the electromagnetic spectrum . Radio and microwave photons sit at the lower energy, longer wavelength end of the spectrum, while in the shorter wavelength, higher-energy regime are photons of ultraviolet, X-rays and the most energetic of them all with the shortest wavelengths: gamma rays.
Gamma rays have wavelengths shorter than 10^-11 meters and frequencies above 30 x 10^18 hertz. The European Space Agency describes how gamma-ray photons have energies in excess of 100,000 electronvolts (eV). We can compare this to X-rays, which NASA describes as having energies between 100 eV and 100,000 eV , and optical photons that we can see with our eyes, which are about 1 eV.
Related: What is the cosmic microwave background?
On Earth, gamma rays are produced by radioactive decay, nuclear weapons and lightning, while in space they are produced by violent, high-energy sources such as solar flares , quasars , black holes tearing stars apart , black-hole accretion disks, exploding stars and the strong gravitational environments of neutron stars .
At the turn of the twentieth century, two forms of radiation emitted by decaying atoms were known, namely alpha particles (which are helium nuclei) and beta particles (which are electrons and positrons).
However, when the French chemist Paul Villard began experimenting with the radioactive element radium, which had been discovered two years prior by Marie and Pierre Curie , he noticed that the ionizing radiation produced by the decay of radium packed a harder punch than either alpha or beta particles.
This radiation received its name — gamma-rays — simply because gamma is the third letter in the Greek alphabet after alpha and beta. Unbeknownst to Villard and his cohorts in the early 1900s, the key difference between gamma rays and alpha/beta particles is that gamma rays are a form of light, whereas alpha and beta particles are made of matter.
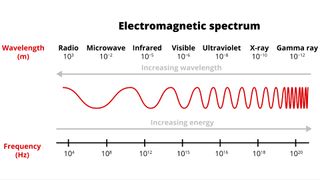
To block gamma rays requires a dense material, and the thickness of that material depends on the substance. To reduce the strength of incoming gamma rays by a billion, you need 13.8 feet (4.2 meters) of water, 6.6 feet (2 m) of concrete or 1.3 feet (0.39 m) of lead, according to the radiation protection solution website StemRad .
This poses a problem for gamma-ray telescopes, such as NASA's Fermi Space Telescope . Ordinary telescopes like the Hubble Space Telescope use mirrors and lenses to collect and focus light, but gamma rays will simply pass straight through an ordinary telescope. Instead, gamma-ray telescopes have to employ other means.
On the Fermi Space Telescope, a gamma-ray photon will pass through a device called the Anti-coincidence Detector, which blocks cosmic rays that might give a false signal, according to NASA . The gamma-ray is then absorbed by one of 16 sheets of tungsten, a material that is dense enough to stop gamma rays.
By interacting with the tungsten, the gamma-ray is converted into an electron and a positron (the antimatter or antiparticle counterpart of an electron), the paths of which are read by a tracker, which is a module of silicon strips interweaved by tungsten foil that can determine the direction that the gamma-ray came from in space, based on the trajectory of the electron and the positron.
Finally, the electron and then positron have their energies measured by a calorimeter — a device that measures the energy of a particle by absorbing it — made from cesium iodide, and therefore the energy of the gamma-ray can be determined.
Because of their high energy, gamma rays are ionizing, meaning they can dislodge electrons from atoms , ultimately damaging living cells and causing a hazard to health. However, as with all radiation, it depends upon the dose that you receive.
In small doses, very carefully targeted to limit exposure, they can be used safely as a medical diagnostic tool, or even to kill cancerous cells (ironic since exposure to radiation including gamma rays can cause cancer). In particular, one tool used by doctors is the ' Gamma Knife ', which is an ultra-precise form of surgery in which a beam of gamma rays cuts away diseased brain cells and can even penetrate deep into the brain without damaging the exterior lobes.
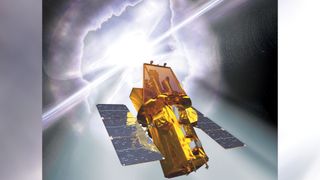
Given their ionizing power, it's fortunate that Earth's atmosphere is able to block gamma rays from space. For astronomers, however, that's unfortunate, because it means that to conduct gamma-ray astronomy observatories have to either be built on mountaintops where the atmosphere is thinner or sent into space.
The first gamma-ray space telescope was launched in 1961 on the NASA Explorer 11 satellite, but things didn't really begin to kick off until the late 1960s and early 1970s with a major finding, and it wasn't even an astronomical telescope that made the discovery.
Over the years there have been many observatories, both on the ground and in space, that have been designed to observe cosmic gamma-ray radiations. In 1990, NASA launched the Compton Gamma-Ray Observatory as the gamma-ray counterpart to the Hubble Space Telescope. The Compton Gamma-Ray Observatory explored the cosmos from 1991 until 2000. The aforementioned BeppoSAX was a joint Italian–Dutch mission that operated between 1996 and 2003, while NASA launched HETE-2 (the High-Energy Transient Explorer; HETE-1 had previously failed in orbit) that tracked down many GRBs between 2000 and 2008.
Currently, as of the end of 2022, several satellites , observatories and telescopes continue to conduct gamma-ray astronomy both on Earth and in space. NASA's Swift satellite , launched in 2004, combines both X-ray and gamma-ray observations, as does Italy's AGILE satellite launched in 2007. In 2002, the European Space Agency launched INTEGRAL , the International Gamma-Ray Astrophysics Laboratory.. The current most sophisticated gamma-ray space telescope is Fermi, which NASA launched in 2008.
Meanwhile, on the ground, there are several gamma-ray observatories including VERITAS (Very Energetic Radiation Imaging Telescope Array System) at the Fred Lawrence Whipple Observatory in Arizona and HESS (High Energy Stereoscopic System) in Namibia.
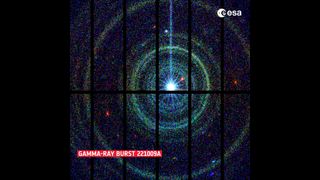
In 1963, the Soviet Union, the United Kingdom and the United States signed a nuclear test ban treaty that prohibited the world's superpowers from testing any nuclear devices in the atmosphere or in space. However, the U.S. was suspicious that the Soviet Union wouldn't adhere to the treaty, so they launched the Vela series of satellites to watch for any pulses of gamma-ray radiation that could be coming from secretive nuclear detonations. As it happened, gamma rays were detected, but from space: random blasts of powerful gamma-ray energy that seemed to be coming from all around the Earth. But how far away were these gamma-ray bursts ?
Related: Most powerful gamma-ray burst ever seen could help reveal how black holes are born
If these gamma-ray bursts, which are abbreviated to GRBs for short, were coming from our galaxy, then astronomers would detect them mostly in the plane of the Milky Way . Instead, they were spread all over the sky, it could mean only one of two things. Either they were very close, within our solar system , or they were very far away, beyond our galaxy. A special debate was even convened in 1995, echoing a similar ' Great Debate ' in 1920 between Harlow Shapley and Heber D. Curtis that discussed the size of our galaxy based on the distribution of globular clusters .
In the 1995 debate , chaired by Martin Rees, astronomer Bohdan Paczynski of Princeton University argued that GRBs came from very far away, while Donald Lamb of the University of Chicago reasoned that GRBs must be from close by because the energy required for them to be billions of light-years away would contravene the laws of physics.
Just two years later astronomers had their answer when the BeppoSAX satellite detected a gamma-ray burst that the William Herschel Telescope in the Canary Islands was able to quickly follow up on, in the process detecting the faint afterglow of whatever explosion had created the GRB. Measuring the redshift of the afterglow's light revealed it to have come from six billion light-years away. Bohdan Paczynski was right!
There are two main types of GRB. One type is called short GRBs which last just fractions of a second, while the other kind is known as the long GRBs, and can last many seconds up to an hour. Short GRBS are emitted during the merger of two neutron stars , while long GRBs are the death cries of rare, massive stars .
Physicists Andrew MacFadyen and Stan Woosley of the University of California, Santa Cruz, developed a model to explain how stars could explode and produce long GRBs without breaking the laws of physics. When a massive star with 50–100 times the mass of the sun reaches the end of its life, the star begins to collapse in on its core, and if the star is rotating fast enough, the energy within the collapsing layers rebounds off the core and is blasted out in two jets that move at almost the speed of light and blow the star apart. Charged particles within these jets spiral around powerful magnetic fields and produce something called synchrotron radiation, in the form of the gamma rays that we observe. Because the gamma rays are only released in the direction of the jets, and not in all directions at once, the total energy released does not contravene the laws of physics.
Learn more about ionizing radiation with the United States Environment Protection Agency (EPA) , and the American Cancer Society . Explore gamma rays in more detail in a tour of the electromagnetic spectrum with NASA Science.
Follow Keith Cooper on Twitter @21stCenturySETI. Follow us on Twitter @Spacedotcom and on Facebook .
Bibliography
Flash! The Hunt for the Biggest Explosions in the Universe by Govert Schilling (Cambridge University Press, 2002)
The Biggest Bangs: The Mystery of Gamma-Ray Bursts, The Most Violent Explosions in the Universe by Jonathan Katz (Oxford University Press, 2002)
Join our Space Forums to keep talking space on the latest missions, night sky and more! And if you have a news tip, correction or comment, let us know at: [email protected].
Get the Space.com Newsletter
Breaking space news, the latest updates on rocket launches, skywatching events and more!
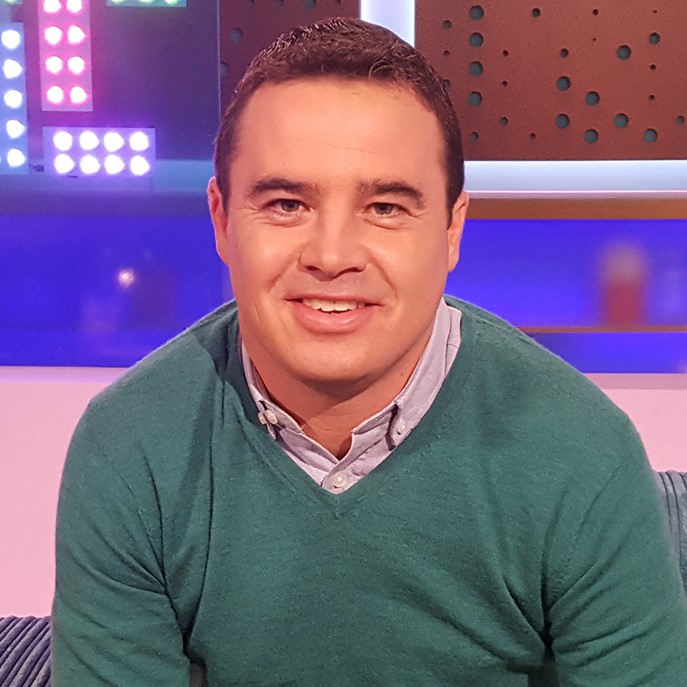
Keith Cooper is a freelance science journalist and editor in the United Kingdom, and has a degree in physics and astrophysics from the University of Manchester. He's the author of "The Contact Paradox: Challenging Our Assumptions in the Search for Extraterrestrial Intelligence" (Bloomsbury Sigma, 2020) and has written articles on astronomy, space, physics and astrobiology for a multitude of magazines and websites.
Ice-penetrating radar will help JUICE and other spacecraft find water beyond Earth
Uranus and Neptune aren't made of what we thought, new study hints
Boeing's Starliner spacecraft is 'go' for May 6 astronaut launch
- larbud Interesting; no mention of 1859. Reply
- billslugg The 1859 Carrington event was caused by a stream of charged particles from a solar flare. Any gamma rays emitted would have been blocked by the Earth's atmosphere. Reply
- View All 2 Comments
Most Popular
- 2 Russian cosmonauts make quick work of space station spacewalk
- 3 Curiosity rover may be 'burping' methane out of Mars' subsurface
- 4 Boeing Starliner 1st astronaut flight: Live updates
- 5 Lego reveals NASA Artemis rocket, Milky Way galaxy sets coming in May
What is Radiation? Properties of Radioactive Isotopes
- Types of Radiation
- Radioactive Decay Chains
isotopes decay chain
Radioactive decay is the process in which a radioactive atom spontaneously gives off radiation in the form of energy or particles to reach a more stable state. It is important to distinguish between radioactive material and the radiation it gives off.
Types of Radiation:
There are four types of radiation given off by radioactive atoms:
- Alpha particles
- Beta particles
Radioactive atoms give off one or more of these types of radiation to reach a more stable state. Additionally, each type of radiation has different properties that affect how we can detect it and how it can affect us.

- Alpha particles are large particles that travel up to an inch in the air.
- Alpha particles are very easy to block, even with something as thin as a sheet of paper.
- Alpha particles do not present an external hazard to people because they can’t get through our outer layer of dead skin cells.
- However, they can be very damaging to cells inside our bodies if we breathe or eat alpha-emitting radioactive material or if the radioactive material is introduced through an open wound.
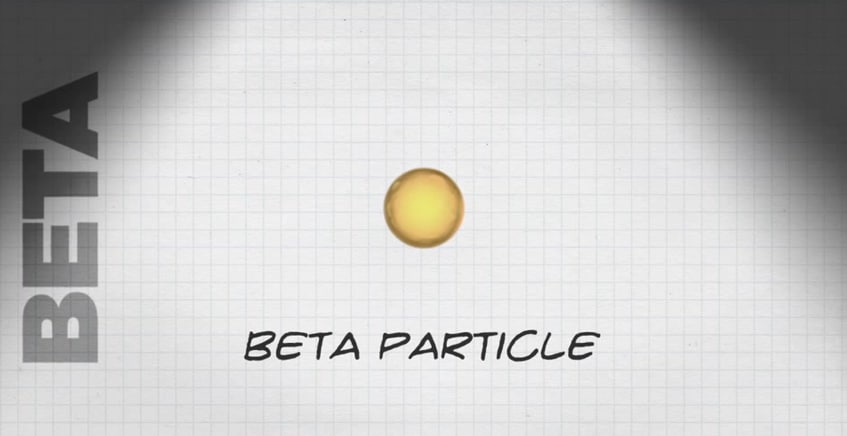
- Beta particles are smaller particles that travel several feet in air.
- Beta particles can be blocked effectively with a few inches of plastic, or even a layer of clothing.
- However, beta particles carry enough energy to cause burns on exposed skin and present an internal hazard if we breathe or eat beta-emitting radioactive material or if the radioactive material is introduced through an open wound.
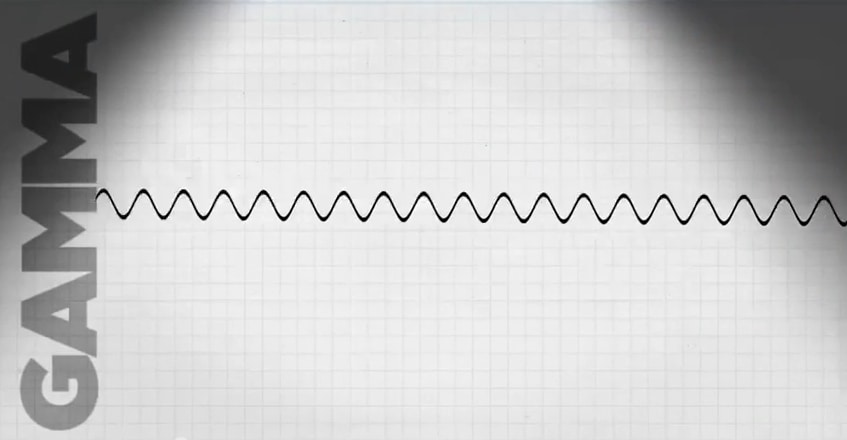
- Gamma rays can travel many yards in air.
- Gamma rays are primarily an external hazard because of their ability to go through material.
- It takes a few inches of lead or other dense substance to block gamma rays.
- Gamma rays also can be an internal hazard if we breathe or eat gamma-emitting radioactive materials, or if the radioactive material is introduce through an open wound, but the damage they do to cells inside our bodies is not as severe as that done by alpha and beta particles.
- The best way to protect yourself from a gamma-emitter is to increase the distance between yourself and the source.
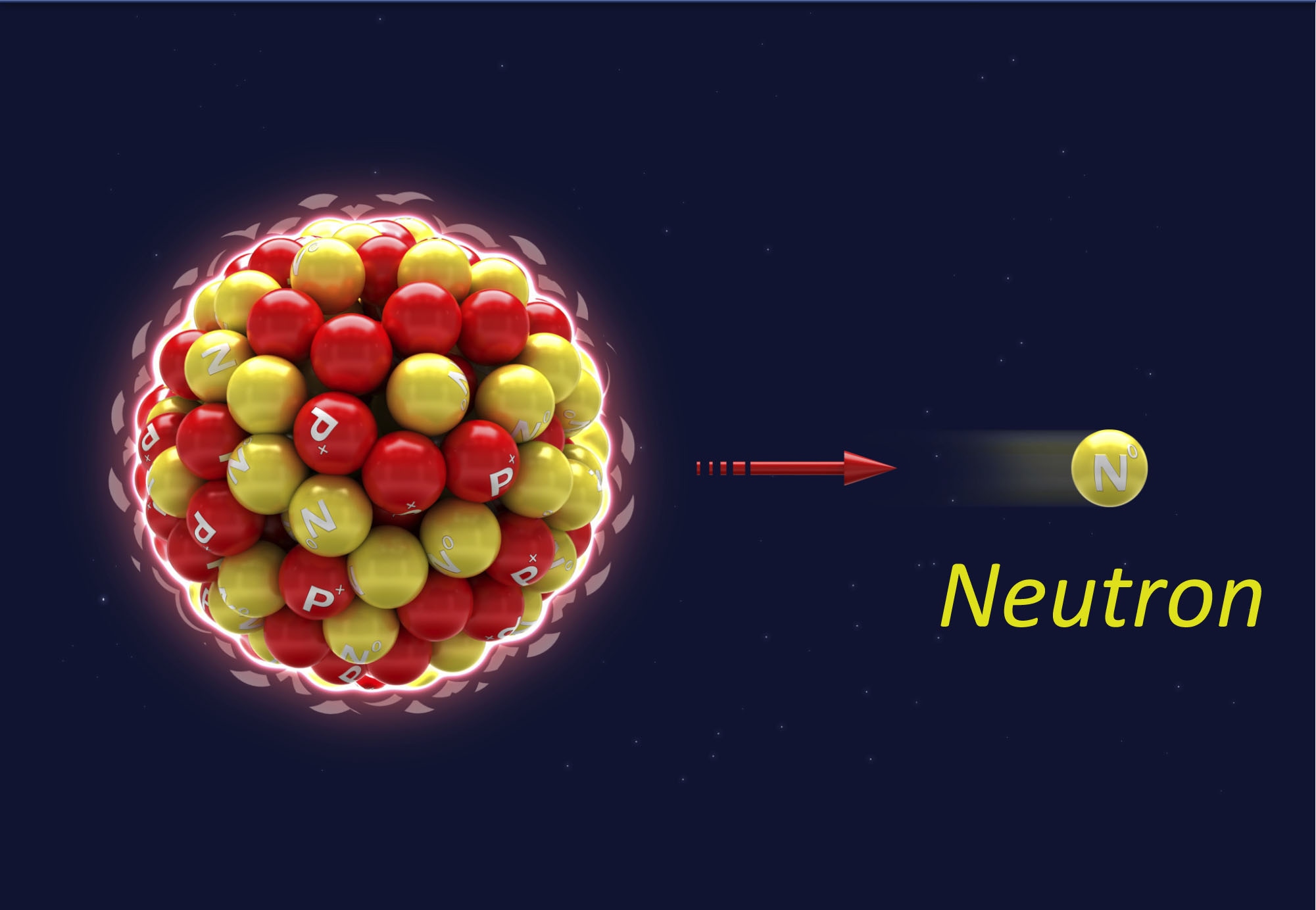
Neutrons are neutral particles with no electrical charge that can travel great distances in the air.
- As neutrons travel through matter, they crash with atoms. These atoms can become radioactive.
- Neutrons are more effective at damaging cells of the body than are other forms of ionizing radiation, such as x-rays or gamma rays.
- The best way to protect against neutron radiation is by providing shielding with thick, heavy materials such as lead, concrete, rock, or dirt.
Radionuclides can give off more than one kind of radiation, so it’s not uncommon to have a radionuclide that gives off both beta and gamma radiation, for example.
Another feature of each radionuclide is its half-life. Half-life is the length of time it takes for half of the radioactive atoms of a specific radionuclide to decay. A good rule of thumb is that, after seven half-lives, you will have less than one percent of the original amount of radiation.
Click here for a closer look at half life.
Depending on the radionuclide, this process could be fast or take a very long time – radioactive half-lives can range from milliseconds to hours, days, sometimes millions of years.
Radionuclides used in nuclear medicine procedures, have short half-lives.
- For example, technetium-99m, one of the most common medical isotopes used for imaging studies, has a half-life of 6 hours.
- The short half-life of technetium-99m helps keep the dose to the patient low. After 24 hours, the radioactivity from the procedure will be reduced by more than 90%.
Uranium is a radionuclide that has an extremely long half-life.
- Naturally occurring uranium-238 present in the Earth’s crust has a half-life of almost 4.5 billion years.
- If you take a soil sample anywhere in the world, including your backyard, you will find uranium atoms that date back to when the Earth was formed.
Let’s take a closer look at half-life.
If you start with 100 atoms, after one half-life you’ll have 50 radioactive atoms.

After two half-lives, you’ll have 25 radioactive atoms.

And after a third half-life, you’ll have 12 radioactive atoms.
Then 6, then 3, then 1, until eventually, all of the radioactive atoms in that population will reach their more stable state.
Some radionuclides go through a series of transformations before they reach a stable state. For example, uranium-238 ultimately transforms into a stable atom of lead. But in the process, several types of radioactive atoms are generated. This is called a decay chain . When uranium-238 decays, it produces several isotopes of:

As a result of this natural process, all of these radioactive atoms are part of our natural environment.
Alpha particles — The nucleus of a helium atom, made up of two neutrons and two protons with a charge of +2. Certain radioactive nuclei emit alpha particles. Alpha particles generally carry more energy than gamma or beta particles , and deposit that energy very quickly while passing through tissue. Alpha particles can be stopped by a thin layer of light material, such as a sheet of paper, and cannot penetrate the outer, dead layer of skin. Therefore, they do not damage living tissue when outside the body. When alpha-emitting atoms are inhaled or swallowed, however, they are especially damaging because they transfer relatively large amounts of ionizing energy to living cells. See also beta particle , gamma ray , neutron , x-ray .
Atom — The smallest particle of an element that can enter into a chemical reaction.
Beta Particles — Electrons ejected from the nucleus of a decaying atom . Although they can be stopped by a thin sheet of aluminum, beta particles can penetrate the dead skin layer, potentially causing burns. They can pose a serious direct or external radiation threat and can be lethal depending on the amount received. They also pose a serious internal radiation threat if beta-emitting atoms are ingested or inhaled . See also alpha particle , gamma ray , neutron , x-ray .
Decay Chain (Decay Series) — The series of decays that certain radioisotopes go through before reaching a stable form. For example, the decay chain that begins with uranium-238 (U-238) ends in lead-206 (Pb-206), after forming isotopes, such as uranium-234 (U-234), thorium-230 (Th-230), radium-226 (Ra-226), and radon-222 (Rn-222).
Gamma Rays — High-energy electromagnetic radiation emitted by certain radionuclides when their nuclei transition from a higher to a lower energy state. These rays have high energy and a short wave length. All gamma rays emitted from a given isotope have the same energy, a characteristic that enables scientists to identify which gamma emitters are present in a sample. Gamma rays penetrate tissue farther than do beta or alpha particles , but leave a lower concentration of ions in their path to potentially cause cell damage. Gamma rays are very similar to x-rays . See also neutron .
Isotope — A nuclide of an element having the same number of protons but a different number of neutrons .
Neutron — A small atomic particle possessing no electrical charge typically found within an atom’s nucleus . Neutrons are, as the name implies, neutral in their charge. That is, they have neither a positive nor a negative charge. A neutron has about the same mass as a proton . See also alpha particle , beta particle , gamma ray , nucleon , x-ray .
Radioactive Decay — Disintegration of the nucleus of an unstable atom by the release of radiation .
Radiation — Energy moving in the form of particles or waves. Familiar radiations are heat, light, radio waves, and microwaves. Ionizing radiation is a very high-energy form of electromagnetic radiation.
Radioactive Material — Material that contains unstable (radioactive) atoms that give off radiation as they decay .
Radionuclide — An unstable and therefore radioactive form of a nuclide .
[Back to Top]
Exit Notification / Disclaimer Policy
- The Centers for Disease Control and Prevention (CDC) cannot attest to the accuracy of a non-federal website.
- Linking to a non-federal website does not constitute an endorsement by CDC or any of its employees of the sponsors or the information and products presented on the website.
- You will be subject to the destination website's privacy policy when you follow the link.
- CDC is not responsible for Section 508 compliance (accessibility) on other federal or private website.

An official website of the United States government
The .gov means it's official. Federal government websites often end in .gov or .mil. Before sharing sensitive information, make sure you're on a federal government site.
The site is secure. The https:// ensures that you are connecting to the official website and that any information you provide is encrypted and transmitted securely.
- Publications
- Account settings
- Browse Titles
NCBI Bookshelf. A service of the National Library of Medicine, National Institutes of Health.
StatPearls [Internet]. Treasure Island (FL): StatPearls Publishing; 2024 Jan-.

StatPearls [Internet].
Radiation physics.
Justyn Nakashima ; Hieu Duong .
Affiliations
Last Update: May 1, 2023 .
- Definition/Introduction
hThe basic understanding of radiation physics is important when dealing with radiation and explaining its associated health effects. Atoms are the fundamental building blocks of matter and are composed of protons, neutrons, and electrons. A chemical element holds the same number of protons, which corresponds to its atomic number. However, depending on the number of neutrons in the nucleus, the atomic mass of the element can change. This difference is what creates an isotope of an element. Electrons orbit the nucleus and are arranged in layers called shells. Removing one of the outer electrons from an atom emits energy, usually in the form of gamma rays. This phenomenon can is called ionizing radiation. [1] An important principle in ionizing radiation is the stability of isotopes and nuclides. When an isotope of an element has too few or too many neutrons in the nucleus, it becomes unstable. Nuclei are most stable when protons and neutrons add up to certain numbers, including 2, 8, 20, 82, and 126. Physics shows that odd numbers of neutrons and protons are less stable than even numbers. In a radioactive decay process, these radioactive and unstable nuclides can transform into a more stable nuclide that ultimately emits beta particles, alpha particles, or photons that include X-rays and gamma rays. The particles emitted are a form of radiation. [2]
Radioactive isotopes have unique characteristics determined by the type of particle, how much energy it emits, and the rate of decay. The different types of particles include alpha, beta, and photons. It is important to appreciate the different properties of each particle to understand the effect it has on the atoms with which it interacts. Depending on the mass, velocity, charge, and electron density of the target material, the extent of kinetic energy lost and the subsequent transfer of energy to certain mediums vary. [3]
Alpha particles consist of two protons and two neutrons. The emission of this particle results in a reduction of its atomic mass by four and a reduction of its atomic number by two. The emission of alpha particles usually results from the radioactive decay of heavy elements such as plutonium, uranium, and radium. Alpha particles have a positive charge of +2 and have a large ionizing power. However, due to their charge and large density, they typically lose energy rapidly over a short period and distance. The average distance that an alpha particle travels is about 3 to 5 cm, and it usually cannot penetrate clothes or skin. As a result of these characteristics, to cause any effect or damage, alpha particles must be emitted close to their target. [4] [1] [5]
Beta particles subdivide into negative and positive emissions. A negative beta emission has a single negative charge, and it increases the number of protons by one and decreases the number of neutrons by one. A positive beta emission contains a single positive charge, and it decreases the number of protons by one and increases the number of neutrons by one. In both cases, the atomic mass stays the same, but it forms a different element. Unlike alpha particles, beta particles can travel far and penetrate through water and tissue with high energy. [4] [5]
When radioactive decay occurs in an element, residual energy produced from the transformation is typically stored within its nucleus and, therefore, ultimately excites the nucleus. Because high energy states cause instability, the nuclei release this energy to return to their baseline energy level. The energy that is released is typically in the form of gamma rays. [1] Gamma rays can also travel very far and can deposit or travel through substances such as iron, tissue, and even concrete. X-ray production occurs in a similar pattern, but instead of creating energy in the nucleus, energy generation occurs in the surrounding electrons. This process is called an internal conversion. When a nucleus is in an excited state, it ejects a gamma-ray. This gamma-ray interacts with one of the orbital electrons, typically in the innermost K layer. The gamma-ray is completely absorbed, and the atom is left with a void in the layer where the electron originally was. The vacant spot then becomes filled by an outer shell electron resulting in the production of an X-ray. X-rays can also be generated with the acceleration of an electron. This mechanism is demonstrated in the production of radiographic images utilizing X-rays. [4] [5] [6]
Radiation can be toxic when exposed in large amounts, and it is thus important to measure. The process of radioactive decay is random, and it is, therefore, difficult to predict when a particular atom will start to decay. However, it is possible to quantify the number of isotopes in a group that decays over a period of time. This rate of decay is called radioactivity, and it is proportional to the number of atoms of a certain radioactive material over its half-life. The half-life of an isotope is the time it takes for 50% of the nuclide in a group to decay. This rate is measured in Becquerels (Bq) in international units or Curies (Ci), which is used in the United States. By emitting particles, the radioactivity of an element can generate radiation. Radiation is clinically measured by the absorbed dose, which is equal to the amount of energy deposited in a specific region. It is calculated as the mean energy absorbed divided by the mass of the target material. In the practice of radiation oncology, the absorbed dose is measured as "gray" (Gy), with doses expressed in fractions over a time period. [7] [1]
- Issues of Concern
Radiation can be exposed internally or externally. Radiation exposure can occur internally when radionuclides enter the body through ingestion, inhalation, or the skin. When the nuclides are deposited into the skin, they undergo radioactive decay and emit radiation. External radiation exposure occurs via sources in the surrounding environment, such as nearby water, ground surfaces, air, or even x-ray tubes. In external radiation, radiation enters the body and penetrates the organs. Another example of this includes terrestrial and cosmic radiation, such as radiation from the sun. The sun continuously emits particles through space, eventually reaching the earth's surface and exposing humans to radiation. These natural exposures commonly occur in our daily life and are typically not destructive due to the body's ability to efficiently repair DNA damage. However, it is important to note that some tissues and organs are more sensitive to radiation than others. Rapidly dividing cells such as ones in the intestinal mucosa and bone marrow are the most vulnerable to radiation. [8]
Other issues of concern relate to geographical locations, house and work environments, and length of exposure. Mining for natural uranium is one example of the harmful effects of radiation. Uranium is known to have a very long half-life and emits powerful alpha particles when it decays. Uranium continues to decay, forming different elements throughout the process until it reaches a stable element of lead. During this decay process, unstable radioactive gas is produced, which can become suspended in the air; this causes a potential problem for miners due to the possibility of inspiring these particles. Alpha emissions contain a large amount of energy but can only travel short distances. When inhaled by miners, these particles can deposit into the lung tissue and cause deleterious effects directly to the alveoli cells. The same concept applies to any radiation that strikes human tissue. [3] [4] [8] [5]
Cancer development is always an issue of concern when it comes to radiation exposure. In both internal and external radiation, ionizing radiation can damage DNA indirectly or directly. Direct damage typically occurs with high ionizing particles such as alpha or beta particles. These particles can interact and transfer their energy directly to the cell's DNA, causing single-stranded or double-stranded breaks or chromosomal damage. Radiation can also cause damage indirectly by the formation of free radicals produced through ionization of surrounding water. This mechanism is present in about two-thirds of all radiation-induced cell damage. It is always important to note the increased risk of cancer development due to DNA mutations that occur with ionizing radiation. [3] [9]
- Clinical Significance
The principles of radiation physics apply to the field of radiation oncology, and ionizing radiation can be useful in the treatment of many different types of diseases. Radiation therapy uses targeted, high-dose radiation to indirectly or directly kill or stop the growth of cancer cells. The same mechanism of DNA damage is apparent in radiation therapy. Because cancer cells have a high mitotic rate, radiation can be very effective in cancer treatment. To put this into perspective, 1-2 Gy of radiation typically results in 40 double-stranded breaks in DNA. [3] External beam radiation is a form of radiation therapy that uses ionizing radiation to target tumors from sources outside of the body. Similar to the production of radiographic X-ray imaging, an electron is accelerated using a linear accelerator machine, and this results in the generation of photons. This external beam passes through the body, and energy is deposited throughout the path of the beam, targeting the primary tumor. Due to the damaging effects of radiation and the proximity of the tumor to surrounding healthy organs, these beams can be manipulated to minimize their damaging effects. [7] [4]
In 3D conformal radiation therapy, radiation can be given from many different directions so that the beam can converge on the target tumor, and it can better accommodate the size and shape of the tumor. With Intensity-modulated radiation therapy or IMRT, the dosage of the beams can be manipulated to decrease the dose to surrounding healthy tissue and increase the dose to the target tumor. Tumors are carefully mapped out using CT or MRI imaging, and the appropriate dose for the tumor, as well as the surrounding tissues, is proposed during planning. [1] These methods ultimately minimize the effect of radiation on the normal cells surrounding the tumor. Duration is another factor that can be manipulated depending on the type of disease and target organ. Radiation therapy can be fractionated so that a small dose of radiation can be given throughout a period of weeks, a single session, or a couple of days. For example, Stereotactic radiosurgery is a procedure usually done in one to five sessions, depending on the organ treated. It has utility in many conditions such as arteriovenous malformations, trigeminal neuralgia, acoustic neuromas, and cancers of the brain, liver, prostate, lung, breast, and spine. [7] [1]
Cancer is also treatable using proton beam radiation, where protons are used instead of photons and electrons. Hydrogen atoms first split into positively charged protons and negatively charged electrons. The protons are then shot through a vacuum tube into a linear accelerator, where their energy further accumulates as they accelerate along with the delivery system. Once it reaches the desired energy level of about 100 million to 200 million electron volts, these protons can penetrate any depth within the body. These proton beams can be manipulated in shape and direction via magnets. To further customize and increase the precision of delivery, the opening of the proton accelerator can be changed to shape the beam as it exits the machine. When using photons or X-rays in radiation therapy, energy is deposited throughout the path of the beam. As a result, it affects tissue before and after it hits the tumor. Proton beam therapy is beneficial in that physicians can control where the beam deposits most of its energy. Protons travel through the body, releasing some energy throughout the path, but once they reach a certain point, called the Bragg peak, they deposit most of their energy. As a result, this type of radiation therapy focuses more energy on the actual tumor and spares the surrounding tissue. [1]
Brachytherapy is another radiation therapy where a clinician implants radioactive material temporarily or permanently near or inside the target object. It is a type of internal radiation and is used to deliver higher doses of radiation in a more specific and smaller area that cannot be possible with external beam radiation. Brachytherapy categorization depends on the applicator type, anatomical site, source type, the type of implant, or the dose rate. Interstitial implants are radioactive sources either incased in seeds or wires, and they are inserted in the tumor. These are useful for cancers such as prostate cancer. Implants using molds or plaques are typically used for superficial lesions where radioactive sources are directly placed over the skin. Radioactive sources placed inside a body cavity such as the vagina or cervix are called intracavitary implants. High dose rate implants are given at dose rates greater than 20 cGy/min and are typically given only for a few minutes. [10] Low dose rate implants remain inside the target organ or tumor for a few days or permanently left inside. The inverse square law is the fundamental principle behind brachytherapy. This law states that the energy received at a given distance from the source is inversely proportional to the square of the distance from the source, denoted by 1/r^2. In other words, the energy intensity increases as the distance from the source to the target lessen and vice versa. If the source is three times as far, it is one-ninth the intensity or exposure. Brachytherapy uses this law to allow the delivery of higher doses to adjacent areas. [11] [12]
The precise delivery of radiation and its effectiveness in treating tumors has revolutionized the field of cancer treatment, and it has become part of the mainstay treatment for a multitude of cancers.
- Nursing, Allied Health, and Interprofessional Team Interventions
The use of radiation should be performed with caution as it can cause detrimental effects when used for an extended period. The International Committee on Radiological Protection or ICRP has proposed three recommendations before usage of ionizing radiation. The principle of justification states that the use of radiation should always do more good than harm. The principle of optimization of protection highlights the importance of using the lowest possible dose of radiation for any case. The principle of application of dose limits indicates that the dose given should never exceed the recommended dose for an individual. The environmental protection agency or EPA and the CDC have also recommended ways to protect against excessive exposure to radiation through the principle of ALARA (As Low As Reasonably Achievable). The ALARA principle proposes three concepts that can be useful to best protect against radiation exposure. It recommends minimizing the amount of time exposed to radiation, increasing the distance from the radiation source, and shielding against radiation any time you are at risk of exposure. It emphasizes the importance of barriers such as standing behind walls during appropriate times and using personal protective equipment around radioactive sources. [13] These principles merit attention for all healthcare professionals dealing with radiation to help protect themselves and their patients. The usage of radiation should take place in a structured environment, and productive communication is a necessity. Healthcare workers dealing with radiation should talk with their radiation safety professionals and safety officers to discuss the use of personal protective equipment and the proper instrumentation to protect themselves from radiation best. [3] [Level I]
When planning radiation therapy for a patient, a great deal of communication and teamwork is part of the treatment plan. Due to the danger of using high-energy radiation beams for treatment, careful planning needs to occur to minimize radiation exposure to healthy areas in the body. A radiation oncologist works with dosimetrists and medical physicists to calculate and propose the best dose and treatment plan for the patient. It can take several days to create a treatment regimen, and the result is a personalized treatment for that specific disease or tumor. Dosimetrists contribution to the treatment process involves generating the dose calculations and dose distribution of the radiation beam. Once the radiation oncologist prescribes a dose to the defined tumor volume, the dosimetrists will design and determine the treatment field, technique, and patient set up to accurately deliver the prescribed dose while avoiding vital structures. [14] Medical physicists also contribute to radiation therapy treatment by applying their knowledge in physics into the practice of medicine. One of their primary duties is to accurately calculate the radiation output during treatments using external radiation beams or internal radiation sources. Medical physicists use extensive calculations to determine the best dosage and projection of radiation while assessing other physiologic variables such as blood flow and metabolic rates. While they play a vital role in diagnosing and treating patients, they also aid in quality assurance, radiation safety, and technical consultancy of their medical equipment and facility. For the treatment team to efficiently and accurately diagnose and treat patients, all equipment must undergo extensive testing. It is also essential to address the safety, handling, storage, and disposal of radioactive substances. [7] [4] [15]
- Review Questions
- Access free multiple choice questions on this topic.
- Comment on this article.
Disclosure: Justyn Nakashima declares no relevant financial relationships with ineligible companies.
Disclosure: Hieu Duong declares no relevant financial relationships with ineligible companies.
This book is distributed under the terms of the Creative Commons Attribution-NonCommercial-NoDerivatives 4.0 International (CC BY-NC-ND 4.0) ( http://creativecommons.org/licenses/by-nc-nd/4.0/ ), which permits others to distribute the work, provided that the article is not altered or used commercially. You are not required to obtain permission to distribute this article, provided that you credit the author and journal.
- Cite this Page Nakashima J, Duong H. Radiation Physics. [Updated 2023 May 1]. In: StatPearls [Internet]. Treasure Island (FL): StatPearls Publishing; 2024 Jan-.
In this Page
Bulk download.
- Bulk download StatPearls data from FTP
Related information
- PMC PubMed Central citations
- PubMed Links to PubMed
Similar articles in PubMed
- Evidence for a new nuclear 'magic number' from the level structure of 54Ca. [Nature. 2013] Evidence for a new nuclear 'magic number' from the level structure of 54Ca. Steppenbeck D, Takeuchi S, Aoi N, Doornenbal P, Matsushita M, Wang H, Baba H, Fukuda N, Go S, Honma M, et al. Nature. 2013 Oct 10; 502(7470):207-10.
- Review Physics and basic parameters of brachytherapy. [J Surg Oncol. 1997] Review Physics and basic parameters of brachytherapy. Lee EJ, Weinhous MS. J Surg Oncol. 1997 Jun; 65(2):143-50.
- Photonuclear dose calculations for high-energy photon beams from Siemens and Varian linacs. [Med Phys. 2003] Photonuclear dose calculations for high-energy photon beams from Siemens and Varian linacs. Chibani O, Ma CM. Med Phys. 2003 Aug; 30(8):1990-2000.
- [Wilhelm Conrad Röntgen and the discovery of X-rays]. [Bull Acad Natl Med. 1996] [Wilhelm Conrad Röntgen and the discovery of X-rays]. Tubiana M. Bull Acad Natl Med. 1996 Jan; 180(1):97-108.
- Review Short Refresher of Radiobiology. [Nuclear and Radiological Emerg...] Review Short Refresher of Radiobiology. Averyn VS. Nuclear and Radiological Emergencies in Animal Production Systems, Preparedness, Response and Recovery. 2021
Recent Activity
- Radiation Physics - StatPearls Radiation Physics - StatPearls
Your browsing activity is empty.
Activity recording is turned off.
Turn recording back on
Connect with NLM
National Library of Medicine 8600 Rockville Pike Bethesda, MD 20894
Web Policies FOIA HHS Vulnerability Disclosure
Help Accessibility Careers
- Utility Menu

Harvard Natural Sciences Lecture Demonstrations
1 Oxford St Cambridge MA 02138 Science Center B-08A (617) 495-5824
- Key to Catalog
enter search criteria into the search box
Α, β, γ penetration and shielding, what it shows.
The interactions of the various radiations with matter are unique and determine their penetrability through matter and, consequently, the type and amount of shielding needed for radiation protection. Being electrically neutral, the interaction of gamma rays with matter is a statistical process and depends on the nature of the absorber as well as the energy of the gamma. There is always a finite probability for a gamma to penetrate a given thickness of absorbing material and so, unlike the charged particulate radiations which have a maximum range in the absorber where all are stopped regardless of source strength, some gammas will always get through and, given a strong enough source, a lot may get through.
How it Works
This demo is usually presented in conjunction with the Sources and Detection demo; details about the radionuclides and detectors used here can also be found in that writeup .
(1) Alpha particles interact with matter primarily through Coulomb forces between their positive charge and the negative charge of the atomic electrons within the absorber. The range of alphas of a given energy is a fairly unique quantity in a specific absorber material. [1] For a given energy, alpha particles are much slower than beta particles, giving rise to greater impulses. Additionally, its double charge (+2 e ) makes an alpha particle have a very high rate of energy loss in matter, thus making it heavily ionizing radiation. Consequently, the penetration depth of alpha particles is very small compared to the other radiations. For low density materials, the range [2] of 5.5 MeV alphas (from Am-241) is between 4.5 to 5 mg/cm 2 ; higher density materials give a range between 5 and 12 mg/cm 2 . The table below gives some specific values.
The thickness of a single sheet of paper (0.0035") is enough to stop all the alphas. [3]
(2) Beta particles also interact through Coulomb forces with the atomic electrons. Betas have much higher speeds due to their smaller mass, and smaller impulses are involved in collisions. Their penetration into matter is thus considerably greater than alphas, but because of the nature of the Coulomb force interactions, betas too are stopped by very little matter (compared to gammas). Because their masses are identical to the scattering electrons, large deviations in the beta particle path are possible, and even thin absorbers will attenuate betas by virtue of the fact that they readily get scattered out of the direct beam. Another difference that complicates the comparison is that, unlike mono-energetic alphas, beta particles come in a continuous spectrum of energies, with the average energy being about 1/3 the maximum. The low energy betas are rapidly attenuated.
A useful rule-of-thumb for the maximum range of electrons is that the range (in gm/cm 2 ) is half the maximum energy (in Mev). [4] This is of course complicated by the density: electron ranges tend to be about 2 mm per MeV in low-density materials, and about 1 mm per MeV in medium density absorbers. For our Sr/Y-90 source (maximum beta energy = 2.27 MeV, average energy = 1.13 MeV), more precise beta ranges are tabulated below:
C-14 gives off betas with a maximum energy of 0.156 MeV and average energy of 0.049 MeV. The maximum range of C-14 betas is only 0.25 mm (0.01") in plastic. We have both sources and it's nice to contrast them. A Harvard ID card is about 0.8 mm thick and stops all C-14 betas. Not so when you switch over to the Sr-90 source. A 3/8" (9.6 mm) thick piece of plastic is required to stop all the Sr-90 betas.
(3) Gamma ray interactions with matter are entirely different from that of charged particles. The lack of charge eliminates Coulomb interactions and allows gamma rays to be much more penetrating. The interactions that do occur are by way of the photoelectric effect, Compton scattering, and pair production. The probability for any of these happening is specified by a cross section, and the linear attenuation coefficients for gamma rays are defined by these cross sections.
Since linear attenuation coefficients vary with the density of the absorber, even for the same absorber material, the mass attenuation coefficient μ/ρ (linear attenuation coefficient μ in 1/cm -1 divided by the density ρ in g/cm 3 ) is more useful, and the attenuation law is written as
I = I o e -(μ/ρ)ρt Equation (1)
where I is the intensity of the radiation and t is the thickness. The product ρt is the significant parameter and the units (as with β and α particles) are mg/cm 2 , making the exponent in Equation (1) dimensionless.
Unlike charged particles, a certain percentage of gammas will always make it through the absorber, and it is useful to consider the half-value thickness of a given absorbing material for the gamma ray energies of interest. The half-value thicknesses are determined from Equation (1) using the linear attenuation or mass attenuation coefficients found in the references below. [6] Absorbers of these thicknesses attenuate the radiation reaching the detector by a factor of two and some of the common ones are tabulated below for Co-60 (1.33 and 1.17 MeV) and Cs-137 (662 keV).
Setting it Up
A Co-60 source (labeled #9) from the Phys 191 lab is the most convenient source for this demonstration in terms of strength (4 micro Ci as of 2016 ... half-life is 5.27 yrs). Position it approximately 2.5 inches in front of the Geiger-Muller tube. A 1/2" thick piece of lead cuts the count rate down by a factor of two and a 1.5" thick piece reduces the rate by a factor of ten.
Plastic, aluminum, steel, lead, and many other absorbers are available as needed. They range in thicknesses from hundreds of microns (foils) to several centimeters. The absorber is simply placed over the thin end-window of the G-M tube.
One doesn't really want to turn a simple demonstration into a lengthy laboratory exercise, so it's best to decide beforehand the salient features one wants to impress on the audience and use the appropriate absorbers and thicknesses to make the point.
AIP Physics Desk Reference , edited by E. Richard Cohen, David R. Lide, George L.. Trigg, (Springer, New York, 2003) G.F. Knoll, Radiation Detection and Measurement , 2nd ed, (Wiley, NY, 1989) G.W. Morgan, Some Practical Considerations in Radiation Shielding , Isotopes Division Circular B-4, (U.S. Atomic Energy Commission, Oak Ridge) CRC Handbook of Radioactive Nuclides , edited by Y. Wang, (Chemical Rubber Company, Ohio, 1969) A.H. Wapstra, G.J. Nijgh, and R. Van Lieshout, Nuclear Spectroscopy Tables , (North Holland, Amsterdam, 1959) X-ray Attenuation Coefficients from 10 keV to 100 MeV , National Bureau of Standards Circular No. 583 https://physics.nist.gov/PhysRefData/XrayMassCoef/tab3.html https://physics.nist.gov/PhysRefData/XrayMassCoef/tab2.html
[1] Indeed, in the early days of radiation measurement, alpha particle energies were measured indirectly by determining the absorber thickness equivalent to their mean range.
[2] The range is expressed in terms of (density)×(thickness), which is written as the mass/unit area of the absorber of a given thickness. Historically the units have been mg/cm 2 . Density·thickness (also sometimes referred to as mass·thickness) is a useful concept when discussing the energy loss of alphas and betas because, for absorber materials with similar neutron/proton ratios, a particle will encounter about the same number of electrons passing through absorbers of equal density·thickness. Therefore the stopping power and range, when expressed in these units, are roughly the same for materials that do not differ greatly in Z.
[3] The paper weighs 4.77 gm/sheet which gives it a density of 0.89 gm/cm 3 and a density·thickness of 7.9 mg/cm 2
[4] This rule of thumb is applicable only when E > 0.8 MeV. For other energy ranges, see Wang, p 912.
[5] Values are from Y. Wang ( reference ). Another rule-of-thumb is that the half-value range is approximately 1/7 of the maximum range but may vary between 1/5 and 1/10 (depending on beta energy and absorber density).
[6] The mass absorption coefficient also depends on the energy of the radiation. To calculate the half-value layer for a particular material and specific radiation energy, then one has to look up the "mass energy absorption coefficient" in the CRC Handbook or AIP Physics Desk Reference , or whichever reference is handy.
Demo Subjects
Newtonian Mechanics Fluid Mechanics Oscillations and Waves Electricity and Magnetism Light and Optics Quantum Physics and Relativity Thermal Physics Condensed Matter Astronomy and Astrophysics Geophysics Chemical Behavior of Matter Mathematical Topics
Key to Catalog Listings
Size : from small [S] (benchtop) to extra large [XL] (most of the hall) Setup Time : <10 min [t], 10-15 min [t+], >15 min [t++] /span> Rating : from good [★] to wow! [★★★★] or not rated [—]
Complete key to listings
Guidance for Radiation Accident Management
What is radiation.
Radiation is energy that comes from a source and travels through some material or through space. Light and heat are types of radiation. The kind of radiation discussed on this site is called ionizing radiation because it has enough energy to remove an electron from an atom, making that atom an ion.
In order to reach stability, these atoms give off, or emit, the excess energy or mass in the form of radiation. The two types of radiation are electromagnetic (like light) and particulate (i.e., mass given off with the energy of motion). Gamma radiation and X-rays are examples of electromagnetic radiation. Beta and alpha radiation are examples of particulate radiation. Ionizing radiation can also be produced by devices such as X-ray machines.
Irradiation refers to exposure to radiation. Irradiation occurs when all or part of the body is exposed to radiation from a source. Irradiation does not make a person radioactive.
What is radioactive contamination?
Contamination occurs when radioactive material is deposited on skin, clothing, or any place where is it not desired. It is important to remember that radiation does not spread or get "on" or "in" people; rather it is radioactive contamination that can spread. A person contaminated with radioactive materials will be irradiated until the source of radiation (the radioactive material) is removed.
- A person is externally contaminated if radioactive material is on skin or clothing.
- A person is internally contaminated if radioactive material is breathed in, swallowed, or absorbed through wounds.
- The environment is contaminated if radioactive material is spread about or uncontained.
What are the different types of radiation?
Alpha radiation.
Radiation is energy, in the form of particles or electromagnetic rays, released from radioactive atoms. The three most common types of radiation are alpha particles, beta particles, and gamma rays.
- Alpha radiation is not able to penetrate skin.
- Alpha-emitting materials can be harmful to humans if the materials are inhaled, swallowed, or absorbed through open wounds.
- A variety of instruments have been designed to measure alpha radiation. Special training in use of these instruments is essential for making accurate measurements.
- Instruments cannot detect alpha radiation through even a thin layer of water, blood, dust, paper, or other material, because alpha radiation is minimally penetrating.
- Alpha radiation travels a very short distance through air.
- Alpha radiation is not able to penetrate turnout gear, clothing, or a cover on a probe. Turnout gear and clothing can keep alpha emitters off of the skin. Personal protective equipment should be worn to protect clothing and otherwise uncovered skin from contamination of all types.
Beta radiation
- Beta radiation may travel meters in air and is moderately penetrating.
- Beta radiation can penetrate human skin to the innermost layer of the epidermis where new skin cells are produced. If beta-emitting contaminants are allowed to remain on the skin for a prolonged period of time, they may cause skin injury.
- Beta-emitting contaminants may be harmful if deposited internally.
- Most beta emitters can be detected with a survey instrument. Some beta emitters, however, produce very low energy, poorly penetrating radiation that may be difficult or impossible to detect. Examples of these are carbon-14, tritium, and sulfur-35.
- Clothing and turnout gear provide some protection against most beta radiation.Personal protective equipment should be worn to protect clothing and otherwise uncovered skin from contamination of all types.
Gamma radiation
- Gamma radiation and X-rays are electromagnetic radiation like visible light, radio waves, and ultraviolet light. These electromagnetic radiations differ only in the amount of energy they have. Gamma rays and X-rays are the most energetic of these.
- Gamma radiation is able to travel many meters in air and many centimeters in human tissue. It readily penetrates most materials.
- X-rays are like gamma rays. They can also travel over long distances in both air and human tissue.
- Radioactive materials that emit gamma radiation and X-rays constitute both an external and internal hazard to humans.
- Dense materials are needed for shielding from gamma radiation. Clothing and turnout gear provide little shielding from penetrating radiation but will prevent contamination of the skin by radioactive materials.
- Gamma radiation is detected with survey instruments, including civil defense instruments. Low levels can be measured with a standard Geiger counter.
- Gamma radiation or X-rays frequently accompany the emission of alpha and beta radiation.
- Instruments designed solely for alpha detection will not detect gamma radiation.
- Pocket chamber (pencil) dosimeters, film badges, thermoluminescent, and other types of dosimeters can be used to measure accumulated exposure to gamma radiation.
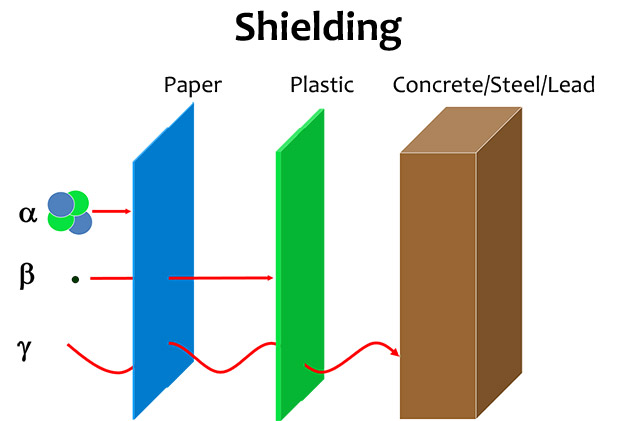
Radiation definitions
The following list provides a selection of general terms that are used to describe aspects of radiation.

- Gamma-rays: what are they?
- " onclick="window.open(this.href,'win2','status=no,toolbar=no,scrollbars=yes,titlebar=no,menubar=no,resizable=yes,width=640,height=480,directories=no,location=no'); return false;" rel="nofollow"> Print
Almost all of our information about the universe beyond our planet comes from the study of electromagnetic radiation. Gamma radiation is part of the electromagnetic spectrum that includes the familiar visible light and radio waves.
However, there are also many differences between gamma-rays and other forms of energy. For example, a gamma-ray photon has one million to one trillion (one million million) times the energy of a photon of visible light. And, unlike photons of radio and visible light, gamma-ray photons cannot penetrate the earth's atmosphere, for they are absorbed by interactions with air molecules ten miles above the Earth's surface. Also, gamma-rays cannot be reflected by mirrors. Thus, normal telescope optics cannot be used to enhance or increase the collection area of a gamma-ray detector.
Because gamma-rays can traverse great distances in space without absorption by intergalactic dust and gas, they can serve as powerful probes of distant regions of the cosmos as well as otherwise obscured regions of our own Milky Way .
Gamma-rays are produced on Earth from the radioactive decay of naturally occurring elements, and oncologists may use such gamma-rays to treat cancer tumors. Particle physicists can produce gamma-rays at accelerators such as Fermilab in Illinois or the Stanford Linear Accelerator in California, where they are used to study the properties of elementary matter. But very-high-energy gamma-rays like those emitted by astronomical bodies do not occur naturally on Earth.
- You are here:

- Atmospheric Cherenkov Technique and VERITAS Technologies
- VERITAS Specifications
- VERITAS Outstanding Contribution Awards
- VERITAS Governance
- VERITAS Code of Conduct
- History & Timeline
- Introduction to VHE Gamma-Ray Astrophysics
- VERITAS Results
- VERITAS Blazar Spectral Information & Light Curves
- VERITAS Blazar Long-term Monitoring
- Mrk 421 Long-term Light Curve
- Theses & dissertations
- Directions & Maps
- FLWO Visitor's Information
- Information for Mountain Users
Current Weather
- All-sky Camera
- How to Collaborate
- Current Associate Members
- Fermi Collaborative Agreement
- Outreach & Education
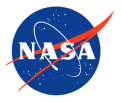
Suggested Searches
- Climate Change
- Expedition 64
- Mars perseverance
- SpaceX Crew-2
- International Space Station
- View All Topics A-Z
Humans in Space
Earth & climate, the solar system.
- The Universe
Aeronautics
Learning resources, news & events.
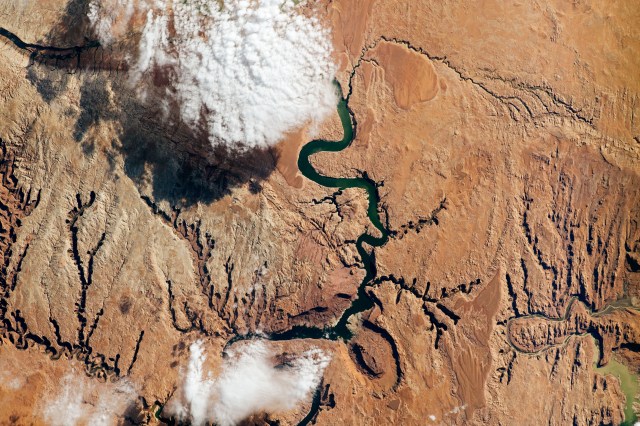
NASA-Led Study Provides New Global Accounting of Earth’s Rivers
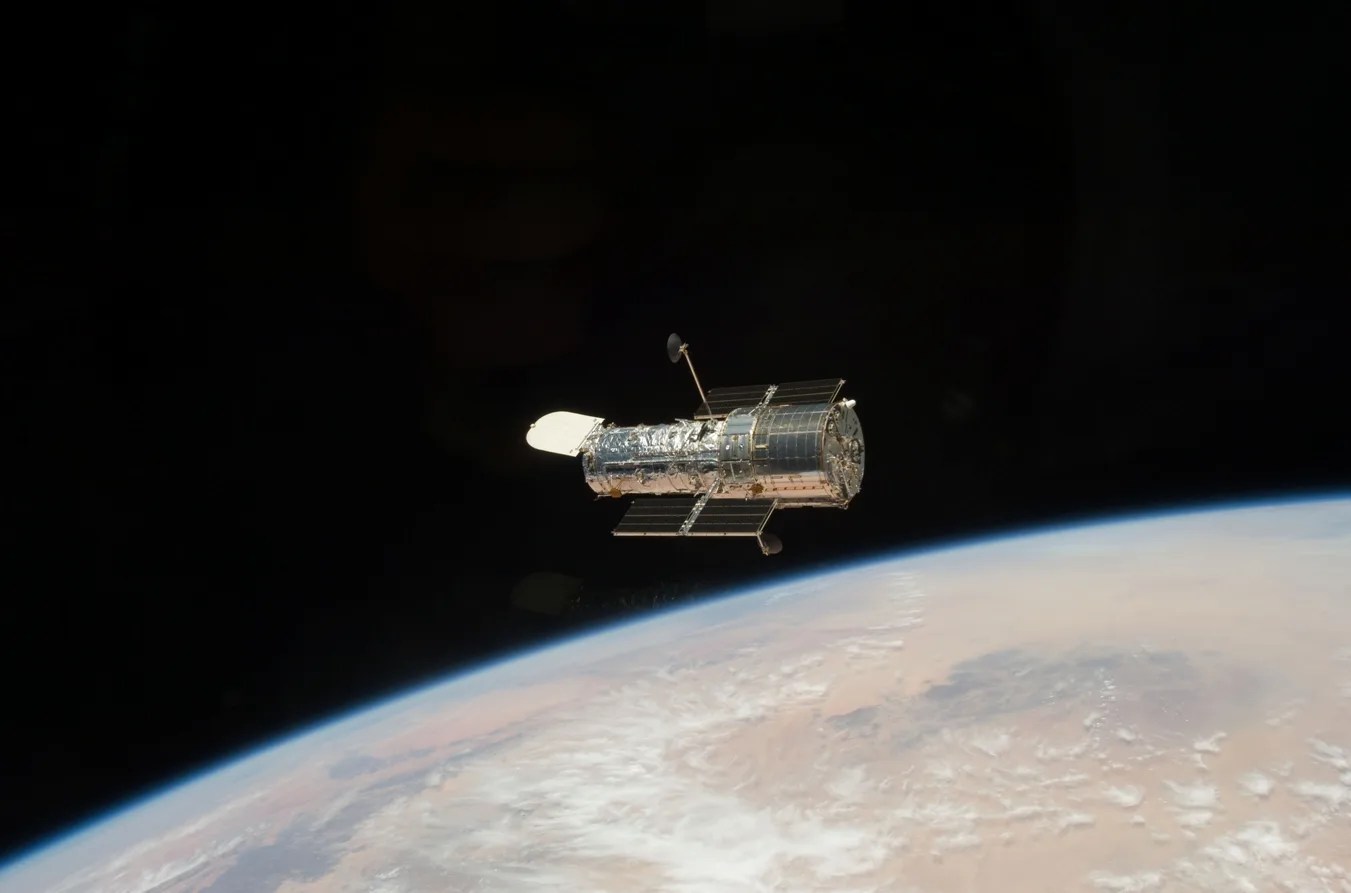
NASA’s Hubble Pauses Science Due to Gyro Issue
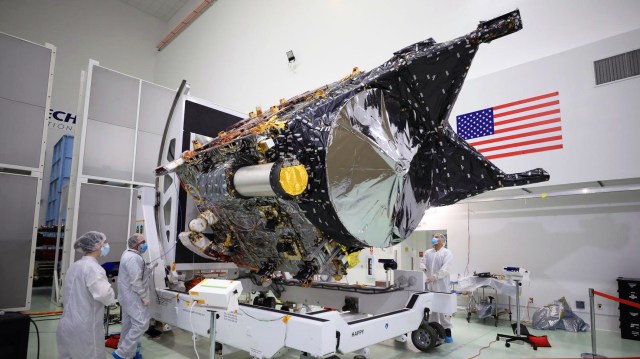
NASA’s Optical Comms Demo Transmits Data Over 140 Million Miles
- Search All NASA Missions
- A to Z List of Missions
- Upcoming Launches and Landings
- Spaceships and Rockets
- Communicating with Missions
- James Webb Space Telescope
- Hubble Space Telescope
- Why Go to Space
- Astronauts Home
- Commercial Space
- Destinations
- Living in Space
- Explore Earth Science
- Earth, Our Planet
- Earth Science in Action
- Earth Multimedia
- Earth Science Researchers
- Pluto & Dwarf Planets
- Asteroids, Comets & Meteors
- The Kuiper Belt
- The Oort Cloud
- Skywatching
- The Search for Life in the Universe
- Black Holes
- The Big Bang
- Dark Energy & Dark Matter
- Earth Science
- Planetary Science
- Astrophysics & Space Science
- The Sun & Heliophysics
- Biological & Physical Sciences
- Lunar Science
- Citizen Science
- Astromaterials
- Aeronautics Research
- Human Space Travel Research
- Science in the Air
- NASA Aircraft
- Flight Innovation
- Supersonic Flight
- Air Traffic Solutions
- Green Aviation Tech
- Drones & You
- Technology Transfer & Spinoffs
- Space Travel Technology
- Technology Living in Space
- Manufacturing and Materials
- Science Instruments
- For Kids and Students
- For Educators
- For Colleges and Universities
- For Professionals
- Science for Everyone
- Requests for Exhibits, Artifacts, or Speakers
- STEM Engagement at NASA
- NASA's Impacts
- Centers and Facilities
- Directorates
- Organizations
- People of NASA
- Internships
- Our History
- Doing Business with NASA
- Get Involved
- Aeronáutica
- Ciencias Terrestres
- Sistema Solar
- All NASA News
- Video Series on NASA+
- Newsletters
- Social Media
- Media Resources
- Upcoming Launches & Landings
- Virtual Events
- Sounds and Ringtones
- Interactives
- STEM Multimedia
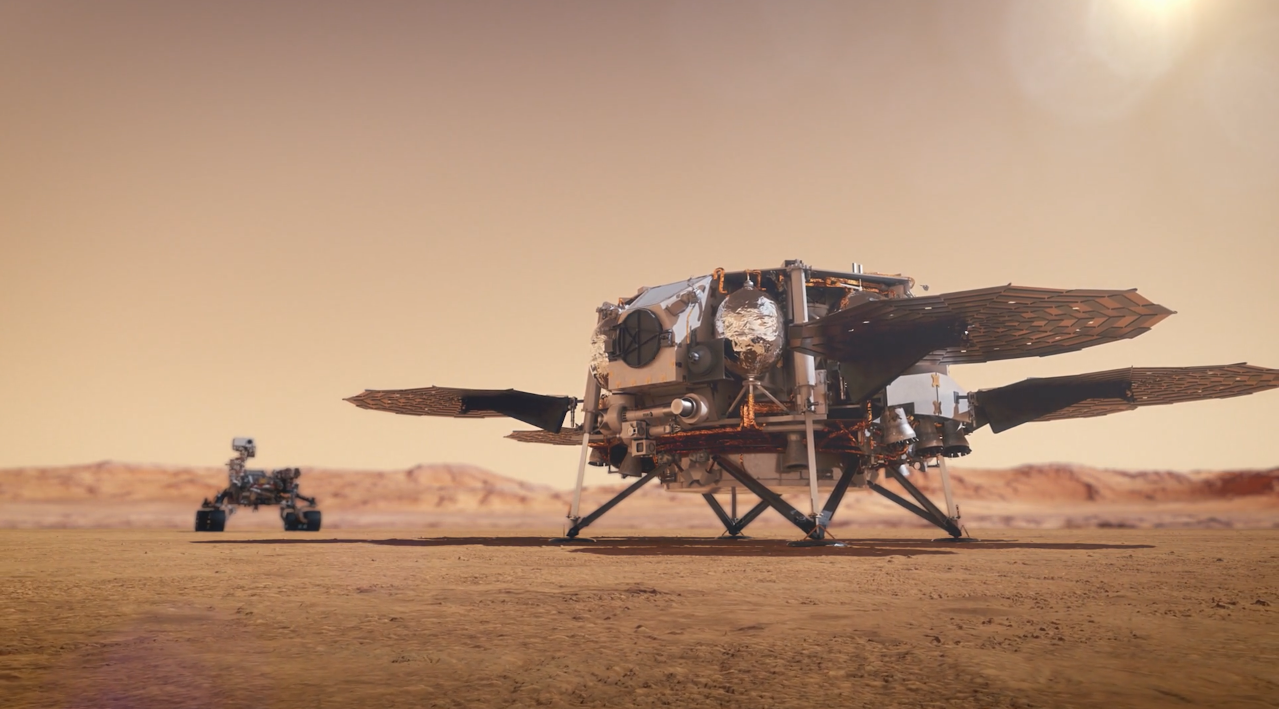
Correction and Clarification of C.26 Rapid Mission Design Studies for Mars Sample Return
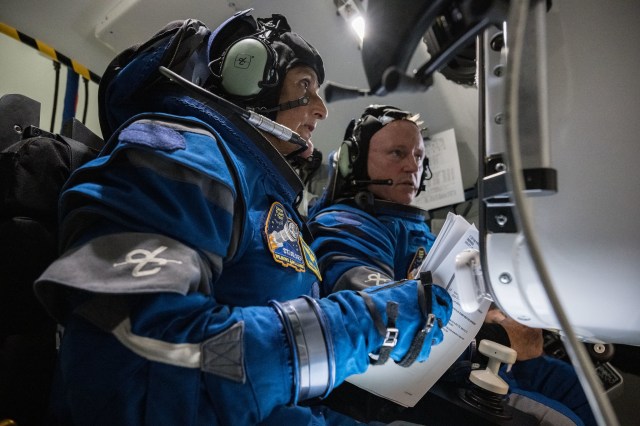
NASA’s Commercial Partners Deliver Cargo, Crew for Station Science
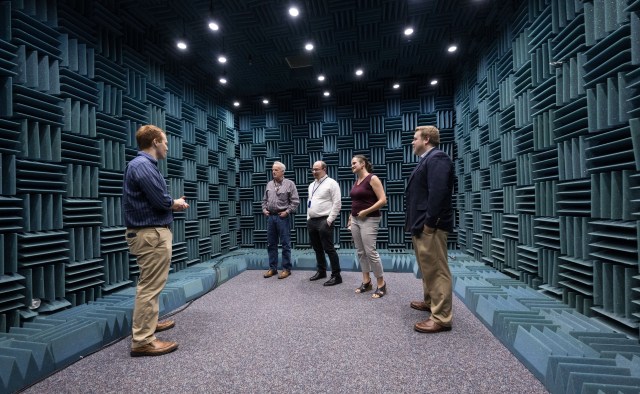
NASA Shares Lessons of Human Systems Integration with Industry

Work Underway on Large Cargo Landers for NASA’s Artemis Moon Missions
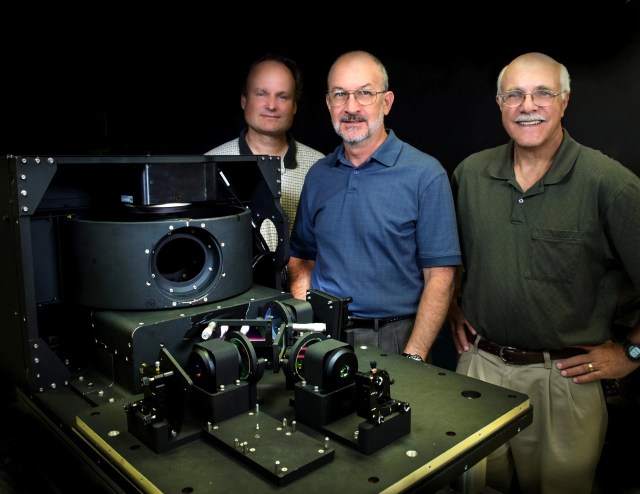
NASA’s ORCA, AirHARP Projects Paved Way for PACE to Reach Space
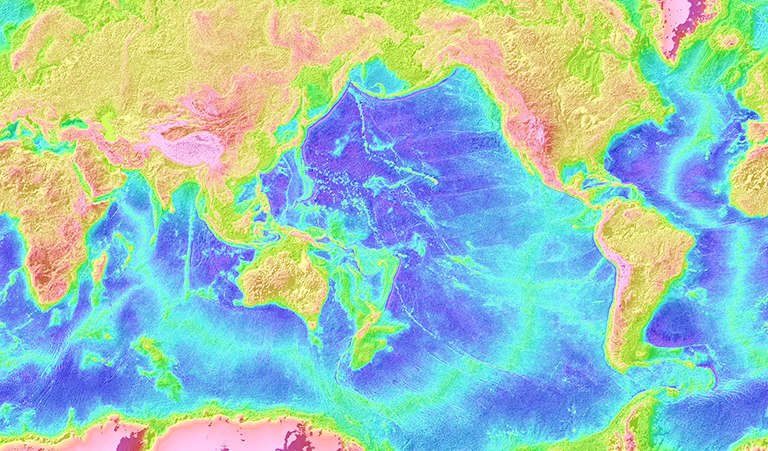
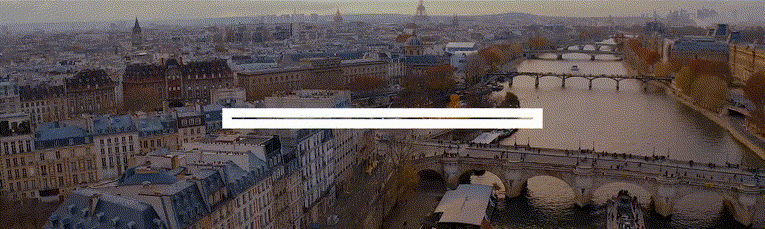
Amendment 11: Physical Oceanography not solicited in ROSES-2024
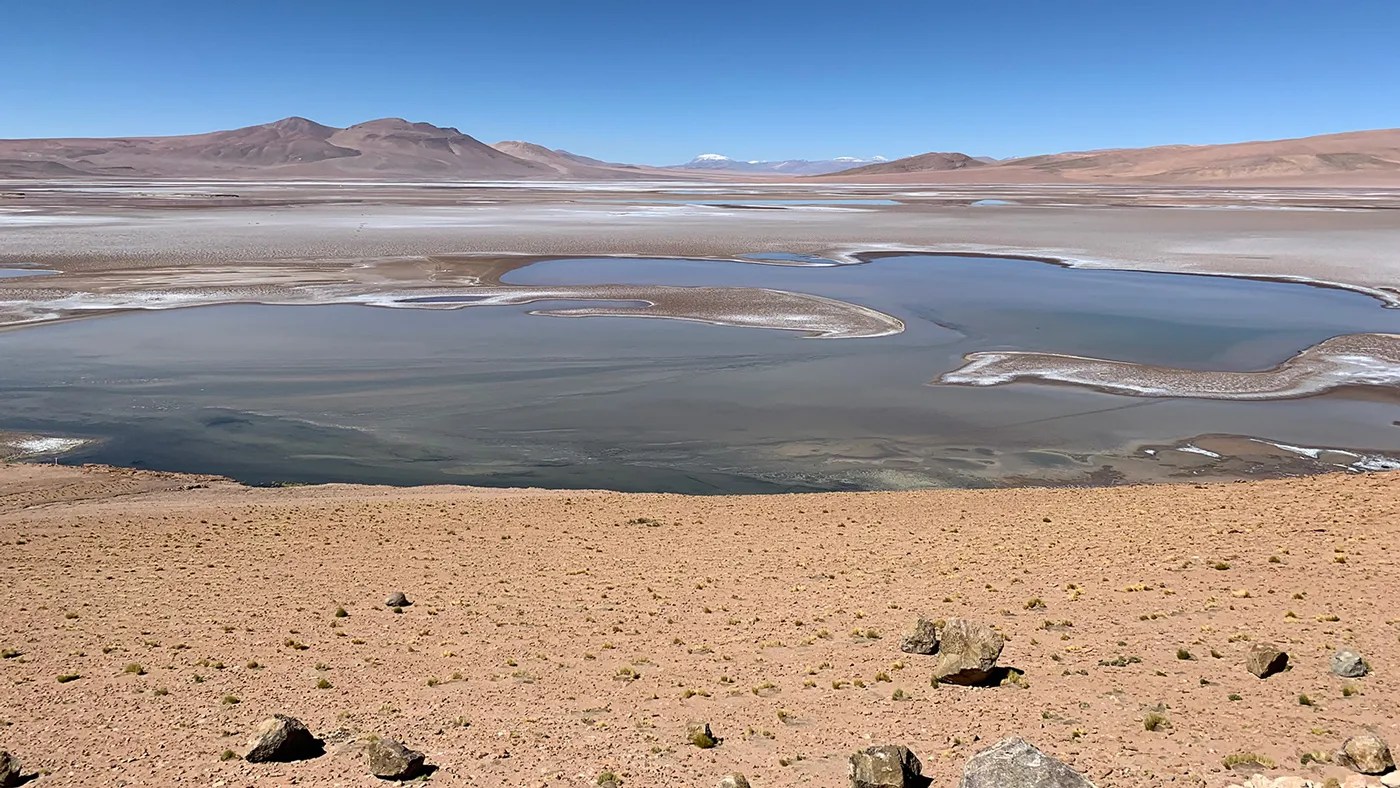
Why is Methane Seeping on Mars? NASA Scientists Have New Ideas
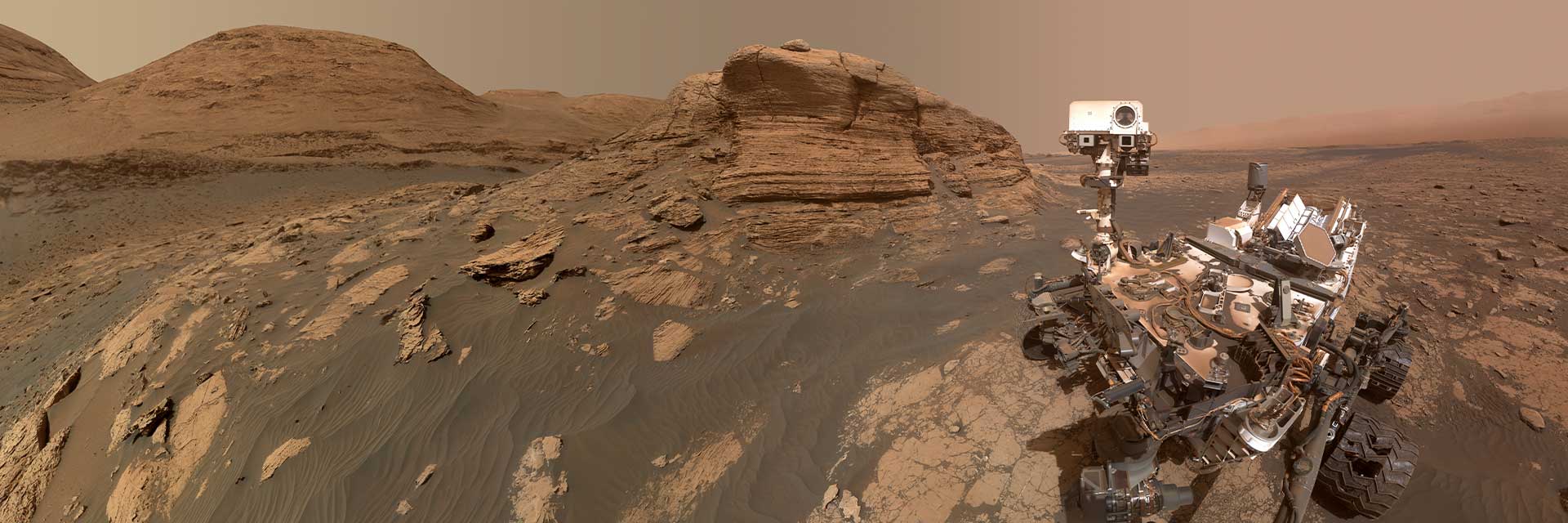
Mars Science Laboratory: Curiosity Rover
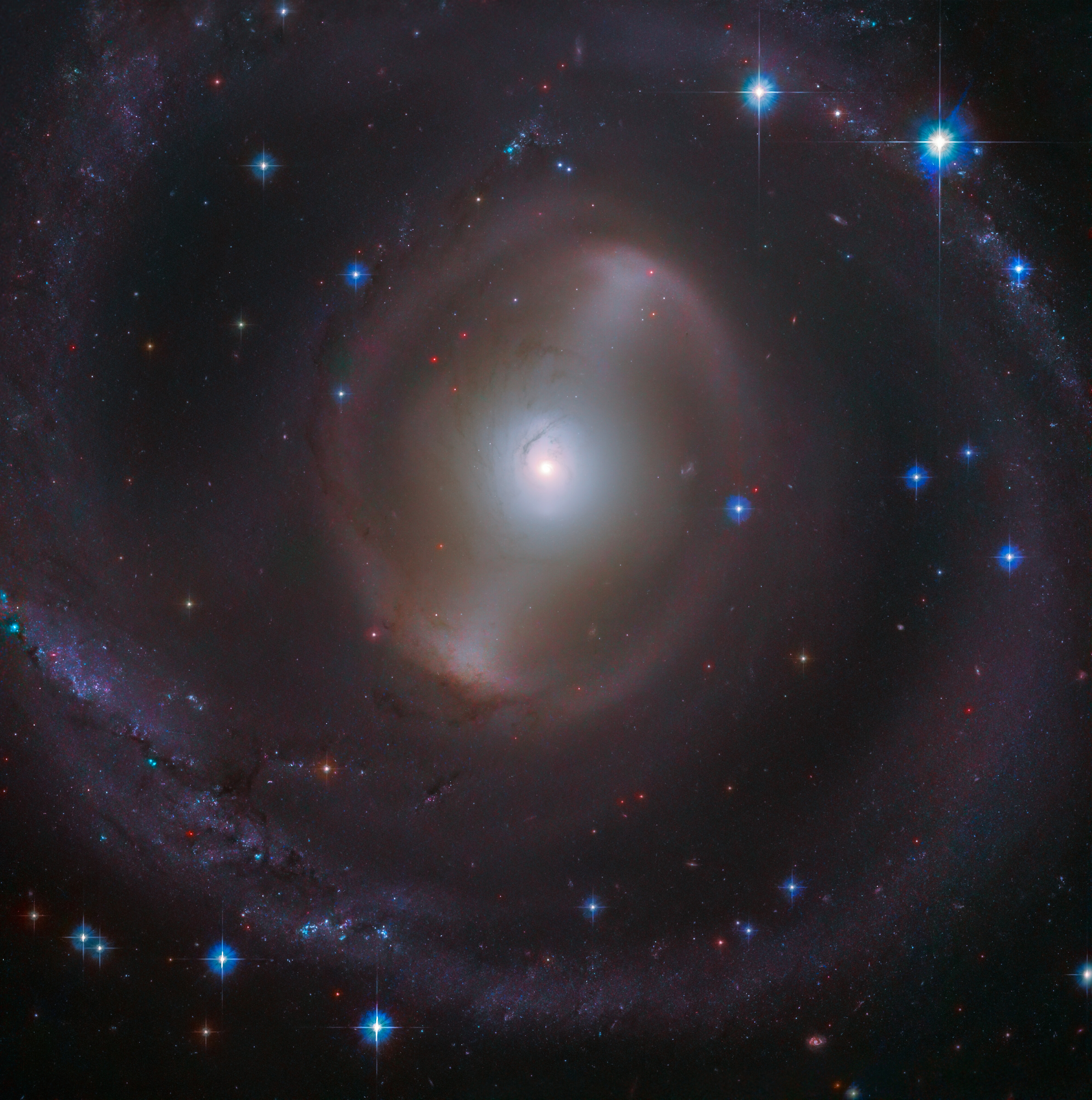
Hubble Spots a Magnificent Barred Galaxy
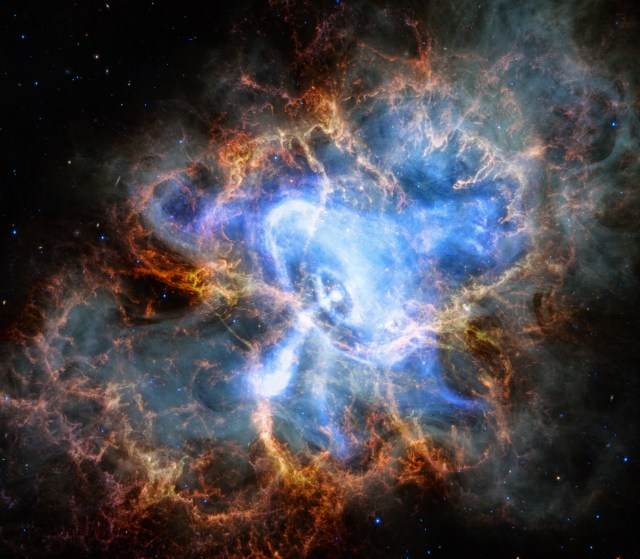
NASA’s Chandra Releases Doubleheader of Blockbuster Hits
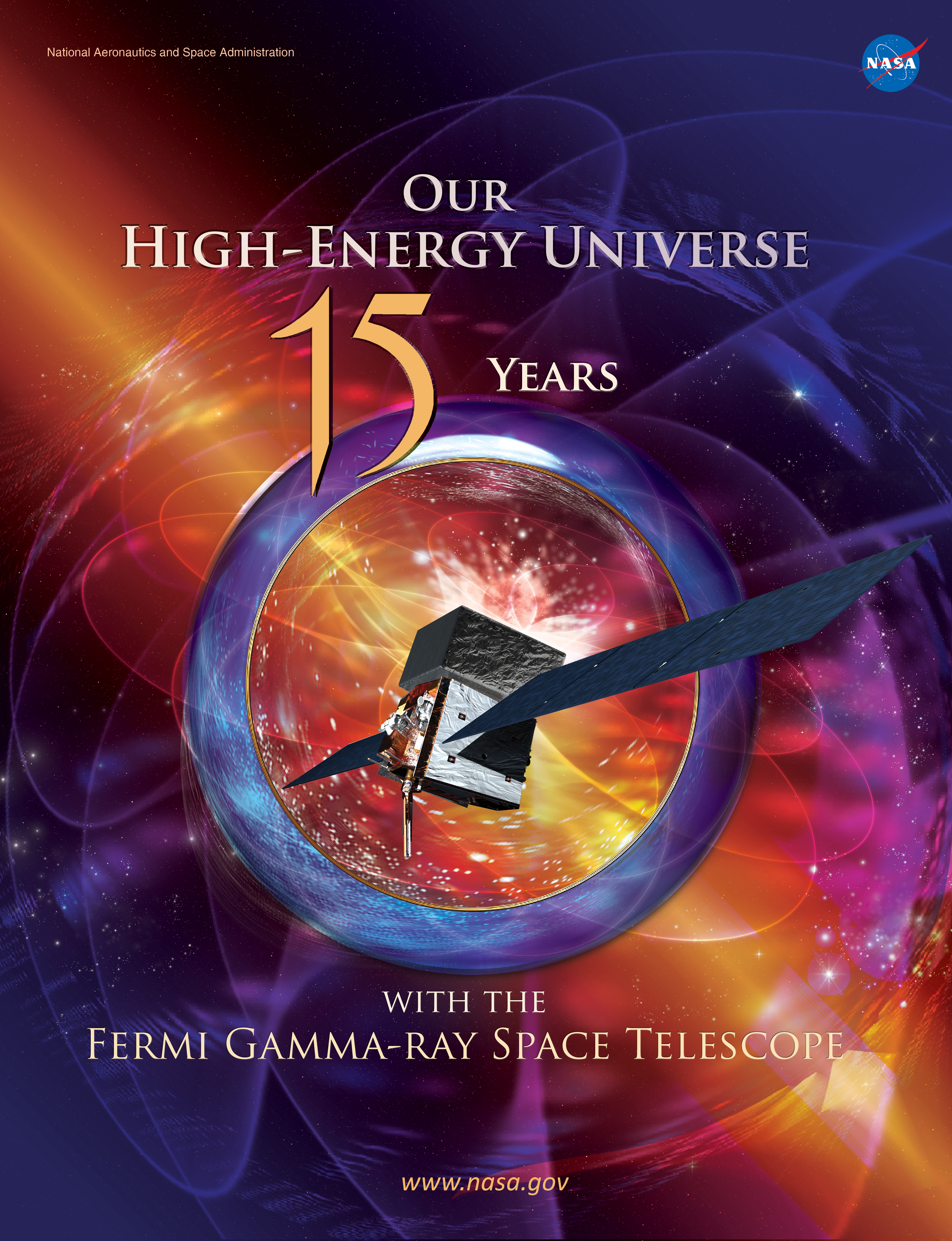
Explore the Universe with the First E-Book from NASA’s Fermi
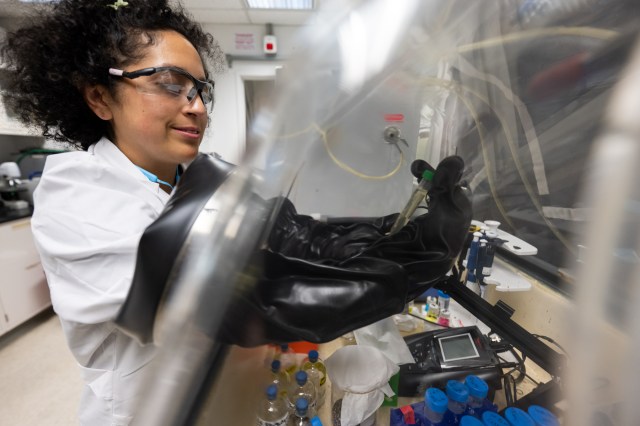
NASA Grant Brings Students at Underserved Institutions to the Stars
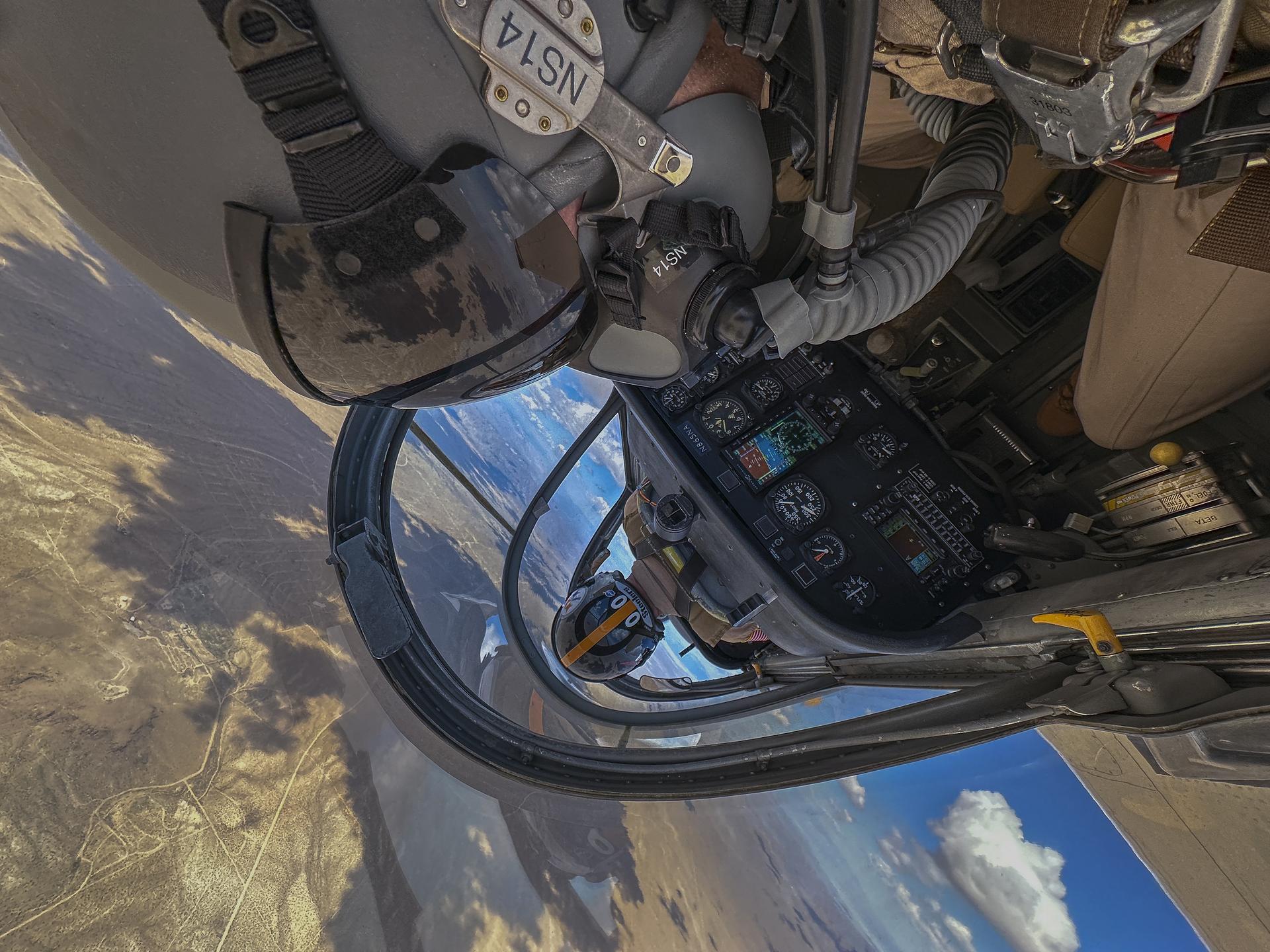
NASA Photographer Honored for Thrilling Inverted In-Flight Image
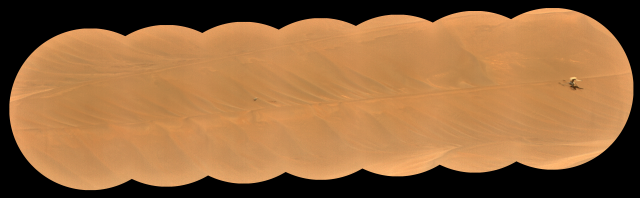
NASA’s Ingenuity Mars Helicopter Team Says Goodbye … for Now
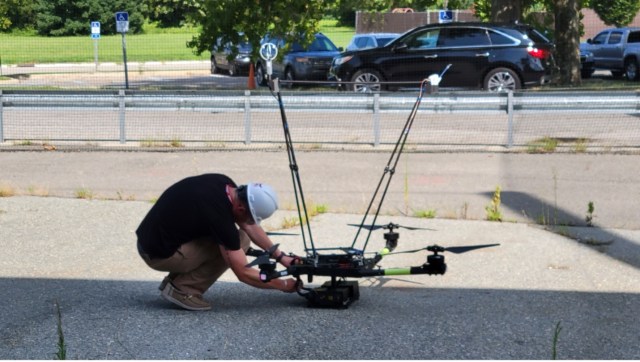
NASA Langley Team to Study Weather During Eclipse Using Uncrewed Vehicles
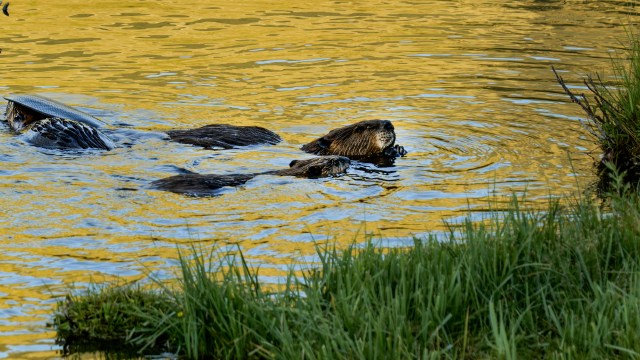
NASA Data Helps Beavers Build Back Streams
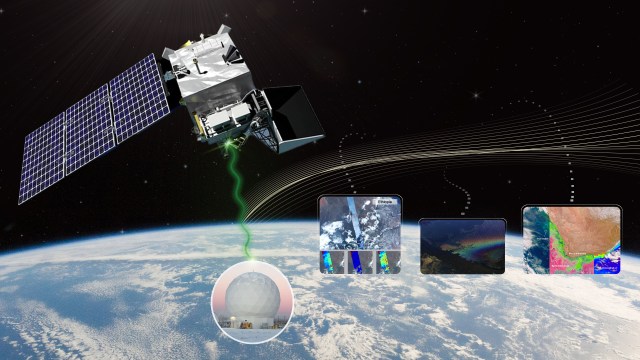
NASA’s Near Space Network Enables PACE Climate Mission to ‘Phone Home’
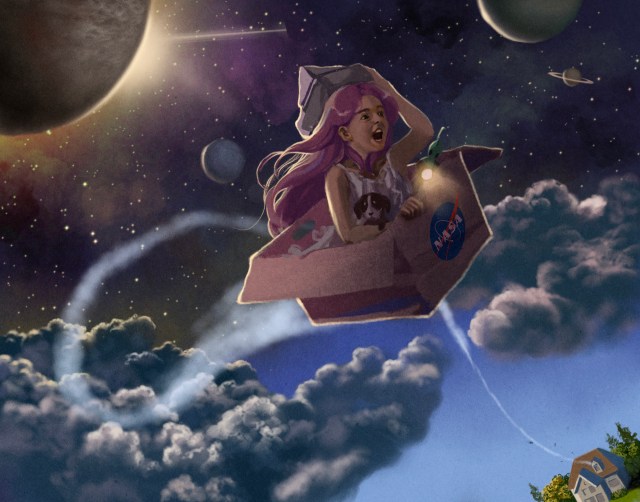
Washington State High Schooler Wins 2024 NASA Student Art Contest
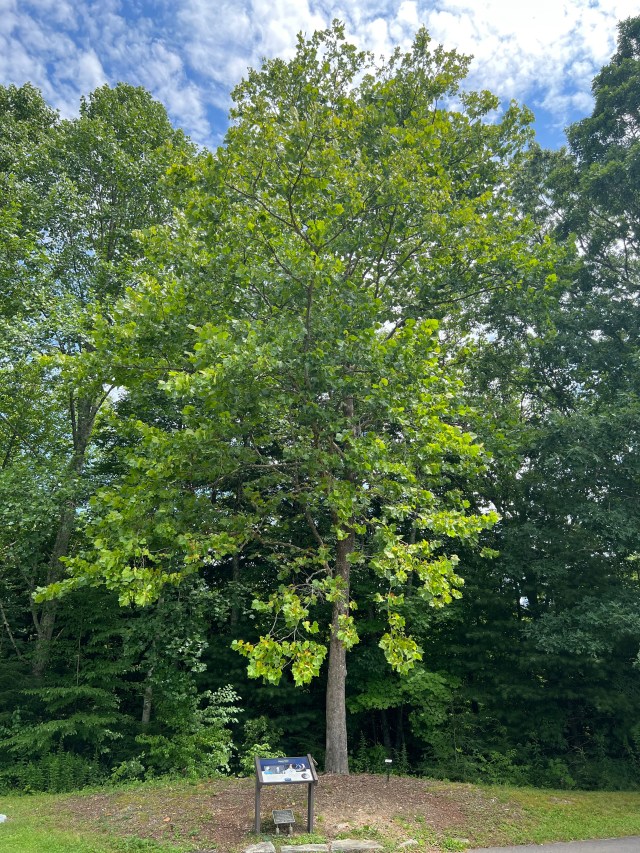
NASA STEM Artemis Moon Trees
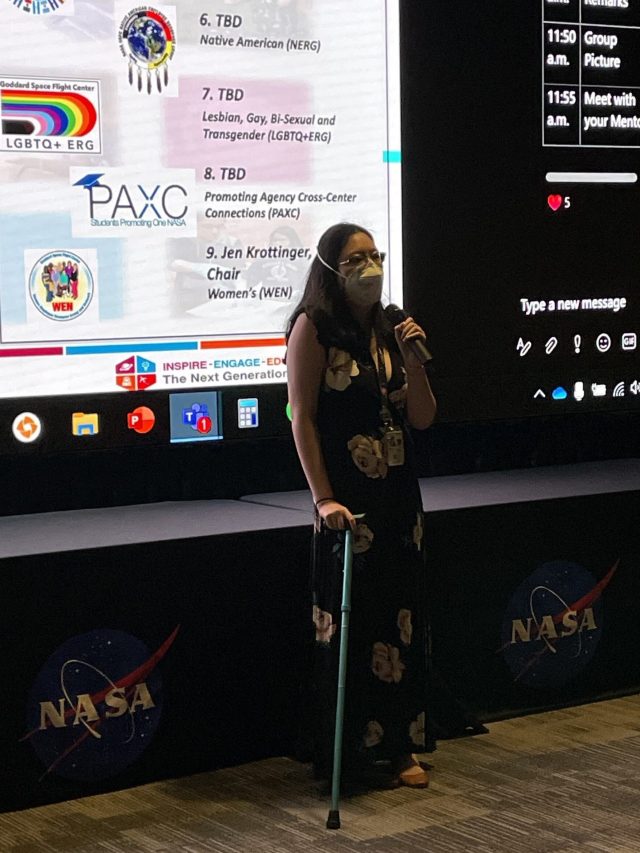
Kiyun Kim: From Intern to Accessibility Advocate
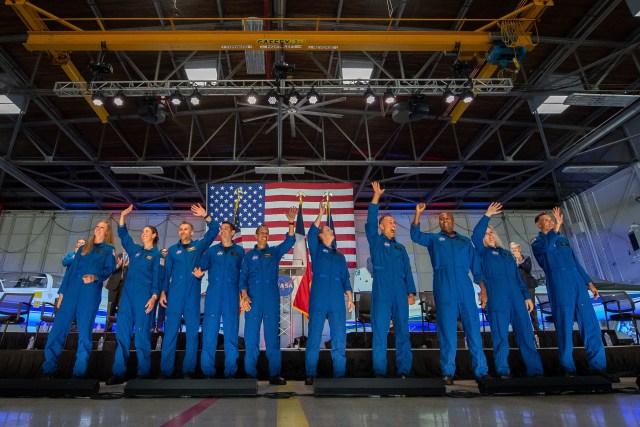
Diez maneras en que los estudiantes pueden prepararse para ser astronautas
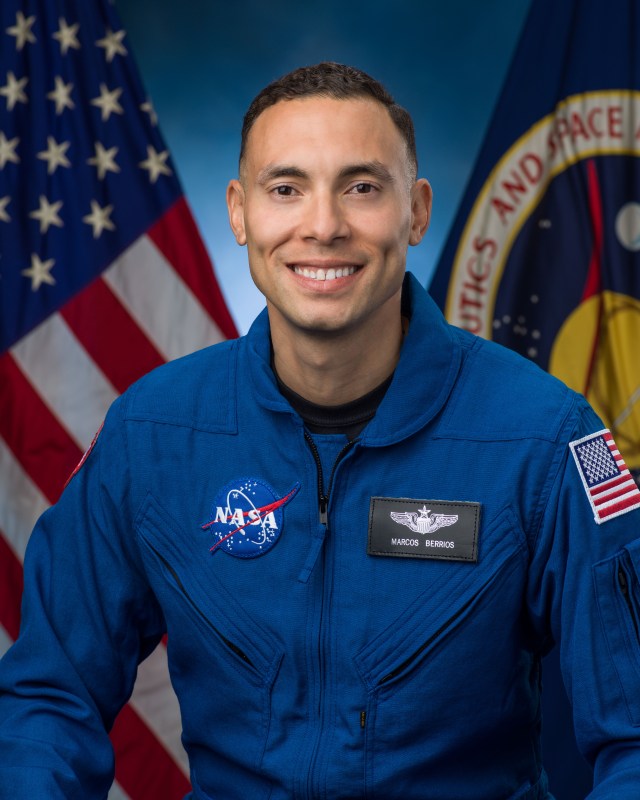
Astronauta de la NASA Marcos Berríos
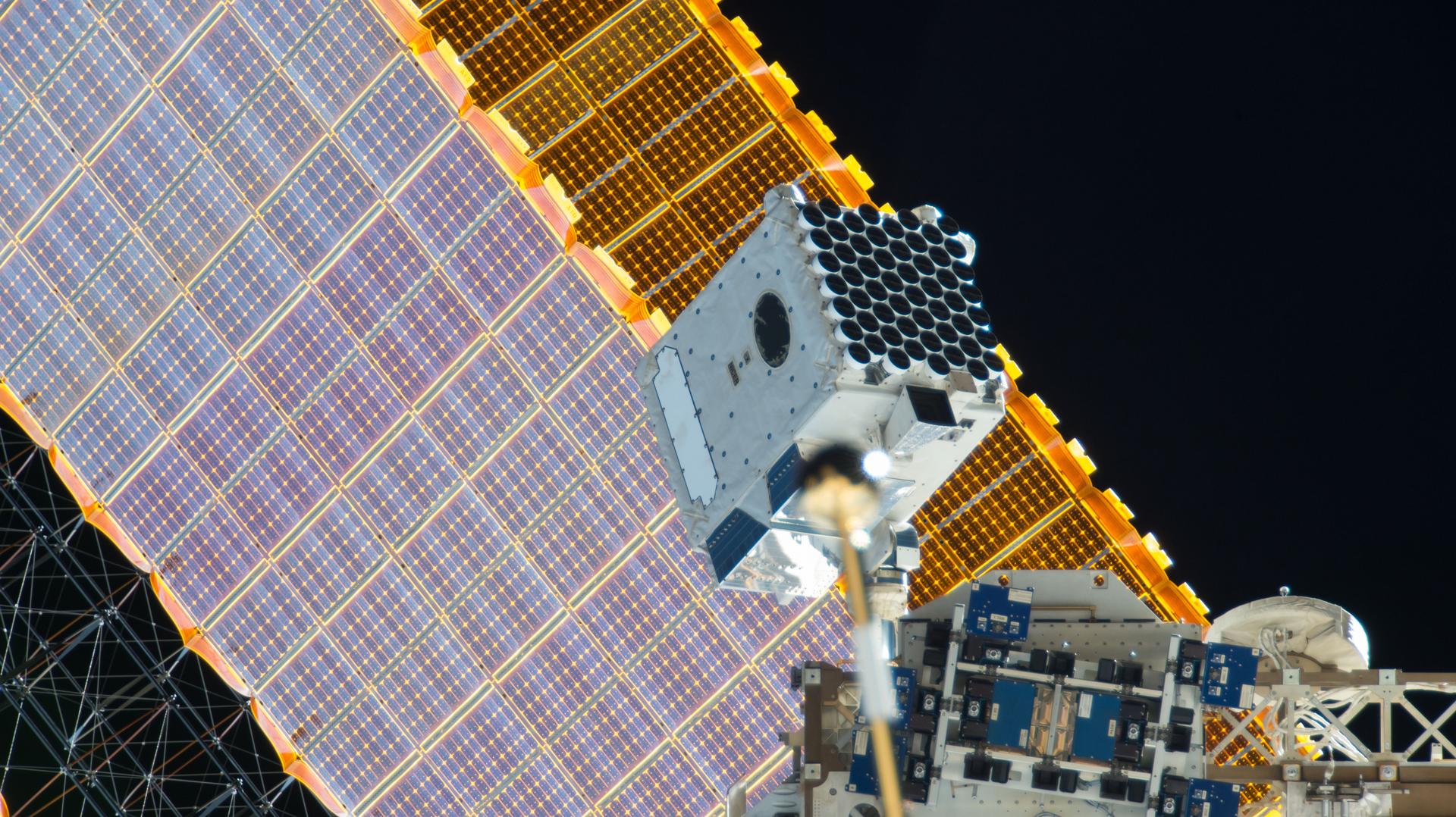
Resultados científicos revolucionarios en la estación espacial de 2023
Nasa looks back at 50 years of gamma-ray burst science.
Francis Reddy
Fifty years ago, on June 1, 1973, astronomers around the world were introduced to a powerful and perplexing new phenomenon called GRBs (gamma-ray bursts). Today sensors on orbiting satellites like NASA’s Swift and Fermi missions detect a GRB somewhere in the sky about once a day on average. Astronomers think the bursts arise from catastrophic occurrences involving stars in distant galaxies, events thought to produce new black holes.
“I can still remember the excitement when gamma-ray bursts were discovered,” said Charles Meegan, a research scientist at the University of Alabama, Huntsville , who helped develop GRB detectors on NASA’s Compton and Fermi satellites. “I was a graduate student then, unaware that the study of these strange events would be my career for the next 50 years.”
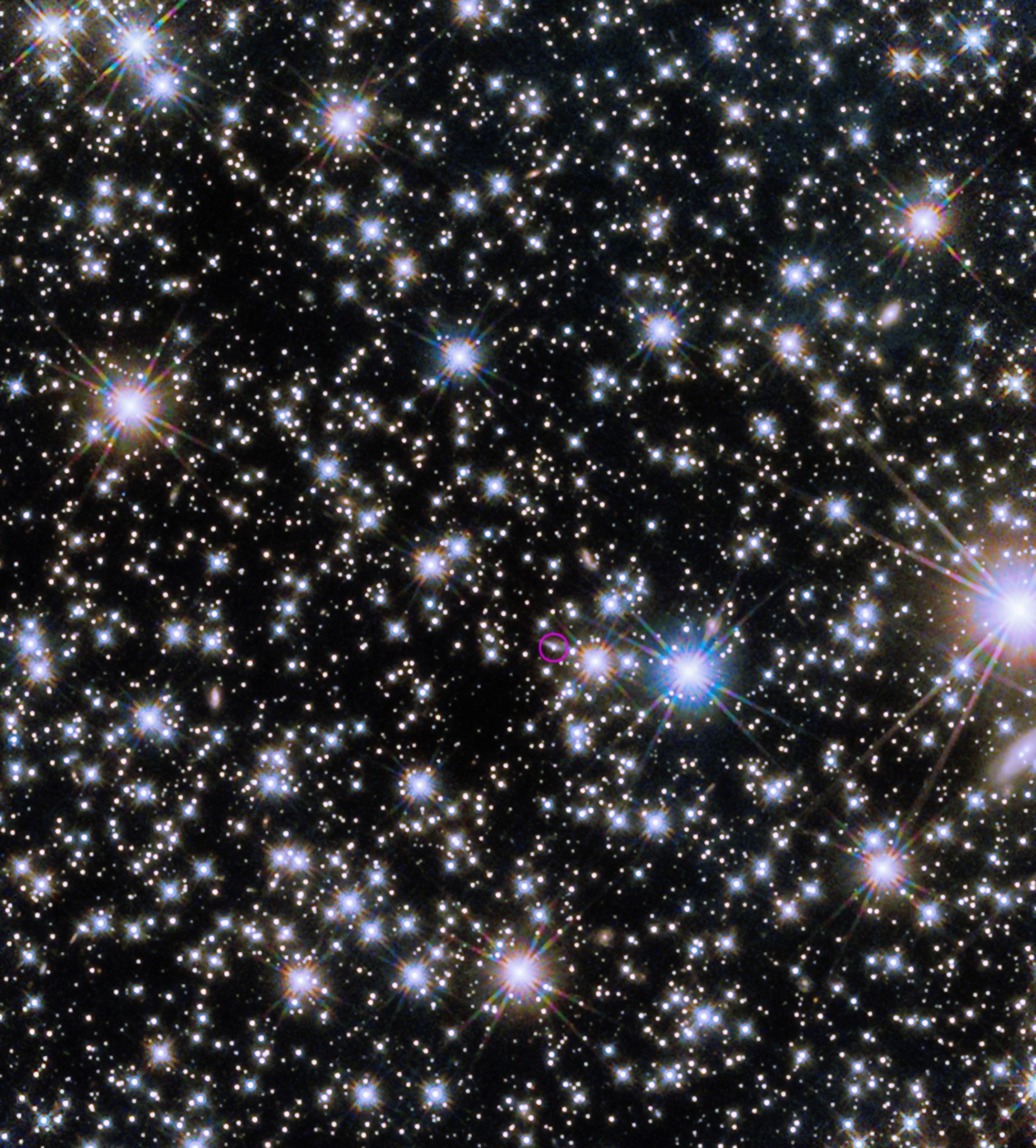
Far-Flung Flare-Ups
With GRBs, just about everything is extreme. They occur so far beyond our galaxy that even the closest-known burst exploded more than 100 million light-years away. Each burst produces an initial pulse of gamma rays, the highest-energy form of light, that typically lasts from milliseconds to minutes. This emission comes from a jet of particles moving close to the speed of light launched in our direction, and the closer we are to looking straight down the barrel, the brighter it appears. Following this prompt emission is a fading afterglow of gamma rays, X-rays, ultraviolet, visible, infrared, and radio light that astronomers may be able to track for hours to months.
Even half a century on, GRBs offer up surprises. One recent burst was so bright it temporarily blinded most of the gamma-ray detectors in space. Nicknamed the BOAT (for brightest of all time), the 7-minute blast may have been the brightest GRB in the past 10,000 years. It also showed that scientists’ most promising models of these events are nowhere near complete.
Nuke Watchers
The GRB story begins in October 1963, when a treaty signed by the United States, the United Kingdom, and the Soviet Union prohibiting the testing of nuclear weapons in the atmosphere, under water, or in space went into effect. To ensure compliance, the U.S. Air Force had been managing an unclassified research and development effort to detect nuclear tests from space. A week after the treaty went into effect, the first two of these satellites, called Vela (from the Spanish “to watch”), began their work.
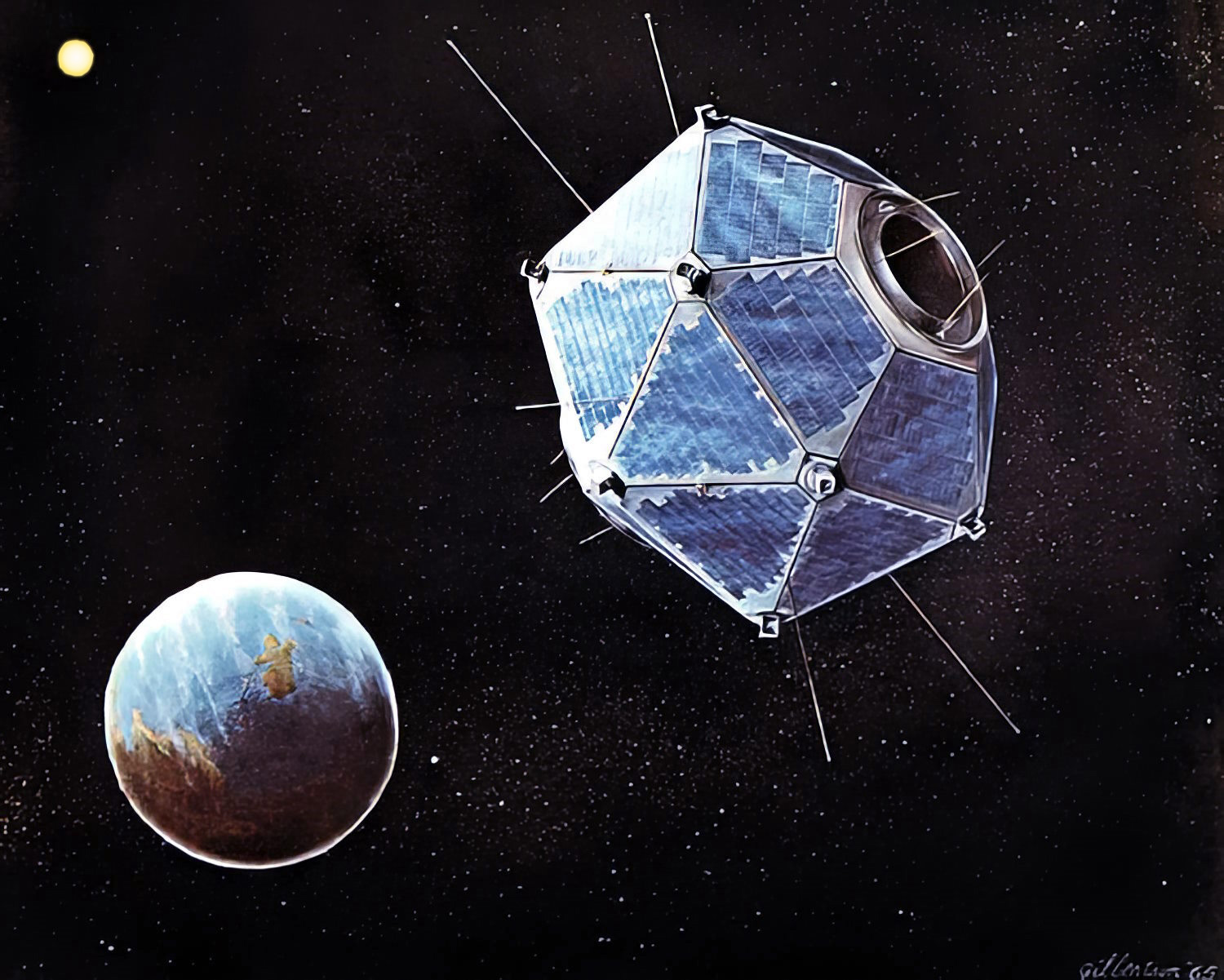
Launched in pairs, the Vela satellites carried detectors designed to sense the initial flash of X-rays and gamma rays from nuclear explosions. Sometimes they triggered on events that clearly were not nuclear tests, and scientists collected and studied these observations. With improved instruments on the four Vela 5 and 6 satellites, Ray Klebesadel at Los Alamos National Laboratory in New Mexico, together with his colleagues Ian Strong and Roy Olsen, determined directions to 16 confirmed gamma-ray events well enough to rule out Earth and the Sun as sources. They published a paper announcing the discovery in The Astrophysical Journal on June 1, 1973.
Using a detector aboard the IMP 6 satellite intended to study solar flares, Tom Cline and Upendra Desai at NASA’s Goddard Space Flight Center in Greenbelt, Maryland, quickly confirmed the Vela findings.
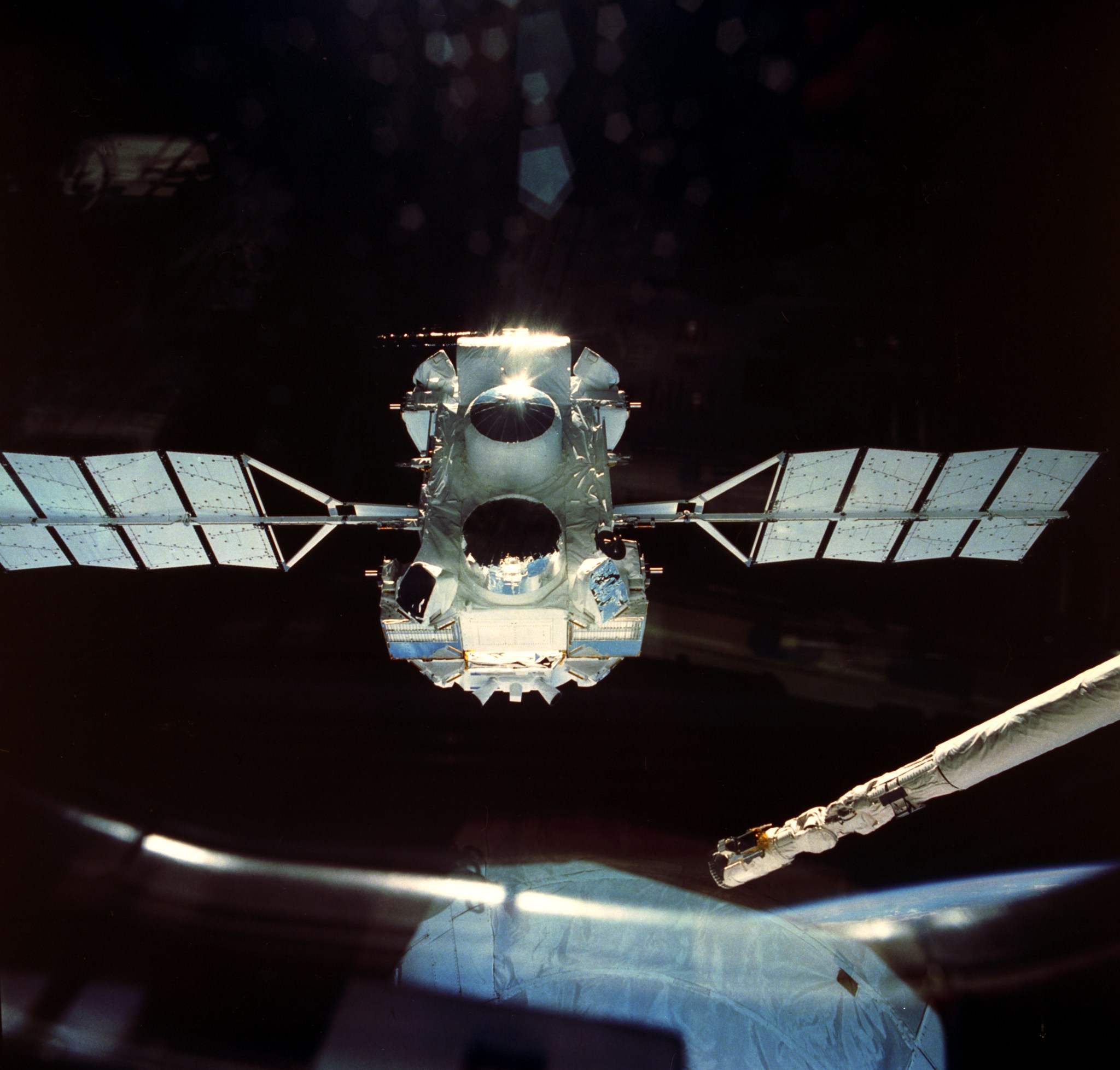
Breakthroughs: BATSE & BeppoSAX
While theorists proposed 100 models in an effort to explain GRBs – most involving neutron stars in our own galaxy – observational progress was slow despite the growing number of detections by different spacecraft. Gamma rays can’t be focused like visible light or X-rays, making precise localizations quite difficult. Without them, it was impossible to search for GRB counterparts in other wavelengths using larger telescopes in space or on the ground.
In 1991, NASA launched the Compton Gamma Ray Observatory , which included an instrument named BATSE (Burst and Transient Science Experiment) dedicated to exploring GRBs. Developed at NASA’s Marshall Space Flight Center in Huntsville, Alabama, by a team that included Meegan, BATSE was about 10 times more sensitive than previous GRB detectors. Over Compton’s nine-year mission, BATSE detected 2,704 bursts, which gave astronomers a rich set of observations made with the same instrument.
In its first year, BATSE data showed that bursts were distributed all over the sky instead of in a pattern that reflected the structure of our Milky Way galaxy. “This suggested that they were coming from distant galaxies, and that meant they were more energetic than most scientists thought possible,” Meegan said.
Around the same time, Chryssa Kouveliotou, another member of the BATSE team, led an effort to classify the bursts. The team found that burst durations clustered into two broad groups – one lasting less than two seconds, the other lasting longer than two seconds – and that short bursts produced higher-energy gamma rays than long ones.
“So both temporal and spectral properties agreed in identifying two separate groups of GRBs: short and long,” said Kouveliotou, who now chairs the department of physics at George Washington University . “Soon after, theorists associated long GRBs with the collapse of massive stars and short ones with binary neutron star mergers .”
The next step in understanding came with watershed observations from the Italian-Dutch satellite BeppoSAX . Although not specifically designed as a GRB mission, its mix of instruments – including a gamma-ray monitor and two wide-field X-ray cameras – proved a boon to the field.
When a burst occurred in the field of view of one of the X-ray cameras, the spacecraft could locate it well enough over a couple of hours that additional instruments could be brought to bear. Whenever BeppoSAX turned to a GRB’s position, its instruments found a rapidly fading and previously unknown high-energy source – the X-ray afterglow theorists had predicted. These positions enabled large ground-based observatories to discover long GRB afterglows in visible light and radio waves, and also permitted the first distance measurements, confirming that GRBs were truly far-away events.
Need for Speed
In 2000, NASA launched HETE 2 , a small satellite designed to detect and localize GRBs. It was the first mission to compute accurate positions onboard and quickly – in tens of seconds – communicate them to the ground so other observatories could study early afterglow phases. The burst it discovered on March 29, 2003, also exhibited definitive supernova characteristics, confirming a suspected relationship between the two phenomena.

What took BeppoSAX a couple of hours, NASA’s Neil Gehrels Swift Observatory, launched in 2004, can do in about a minute. “We named it Swift for a reason,” said Goddard’s S. Bradley Cenko, the mission’s current principal investigator. “Its rapid, automated response allowed us to detect flares and other features in X-ray afterglows not previously seen.”
Following up on GRBs detected by these missions confirmed that long bursts were associated with the star-forming regions of galaxies and were often accompanied by supernovae. In May 2005, Swift was able to pinpoint the first afterglow of a short GRB , showing that these blasts occur in regions with little star formation. This bolstered the model of short bursts as mergers of neutron stars, which can travel far from their birth place over the many millions of years it takes for them to crash together.
In 2008, NASA’s Fermi Gamma-ray Space Telescope joined Swift in hunting GRBs and has observed about 3,500 to date. Its GBM (Gamma-ray Burst Monitor) and Large Area Telescope allow the detection and follow-up of bursts from X-rays to the highest-energy gamma rays detected in space – an energy span of 100 million times. This has enabled the discovery of afterglow gamma rays with billions of times the energy of visible light.
The Next Revolution
In 2017, Fermi and the European INTEGRAL satellite linked a short GRB to a source of gravitational waves , ripples in space-time produced as orbiting neutron stars spiraled inward and merged. This was an important first that connected two different cosmic “messengers,” gravity and light. While astronomers haven’t seen another “gravity and light” burst since, they hope more will turn up in current and future observing runs of gravitational wave observatories.
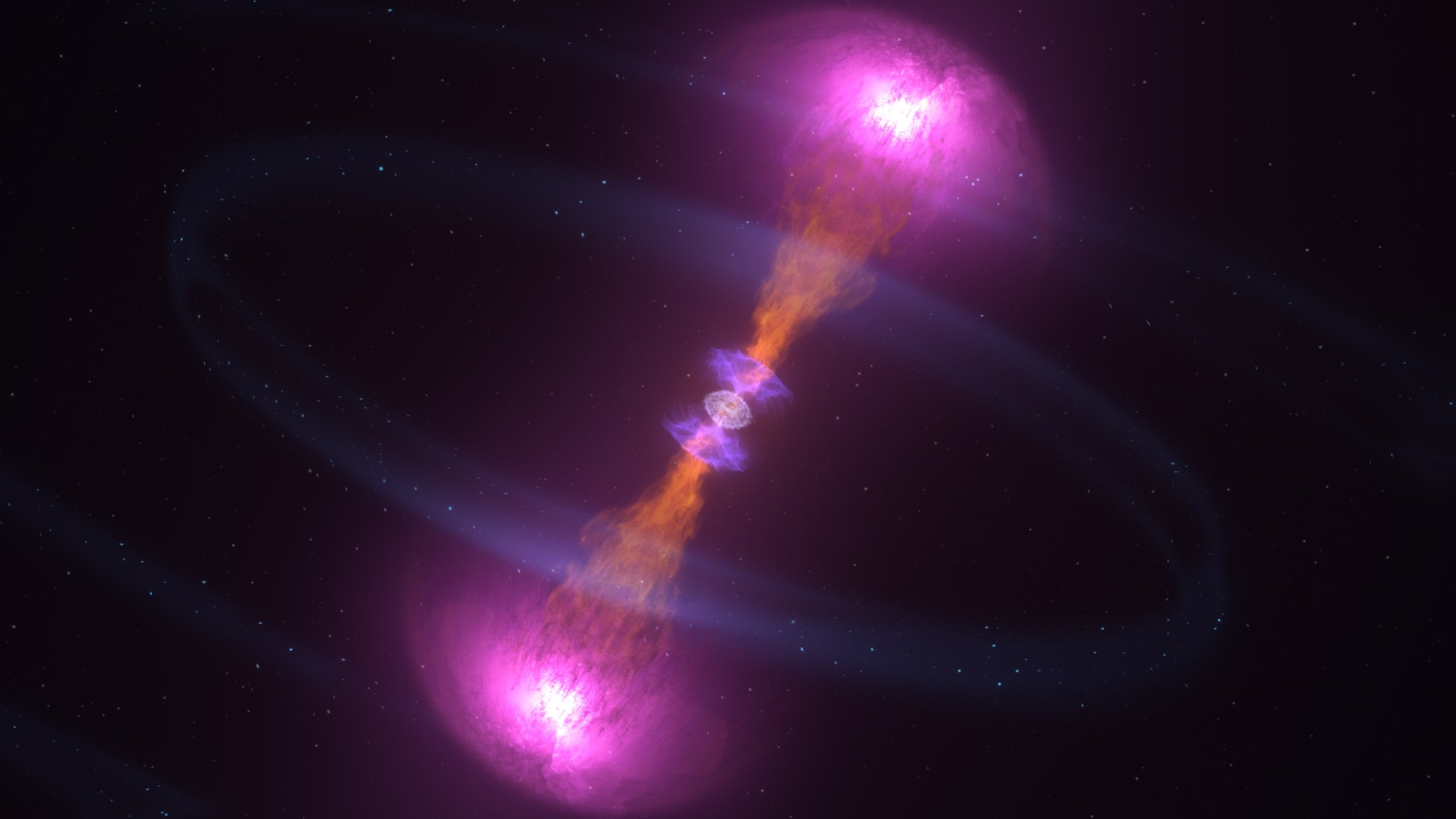
“We’re building new satellites with greater sensitivity to delve more deeply into this phenomenon, so the future of GRB science is bright,” said Marshall’s Dan Kocevski, a member of the Fermi GBM team and the principal investigator for StarBurst , a small satellite designed to explore GRBs from neutron star mergers. Other missions include Glowbug, part of an experiment package launched to the International Space Station in March and led by J. Eric Grove at the U.S. Naval Research Laboratory in Washington; BurstCube , led by Goddard’s Jeremy Perkins and slated for launch in early 2024; MoonBEAM , which would orbit between Earth and the Moon and is led by Marshall’s Chiumun Michelle Hui; and LEAP , designed to study GRB jets from the space station, led by Mark McConnell at the University of New Hampshire, Durham .
And as gravitational and gamma-ray facilities both improve their reach, a new chapter of the GRB story will open.
“What will completely revolutionize our understanding of GRBs,” said Alessandra Corsi, an associate professor at Texas Tech University in Lubbock, “will be the ability to track them back to when the universe was most intensely forming stars, around 10 billion years ago. This part of the universe will be probed by the next generation of gravitational wave detectors – 10 times more sensitive than what we currently have – and by future gamma-ray missions that can ensure continuity with the fantastic science Swift and Fermi have enabled.”
By Francis Reddy NASA’s Goddard Space Flight Center , Greenbelt, Md.
Media Contact: Claire Andreoli NASA’s Goddard Space Flight Center, Greenbelt, Md. (301) 286-1940
Related Terms
- Astrophysics
- Compton Gamma Ray Observatory (CGRO)
- Fermi Gamma-Ray Space Telescope
- Gamma-Ray Bursts
- Goddard Space Flight Center
- International Gamma-ray Astrophysics Laboratory (INTEGRAL)
- International Space Station (ISS)
- Marshall Space Flight Center
- Neil Gehrels Swift Observatory
- Science & Research

An official website of the United States government
Here’s how you know
Official websites use .gov A .gov website belongs to an official government organization in the United States.
Secure .gov websites use HTTPS A lock ( Lock A locked padlock ) or https:// means you’ve safely connected to the .gov website. Share sensitive information only on official, secure websites.
JavaScript appears to be disabled on this computer. Please click here to see any active alerts .
- Radioactive Fallout From Nuclear Weapons Testing
- Immediately following an aboveground nuclear explosion, debris and soil can mix with radionuclides. This mixture is sent up into the air and then falls back to Earth. It is called fallout and it typically contains hundreds of different radionuclides.
- Since the conclusion of the weapons testing in the 1980s, radionuclides in the atmosphere have largely decayed away.
Detonating nuclear weapons aboveground sends radioactive materials as high as 50 miles into the atmosphere. Large particles fall to the ground near the explosion site, but lighter particles and gases travel into the upper atmosphere. The particles that are swept up into the atmosphere and fall back down to Earth are called fallout. The highest particles can circulate around the world for years until they gradually fall to Earth or are brought back to the surface by precipitation. The path of the locations of the fallout depend on wind and weather patterns.
- About Radioactive Fallout From Nuclear Weapons Testing
- Where to learn more
About Radioactive Fallout From Nuclear Weapons Testing
Fallout typically contains hundreds of different radionuclides. Some stay in the environment for a long time because they have long half-lives, like cesium-137, which has a half-life of about 30 years. Most have very short half-lives, so decay away in a few minutes or a few days, for examples iodine-131, has a half-life of 8 days. Very little radioactivity from weapons testing in the 1950s and 1960s can still be detected in the environment now.
The United States conducted the first aboveground nuclear weapon test in southeastern New Mexico on July 16, 1945. Between 1945 and 1963, hundreds of aboveground test took place around the world. Over time the number and size (or yield) of these weapons increased, especially in the late 1950s and early 1960s. After the Limited Test Ban Treaty of 1963 was signed by the United States, the Soviet Union and Great Britain, most aboveground tests ceased. Some aboveground weapons testing by other countries continued until 1980. Since the end of aboveground nuclear weapons testing, the day-to-day radiation in air readings from monitoring sites has fallen. For many years, analysis of air samples has shown risk levels far below regulatory limits. In fact, results are now generally below levels that instruments can detect.
The EPA maintains a system of radiation monitors throughout the United States. These monitors were originally designed to detect radionuclides that were released after a nuclear weapon detonation. Now, the EPA uses this system, called RadNet, to look at background radiation levels at many locations across the United States. Background radiation is around us all the time, mostly from natural sources, like naturally occurring radon and uranium. For more information about the history of RadNet, please visit the Learn About RadNet webpage.
Some of the fallout radionuclides the EPA’s monitoring systems may detect include:
- Americium-241
- Strontium-90
Even though there is very little fallout that still exists in the environment, it is important to remember that recent fallout, within about 10 to 20 miles downwind of the detonation, can be very dangerous. This section talks about the different ways we can be exposed to radiation if a nuclear detonation occurs.
When a nuclear detonation occurs, people, plants, and animals can be exposed to the fallout in several ways. Livestock may eat contaminated plants or drink contaminated water. People who then eat this livestock will then still experience internal contamination, in which radioactive material ends up inside of our bodies, despite not consuming contaminated plants or water directly.
Radionuclides that are inhaled or ingested are not blocked by an external shield. These radionuclides interact with internal cells and tissues, which increases the risk of harmful health effects. When radionuclides can ingested, they can change the structure of cells, which is one of the ways people can develop cancer. The health risks from fallout have been described in many studies. One example is the Federal Radiation Council’s 1962 report, Health Implications of Fallout from Nuclear Weapons Testing through 1961 . This is one of the reasons why radiation protection professionals work hard to protect people from unnecessary exposure to radiation.
The radioactive dust that settles on the environment around us is an example of potential external exposure. Radionuclides that emit alpha and beta particles would pose a lower external exposure threat because they do not travel very far in the atmosphere and are not as penetrating as more energetic radiation. Shielding, one of the three principles of radiation protection, prevents some external exposure because alpha particles are blocked by the dead skin cells that sit on the surface of our bodies. Gamma rays, however, travel much farther in the atmosphere, and are higher energy rays that can only be blocked by heavy shielding, like a concrete wall or a lead apron. These rays pose a higher external exposure risk.
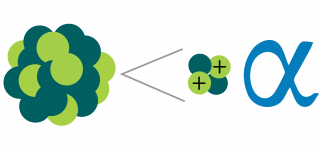
The U.S. State Department
The U.S. State Department negotiated three treaties that govern nuclear weapons testing.
The Treaty on The Limitation Of Underground Nuclear Weapon Tests This treaty is also known as the Threshold Test Ban Treaty. It was signed in July of 1974 by the United States and the Union of Soviet Socialist Republics (USSR). It established a nuclear “threshold,” by prohibiting tests having a yield exceeding 150 kilotons (equal to 150 thousand tons of TNT).
Treaty Banning Nuclear Weapon Tests in The Atmosphere, in Outer Space and Underwater The Test Ban Treaty of 1963 prohibits nuclear weapons tests “or any other nuclear explosion” in the atmosphere, in outer space and under water. It does not ban tests underground. The goal of the treaty is to end radioactive contamination of the environment.
The Comprehensive Nuclear Test-Ban Treaty (CTBT) The CTBT is a legally binding global ban on nuclear explosive testing. The CTBT was opened for signature in 1996. The United States has signed the treaty, but it has not been ratified by the Senate.
The National Archives and Records Administration (NARA)
The National Archives is the U.S. Government’s collection of documents that records important events in American history. The NARA is the Government agency that preserves and maintains these materials and makes them available for research.
Teaching with Documents: Photographs and Pamphlet About Nuclear Fallout This webpage contains a brief description of the nuclear arms race of the 1950s and 1960s. It also provides a 1950s pamphlet about fallout and several pictures related to nuclear weapons testing and fallout shelters.
The U.S. Department of Health and Human Services (HHS), the Centers for Disease Control and Prevention (CDC)
The CDC website provides information about radioactive fallout from nuclear weapons tests conducted in the atmosphere around the world (global weapons testing) during the 1940s and 1950s. The CDC and the National Cancer Institute (NCI) have studied whether it is possible to estimate the health effects to Americans from this global fallout. In the process, the CDC and the NCI were able to make some estimates of how much fallout exposure people received, what some of the possible effects might be, and how frequently the effects might occur.
Radioactive Fallout from Global Weapons Testing This webpage provides information about radioactive fallout from atmospheric nuclear weapons tests conducted during the 1940s and 1950s. It also provides some estimates of how much fallout exposure people received, possible effects, and how frequently the effects might occur.
- RadTown Home
- Neighborhood
- Power Generation
- Depleted Uranium
- Nuclear Submarines and Aircraft Carriers
- Transporting Radioactive Material
- Cleanup Sites
- Classroom Materials for Teachers
- A to Z Subject Index
- Women in Radiation History
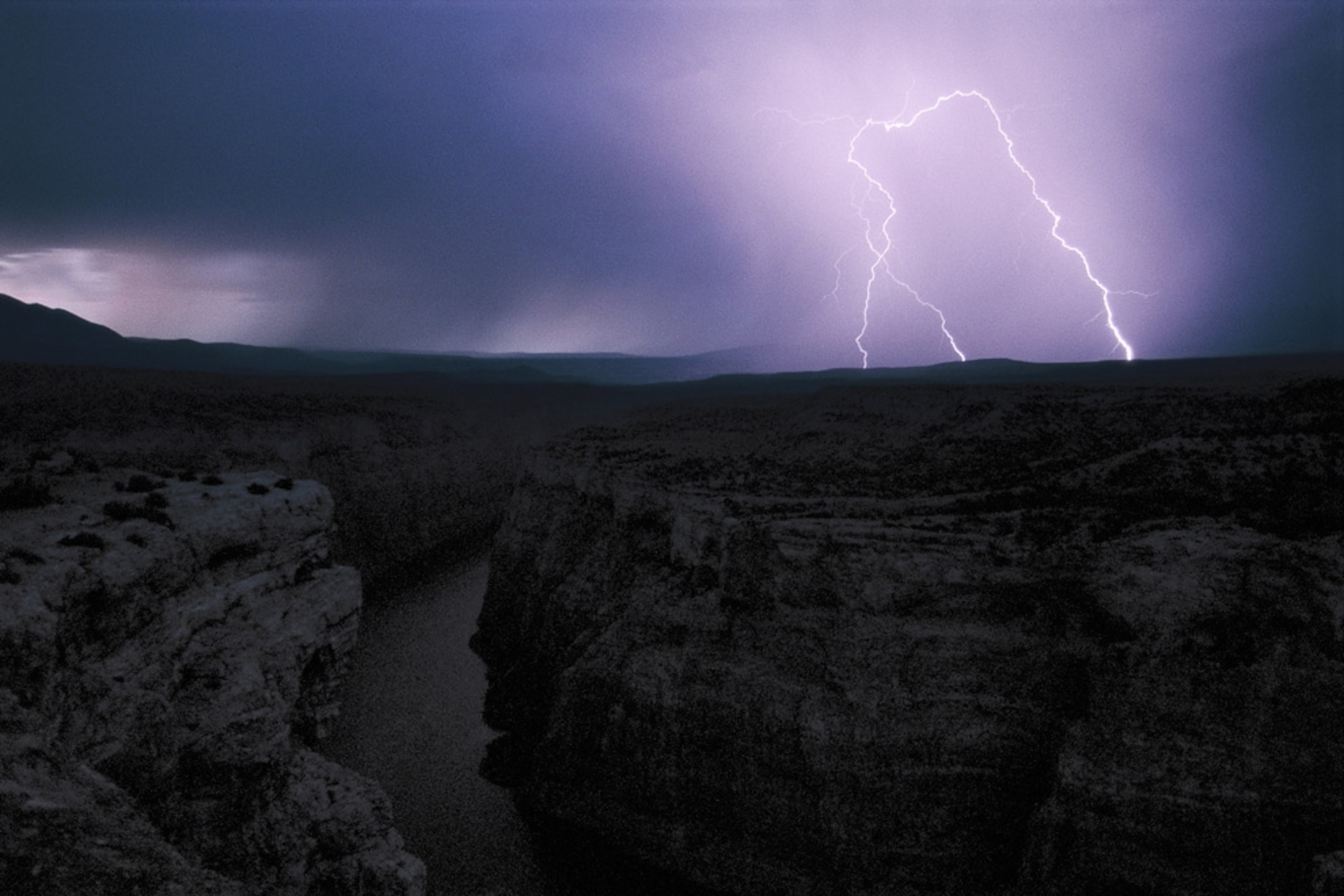
Lightning strikes over Bighorn Canyon National Recreation Area in Montana (file photo).
Gamma Rays a Flight Risk?
Radiation from thunderstorms can equal 400 chest x-rays, study finds.
Airplane passengers flying near thunderstorms could be exposed to dangerously high levels of radiation in the form of terrestrial gamma ray flashes, scientists say.
In space gamma rays—the most energetic forms of light—are created by violent events, such as supernovae , and powerful objects, such as neutron stars .
Scientists have known for decades that thunderstorms on Earth can also create gamma rays , possibly during lightning production. Storms that make gamma rays usually hover about 9 miles (15 kilometers) above Earth, about the same altitude at which many commercial planes fly.
The new study shows that just one of these terrestrial gamma ray flashes, or TGFs, can equal the radiation dosage of about 400 chest x-rays—creating potential hazards for frequent flyers. (Related: "Gamma Ray Burst Caused Mass Extinction?" )
In theory, stray gamma rays can alter the structure of human DNA, possibly triggering cancer .
Still, much is unknown about TGFs, including how likely it is for an airplane to fly close to gamma ray sources in storms, noted study co-author Joseph Dwyer , a space scientist at the Florida Institute of Technology.
"I would put this pretty far down on the list [of airplane hazards]," Dwyer said. "I've worked a lot on this, and I would not hesitate to hop on an airplane and fly with my kids across the country."
In fact, people are probably at bigger risk just driving their cars, said Brant Carlson, a TGF expert at Stanford University in California.
"I would say this is a very, very unlikely event, much less likely than many other flight hazards, or the hazards involved in getting to the airport in the first place," said Carlson, who was not involved in the study.
Gamma Ray Strobe Lights
Terrestrial gamma ray flashes were accidentally discovered in the 1990s, when space telescopes designed to study cosmic gamma ray sources began detecting unexpected gamma rays coming from the direction of Earth. (Related: "Mysterious 'Dragons' Make Universe's Gamma Ray Fog." )
Unlike cosmic gamma ray bursts, which typically last a few seconds, TFGs last only about one to two milliseconds.
"That's how you can recognize them immediately" as Earth-based, rather than cosmic, gamma rays, study co-author Dwyer said.
"You can think of a cosmic gamma ray burst as someone switching on a light, leaving it on for a bit, and turning it off. A terrestrial gamma ray flash is more like a strobe light—very brief and very bright." (See a picture of a gamma ray burst in space that was visible to the naked eye .)
The amount of danger TGFs pose to airplane travelers is still unknown, because scientists aren't sure exactly where TGFs originate inside a thundercloud or how big the TGF formation regions are.
"If [the source region] is big, it's more diffuse, and you don't get as many gamma rays hitting you," Dwyer said. "If it's compact, the dose is more concentrated."
Also, if TGFs originate near the tops of thunderclouds and propagate upward, airline passengers cruising below the clouds should be safe.
Few Gamma Ray Protections
If further research proves that terrestrial gamma ray flashes are a threat, there's very little airlines can do to protect passengers.
(Related: "'Smart Plane' Technology Could Help Damaged Craft Fly Right." )
"These things are so penetrating that you would need quite a bit of lead to have any chance of stopping them," Dwyer said. "And of course you can't really do that with a plane," since it would make the craft too heavy to fly.
The good news is that scientists think TGFs form inside the most violent parts of thunderstorms, areas pilots already try to avoid.
"You can probably list off a half dozen ways that a thunderstorm can kill you in an airplane," Dwyer said. "So you could just think of this as one more reason to stay away from them."
FREE BONUS ISSUE
Related topics, you may also like.
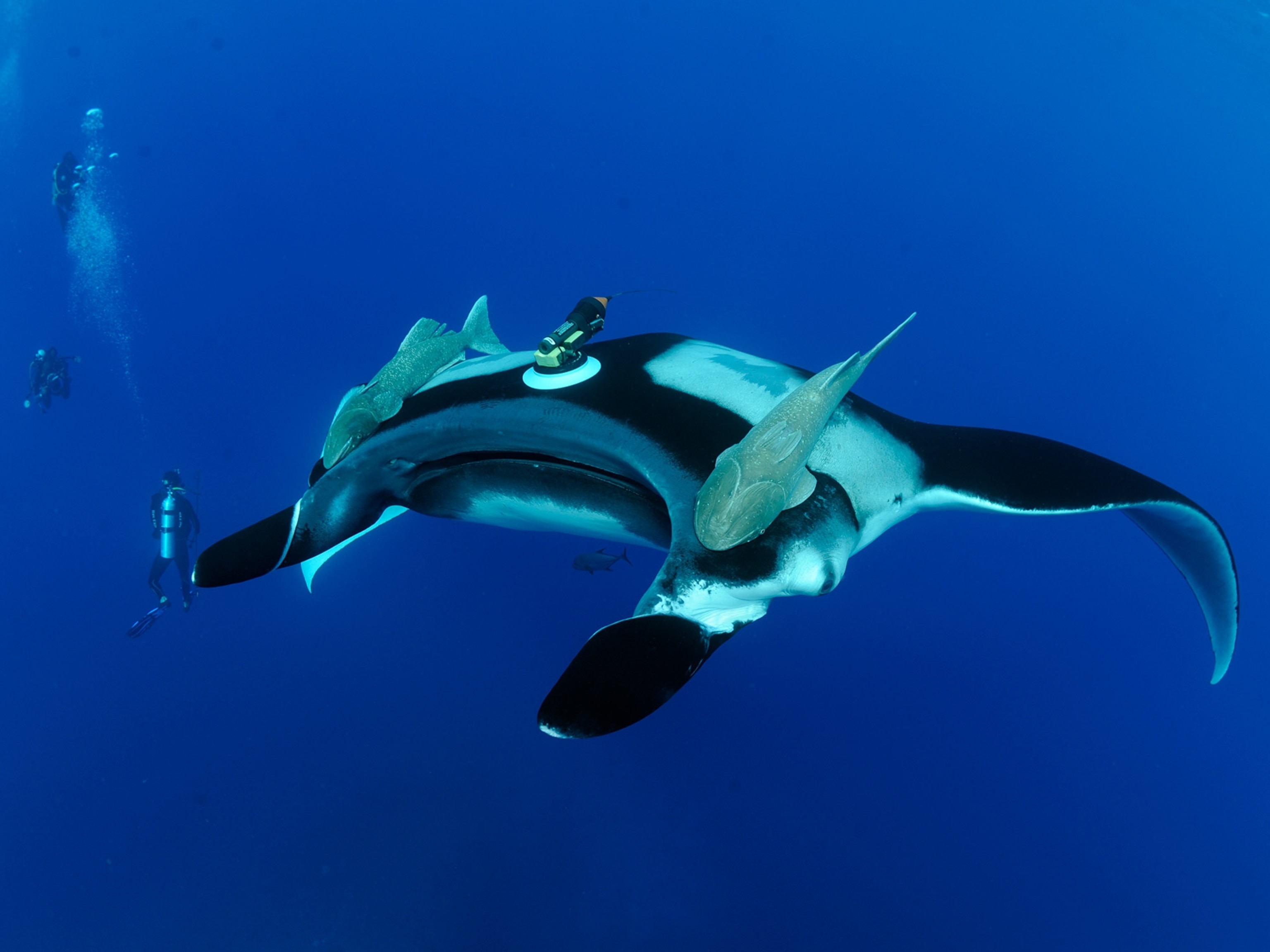
See a rare view of a manta ray courtship ritual deep in the sea
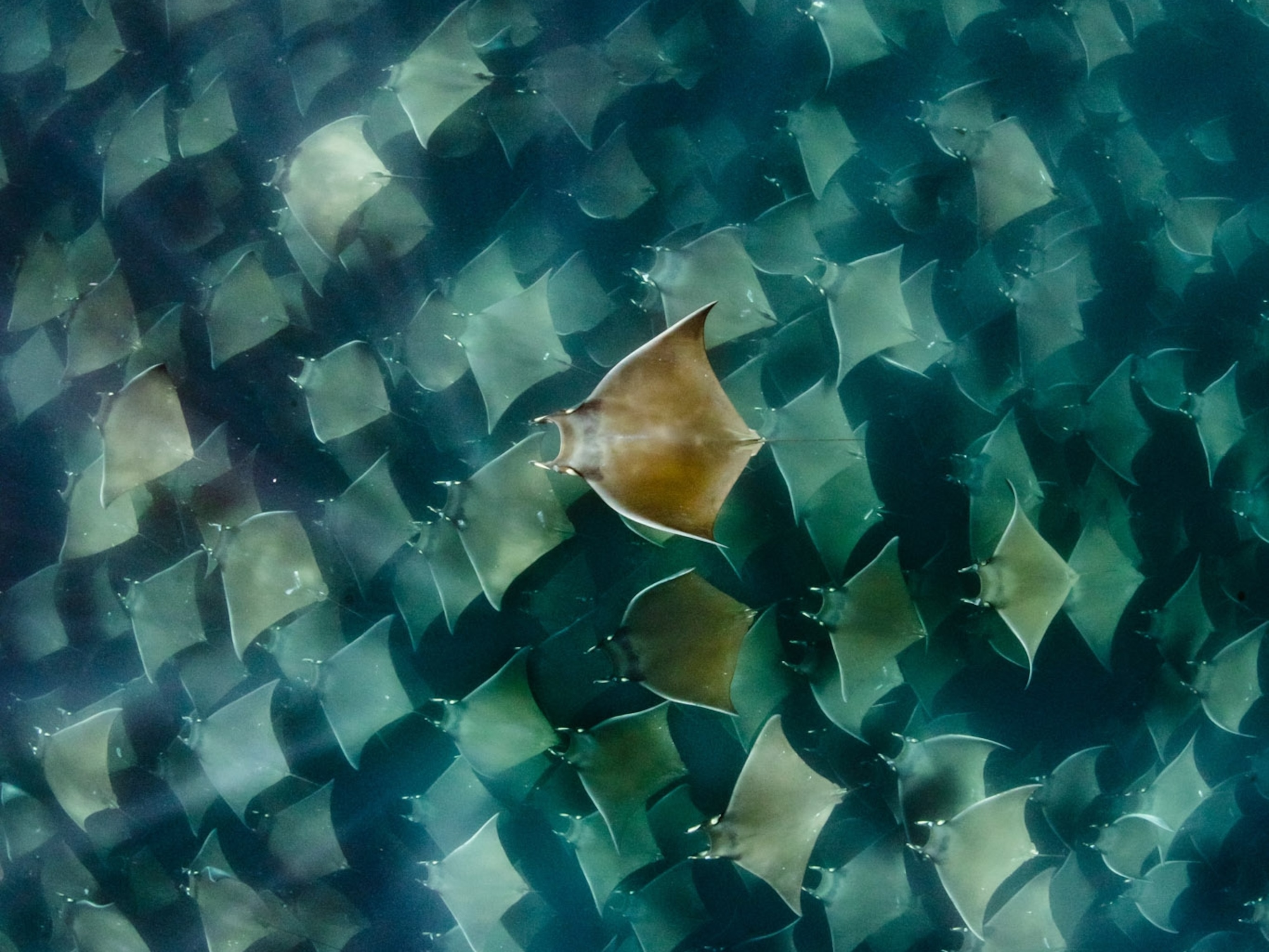
122 devil rays joined in a wild mating ritual that lasted for hours. See it here.
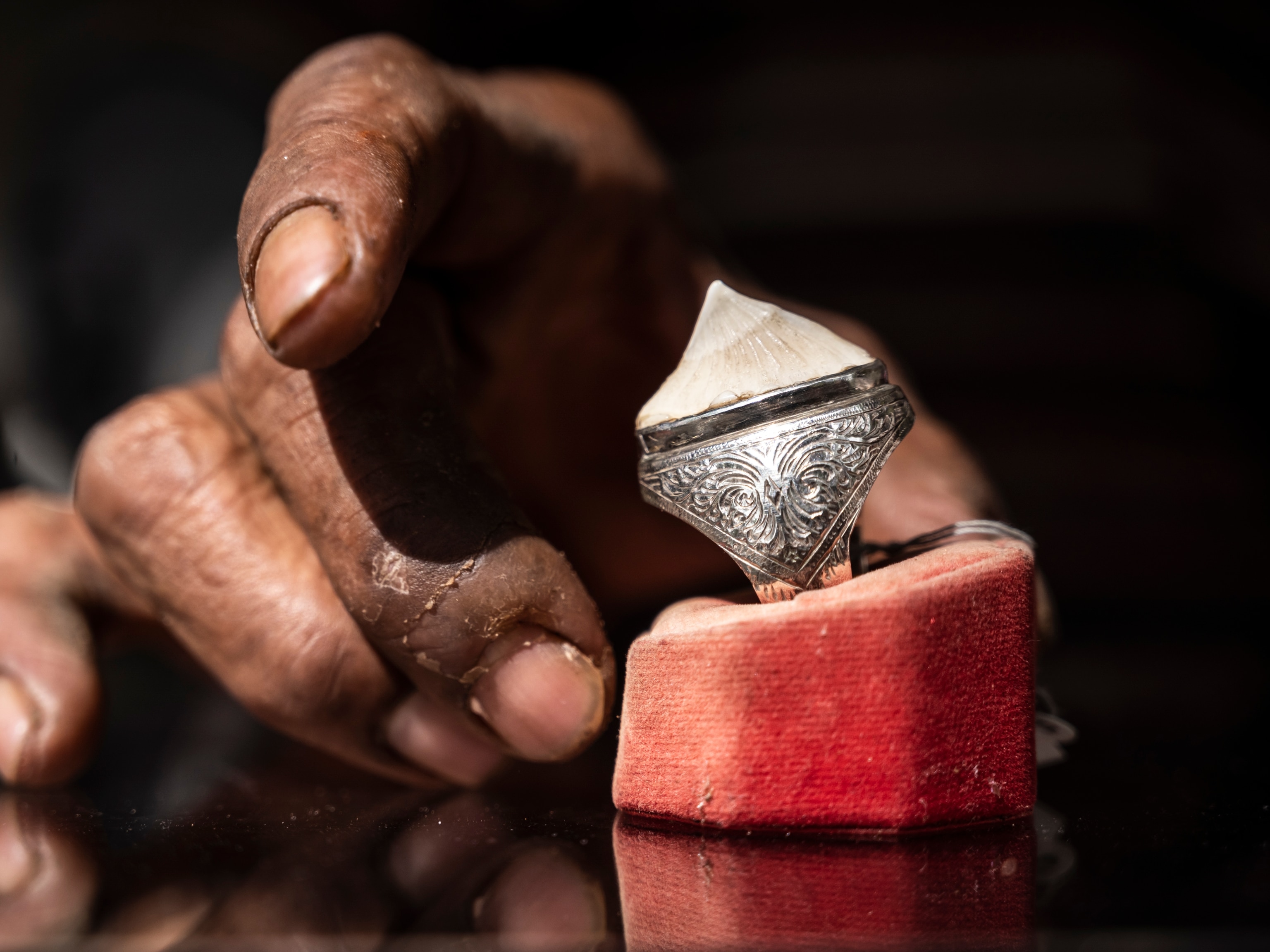
The shark ray is vanishing from our oceans—and being made into jewelry
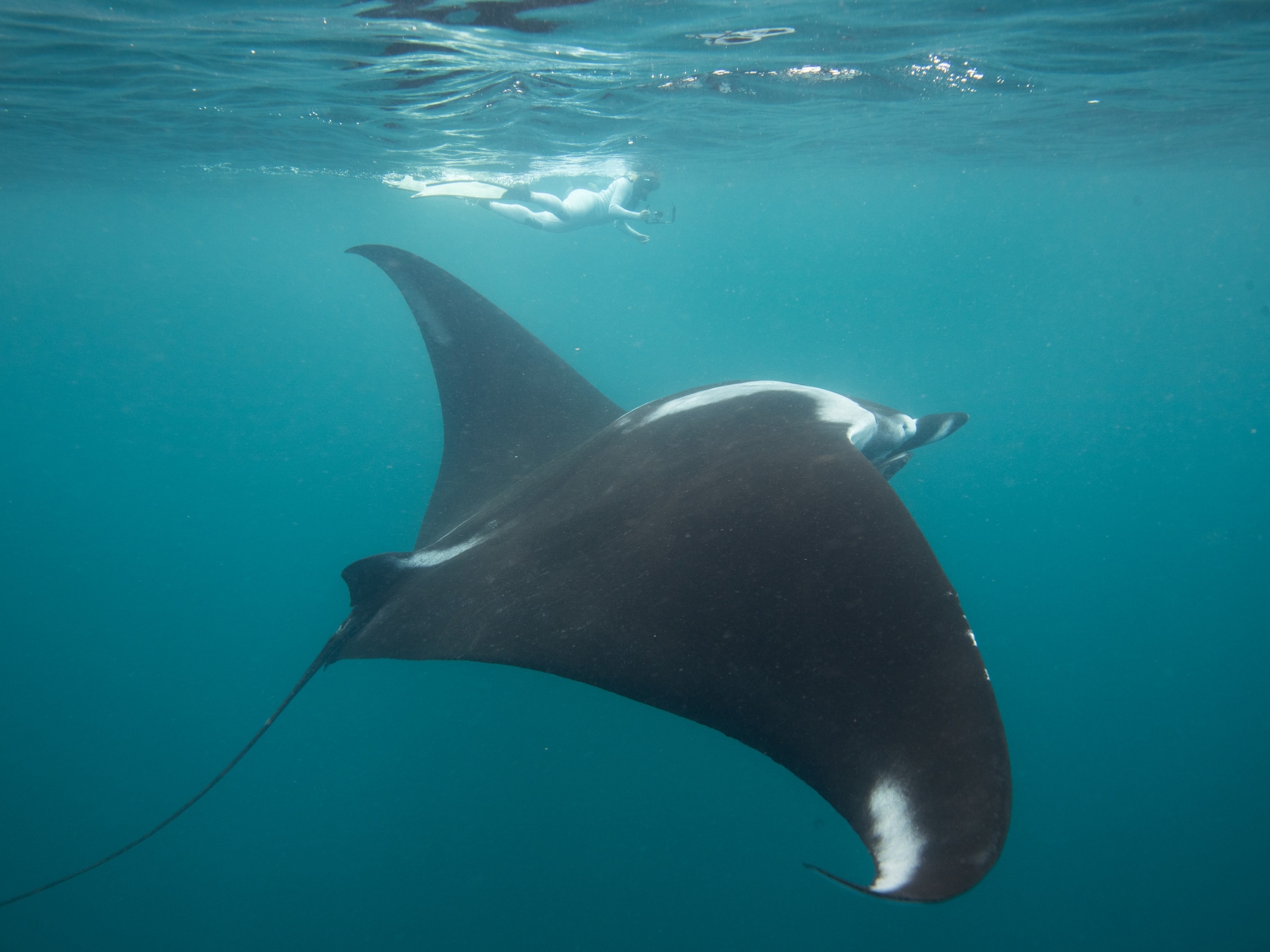
How to Give a Manta Ray a Makeover
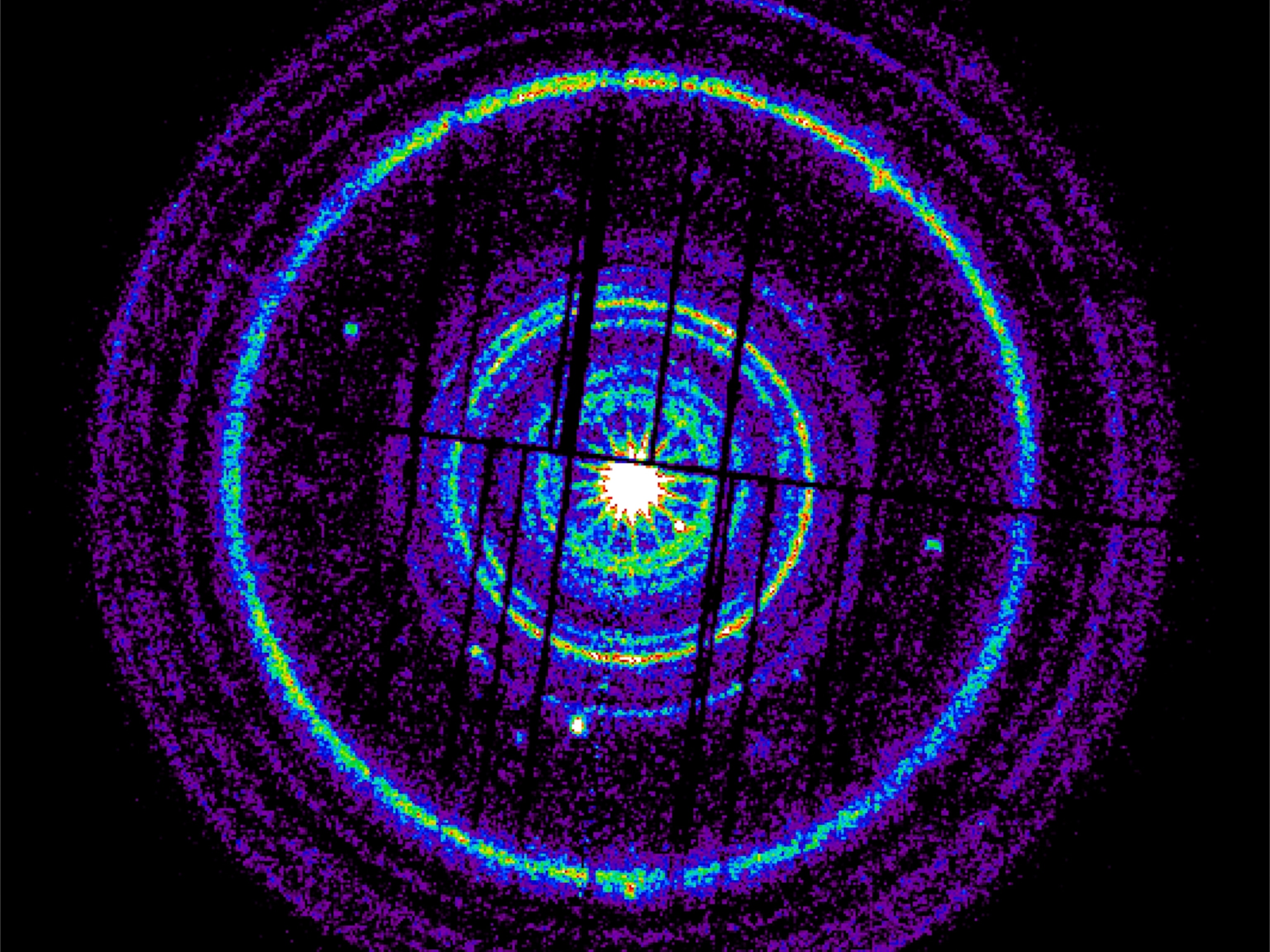
The brightest blast ever seen in space continues to surprise scientists
- Environment
- Perpetual Planet
History & Culture
- History & Culture
- History Magazine
- Mind, Body, Wonder
- Paid Content
- Terms of Use
- Privacy Policy
- Your US State Privacy Rights
- Children's Online Privacy Policy
- Interest-Based Ads
- About Nielsen Measurement
- Do Not Sell or Share My Personal Information
- Nat Geo Home
- Attend a Live Event
- Book a Trip
- Inspire Your Kids
- Shop Nat Geo
- Visit the D.C. Museum
- Learn About Our Impact
- Support Our Mission
- Advertise With Us
- Customer Service
- Renew Subscription
- Manage Your Subscription
- Work at Nat Geo
- Sign Up for Our Newsletters
- Contribute to Protect the Planet
Copyright © 1996-2015 National Geographic Society Copyright © 2015-2024 National Geographic Partners, LLC. All rights reserved
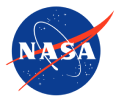
NASA’s Fermi Mission Sees No Gamma Rays from Nearby Supernova
A nearby supernova in 2023 offered astrophysicists an excellent opportunity to test ideas about how these types of explosions boost particles, called cosmic rays , to near light-speed. But surprisingly, NASA’s Fermi Gamma-ray Space Telescope detected none of the high-energy gamma-ray light those particles should produce.
On May 18, 2023, a supernova erupted in the nearby Pinwheel galaxy (Messier 101) , located about 22 million light-years away in the constellation Ursa Major. The event, named SN 2023ixf, is the most luminous nearby supernova discovered since Fermi launched in 2008.
“Astrophysicists previously estimated that supernovae convert about 10% of their total energy into cosmic ray acceleration,” said Guillem Martí-Devesa, a researcher at the University of Trieste in Italy. “But we have never observed this process directly. With the new observations of SN 2023ixf, our calculations result in an energy conversion as low as 1% within a few days after the explosion. This doesn’t rule out supernovae as cosmic ray factories, but it does mean we have more to learn about their production.”
The paper, led by Martí-Devesa while at the University of Innsbruck in Austria, will appear in a future edition of Astronomy and Astrophysics.
Trillions of trillions of cosmic rays collide with Earth’s atmosphere every day. Roughly 90% of them are hydrogen nuclei – or protons – and the remainder are electrons or the nuclei of heavier elements.
Scientists have been investigating cosmic ray origins since the early 1900s, but the particles can’t be traced back to their sources. Because they’re electrically charged, cosmic rays change course as they travel to Earth thanks to magnetic fields they encounter.
“Gamma rays, however, travel directly to us,” said Elizabeth Hays, the Fermi project scientist at NASA’s Goddard Space Flight Center in Greenbelt, Maryland. “Cosmic rays produce gamma rays when they interact with matter in their environment. Fermi is the most sensitive gamma-ray telescope in orbit, so when it doesn’t detect an expected signal, scientists must explain the absence. Solving that mystery will build a more accurate picture of cosmic ray origins.”
Astrophysicists have long suspected supernovae of being top cosmic ray contributors.
These explosions occur when a star at least eight times the Sun’s mass runs out of fuel. The core collapses and then rebounds, propelling a shock wave outward through the star. The shock wave accelerates particles, creating cosmic rays. When cosmic rays collide with other matter and light surrounding the star, they generate gamma rays.
Supernovae greatly impact a galaxy’s interstellar environment. Their blast waves and expanding cloud of debris may persist for more than 50,000 years. In 2013, Fermi measurements showed that supernova remnants in our own Milky Way galaxy were accelerating cosmic rays, which generated gamma-ray light when they struck interstellar matter. But astronomers say the remnants aren’t producing enough high-energy particles to match scientists’ measurements on Earth.
One theory proposes that supernovae may accelerate the most energetic cosmic rays in our galaxy in the first few days and weeks after the initial explosion.
But supernovae are rare, occurring only a few times a century in a galaxy like the Milky Way. Out to distances of around 32 million light-years, a supernova occurs, on average, just once a year.
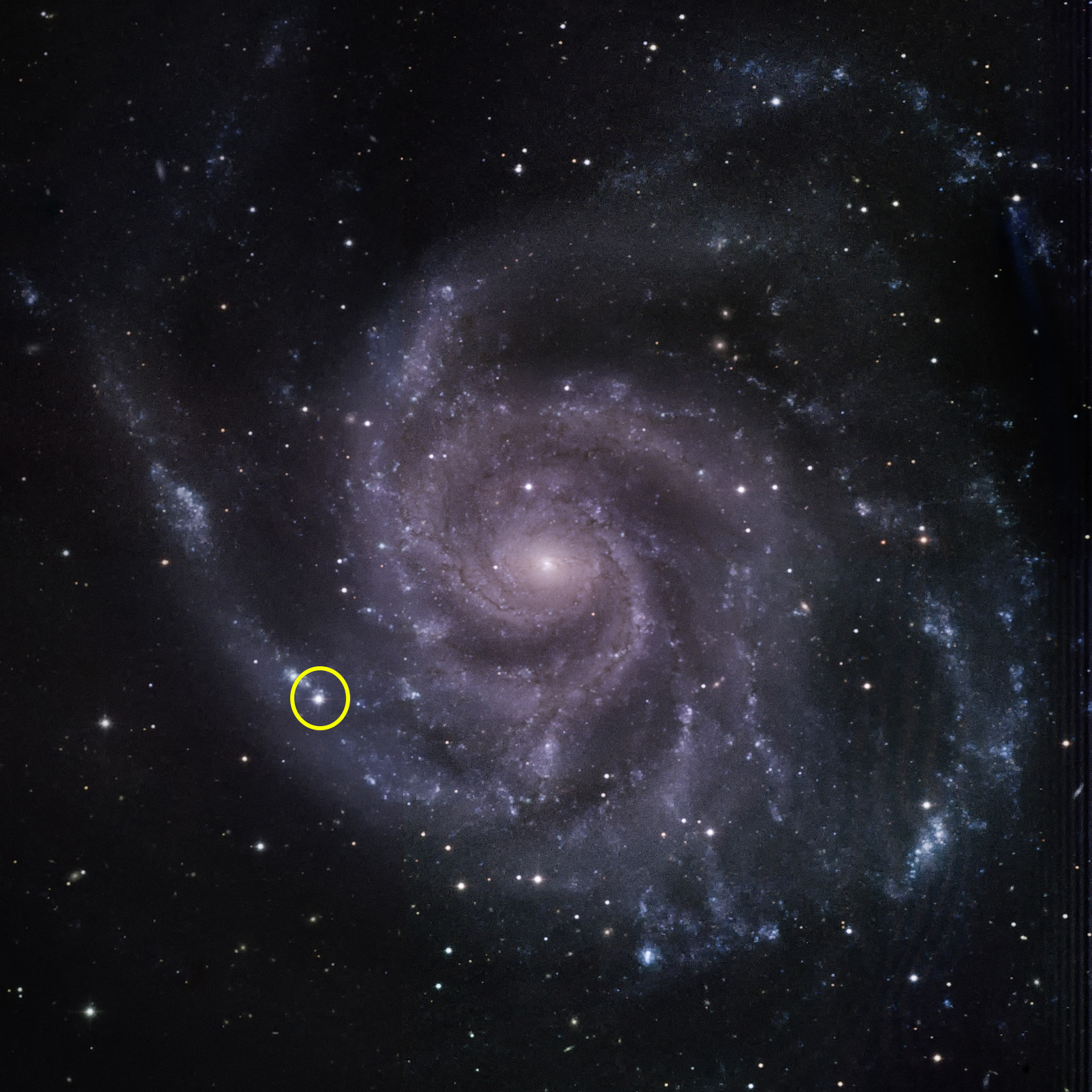
After a month of observations, starting when visible light telescopes first saw SN 2023ixf, Fermi had not detected gamma rays.
“Unfortunately, seeing no gamma rays doesn’t mean there are no cosmic rays,” said co-author Matthieu Renaud, an astrophysicist at the Montpellier Universe and Particles Laboratory , part of the National Center for Scientific Research in France. “We have to go through all the underlying hypotheses regarding acceleration mechanisms and environmental conditions in order to convert the absence of gamma rays into an upper limit for cosmic ray production.”
The researchers propose a few scenarios that may have affected Fermi’s ability to see gamma rays from the event, like the way the explosion distributed debris and the density of material surrounding the star.
Fermi’s observations provide the first opportunity to study conditions right after the supernova explosion. Additional observations of SN 2023ixf at other wavelengths, new simulations and models based on this event, and future studies of other young supernovae will help astronomers home in on the mysterious sources of the universe’s cosmic rays.
Fermi is an astrophysics and particle physics partnership managed by Goddard. Fermi was developed in collaboration with the U.S. Department of Energy, with important contributions from academic institutions and partners in France, Germany, Italy, Japan, Sweden, and the United States.
By Jeanette Kazmierczak NASA’s Goddard Space Flight Center , Greenbelt, Md.
Media contact: Claire Andreoli 301-286-1940 [email protected] NASA’s Goddard Space Flight Center , Greenbelt, Md.
Related Terms
- Astrophysics
- Fermi Gamma-Ray Space Telescope
- Goddard Space Flight Center
- The Universe
Explore More
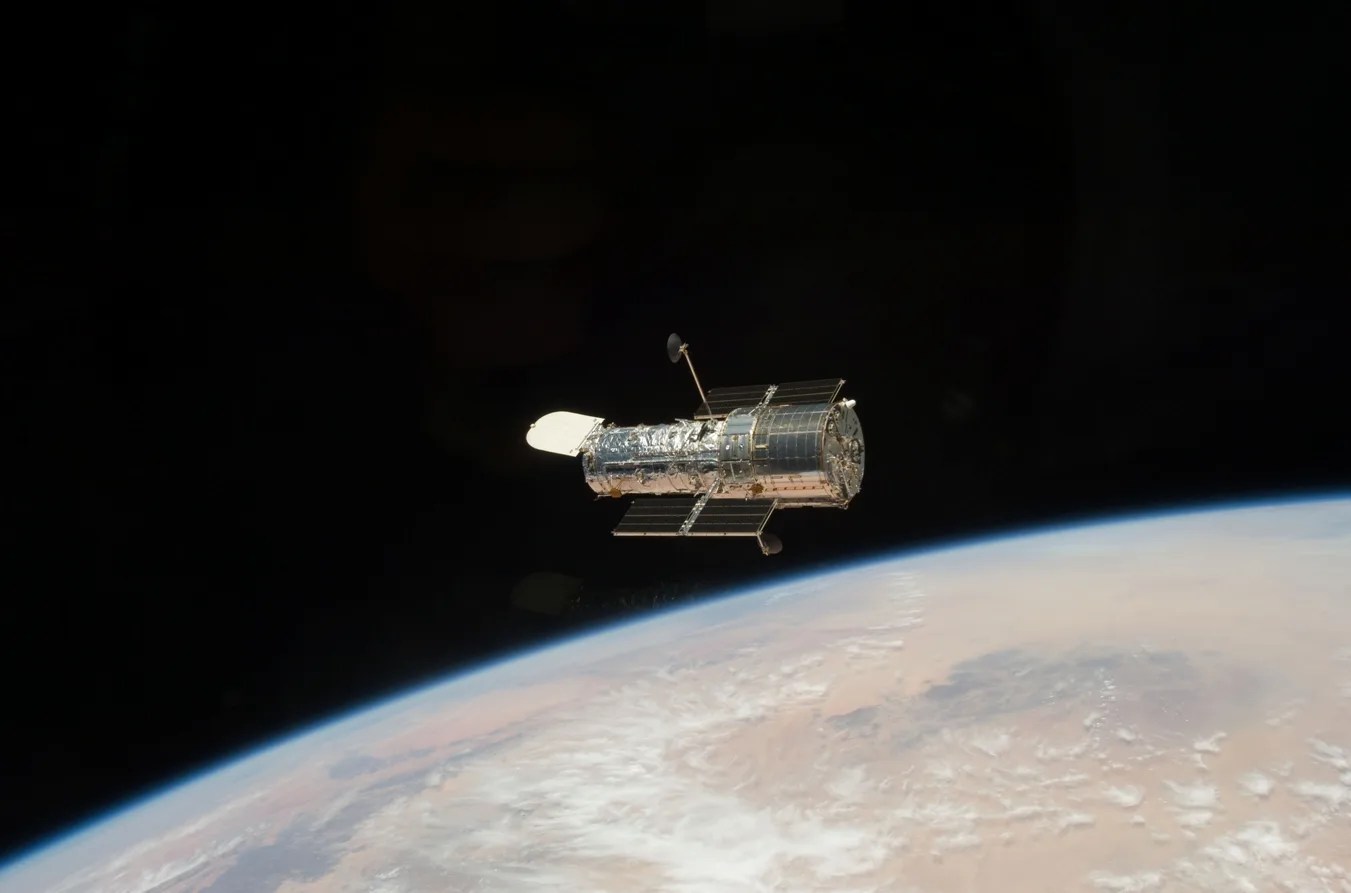
NASA’s Hubble Pauses Science Due to Gyro Issue
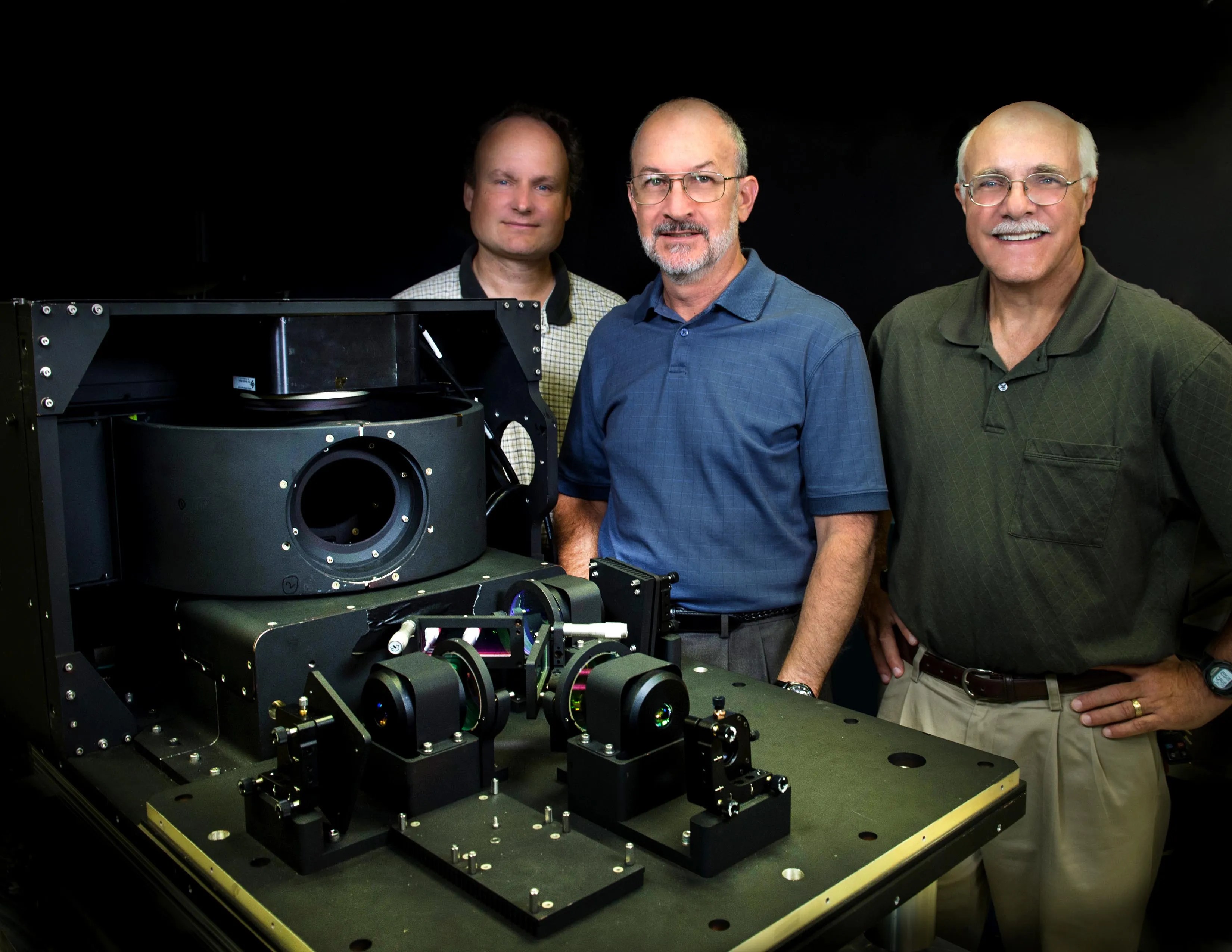
NASA’s ORCA, AirHARP Projects Paved Way for PACE to Reach Space
It took the Plankton, Aerosol, Cloud, ocean Ecosystem (PACE) mission just 13 minutes to reach low-Earth orbit from Cape Canaveral Space Force Station in February 2024. It took a network of scientists at NASA and research institutions around the world more than 20 years to carefully craft and test the novel instruments that allow PACE […]
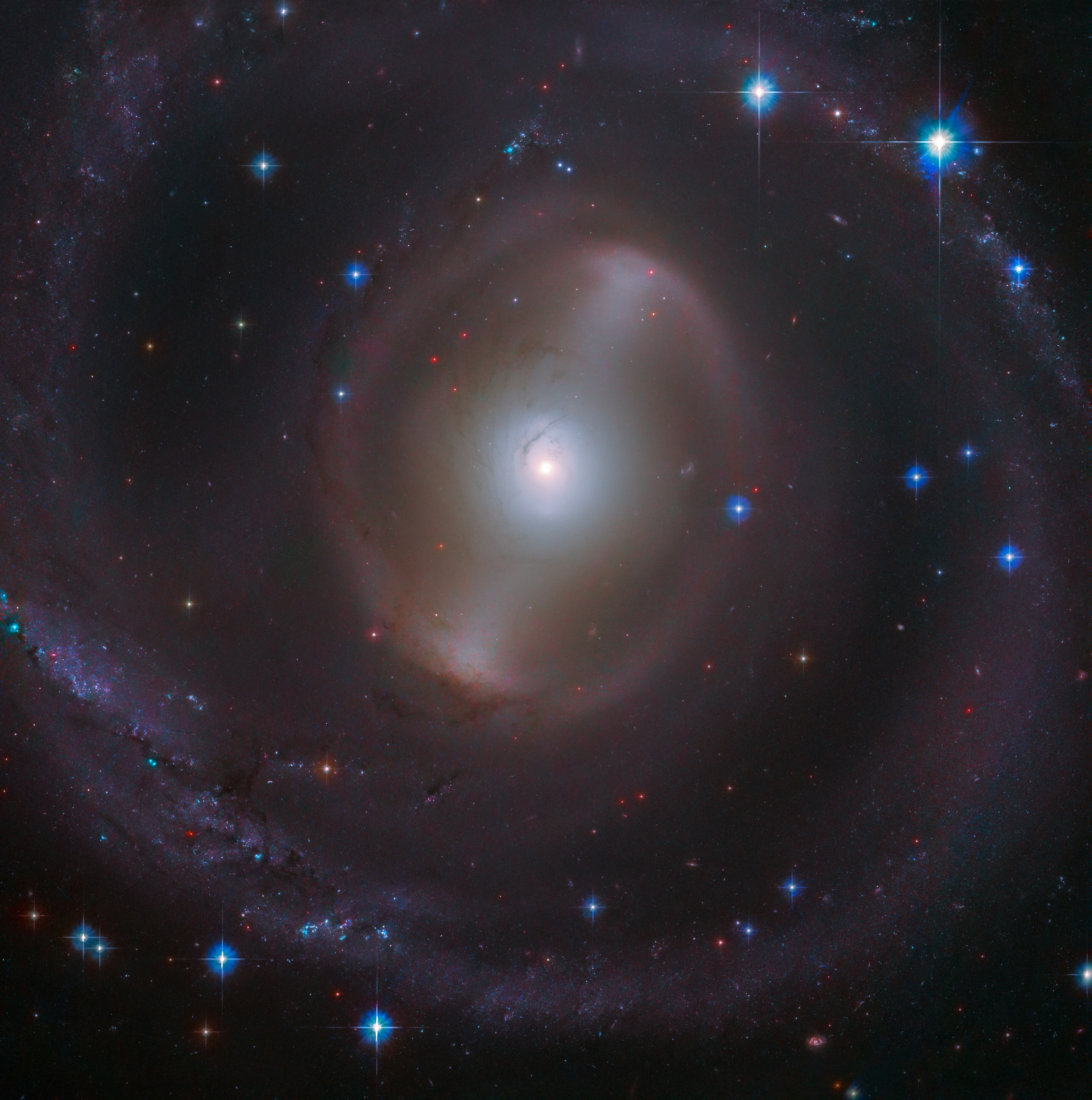
Hubble Spots a Magnificent Barred Galaxy
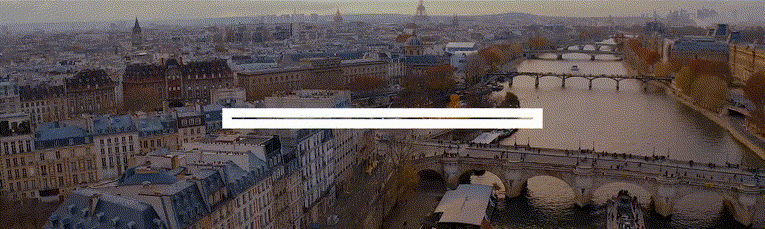
IMAGES
VIDEO
COMMENTS
Gamma rays can pass completely through the human body; as they pass through, ... they are so heavy that they use up their energy over short distances and are unable to travel very far from the atom. ... They travel farther in air than alpha particles, but can be stopped by a layer of clothing or by a thin layer of a substance such as aluminum. ...
A nucleus will regain stability by emitting alpha or beta particles and then 'cool down' by emitting gamma radiation. ... how far into a material the ... and how far they can travel in the air
gamma radiation is high energy electromagnetic waves emitted from unstable nuclei; gamma radiation easily passes through air, paper, skin and aluminium but can be partly blocked by thick lead or ...
Nonetheless, a thin sheet of metal or plastic or a block of wood can stop beta particles. Gamma Rays and X-Rays. Gamma rays and x-rays consist of high-energy waves that can travel great distances at the speed of light and generally have a great ability to penetrate other materials. For that reason, gamma rays (such as from cobalt-60) are often ...
Travel distance depends on the type of radiation, as does the ability to penetrate other materials. Alpha and beta particles do not travel far at all, and they are easily blocked. By contrast, gamma rays, x-rays, and neutrons travel a
Gamma rays have wavelengths shorter than 10^-11 meters and frequencies above 30 x 10^18 hertz. The European Space Agency describes how gamma-ray photons have energies in excess of 100,000 ...
A gamma ray, also known as gamma radiation (symbol γ), is a penetrating form of electromagnetic radiation arising from the radioactive decay of atomic nuclei.It consists of the shortest wavelength electromagnetic waves, typically shorter than those of X-rays.With frequencies above 30 exahertz (3 × 10 19 Hz) and wavelength less than 10 picometer (1 × 10 −11 m) gamma ray photons have the ...
Gamma rays can travel many yards in air. Gamma rays are primarily an external hazard because of their ability to go through material. It takes a few inches of lead or other dense substance to block gamma rays. Gamma rays also can be an internal hazard if we breathe or eat gamma-emitting radioactive materials, or if the radioactive material is ...
Gamma rays can also travel very far and can deposit or travel through substances such as iron, tissue, and even concrete. X-ray production occurs in a similar pattern, but instead of creating energy in the nucleus, energy generation occurs in the surrounding electrons.
A nucleus will regain stability by emitting alpha or beta particles and then 'cool down' by emitting gamma radiation. ... demonstrates how far into ... power and how far they can travel in the air
Being electrically neutral, the interaction of gamma rays with matter is a statistical process and depends on the nature of the absorber as well as the energy of the gamma. ... air (STP) 1.2 mg/cm 3: 3.7 cm - paper (20lb) 0.89 g/cm 3: 53 µm one sheet = 89 µm water (soft tissue) 1.0 g/cm 3: 45 µm ...
2. In a beam of 22 MeV gamma rays passing through dry air at sea level, half will be absorbed in about 350 meters. Photons at lower energies are absorbed in a shorter distance (for 2 MeV, it's about 130 meters).
Gamma radiation is able to travel many meters in air and many centimeters in human tissue. It readily penetrates most materials. X-rays are like gamma rays. They can also travel over long distances in both air and human tissue. Radioactive materials that emit gamma radiation and X-rays constitute both an external and internal hazard to humans.
Gamma radiation is very penetrating and can travel several hundred feet in air. Gamma radiation requires a thick wall of concrete, lead, or steel to stop it. Neutrons-A neutron is an atomic particle that has about one-quarter the weight of an alpha particle. Like gamma radiation, it can easily travel several hundred feet in air.
Gamma-ray bursts are the most energetic and luminous electromagnetic events since the Big Bang and can release more energy in 10 seconds than our Sun will emit in its entire 10-billion-year expected lifetime! Gamma-ray astronomy presents unique opportunities to explore these exotic objects. By exploring the universe at these high energies ...
For example, a gamma-ray photon has one million to one trillion (one million million) times the energy of a photon of visible light. And, unlike photons of radio and visible light, gamma-ray photons cannot penetrate the earth's atmosphere, for they are absorbed by interactions with air molecules ten miles above the Earth's surface.
A beta particle is an electron ejected from a nucleus when a neutron becomes a proton. travels tens of centimetres in air, and gamma radiation travels very large distances.
This bolstered the model of short bursts as mergers of neutron stars, which can travel far from their birth place over the many millions of years it takes for them to crash together. In 2008, NASA's Fermi Gamma-ray Space Telescope joined Swift in hunting GRBs and has observed about 3,500 to date.
For many years, analysis of air samples has shown risk levels far below regulatory limits. In fact, results are now generally below levels that instruments can detect. ... Gamma rays, however, travel much farther in the atmosphere, and are higher energy rays that can only be blocked by heavy shielding, like a concrete wall or a lead apron. ...
Answer and Explanation: 1. Become a Study.com member to unlock this answer! Create your account. View this answer. The distance gamma rays can travel in air depends on the extent of their energy; it can range from about 900 meters to kilometers. In air if one... See full answer below.
Storms that make gamma rays usually hover about 9 miles (15 kilometers) above Earth, about the same altitude at which many commercial planes fly. The new study shows that just one of these ...
Substances in its path can absorb radiation. For example, alpha radiation travels only a few centimetres in air, beta radiation travels tens of centimetres in air, and gamma radiation travels very ...
A nearby supernova in 2023 offered astrophysicists an excellent opportunity to test ideas about how these types of explosions boost particles, called cosmic rays, to near light-speed. But surprisingly, NASA's Fermi Gamma-ray Space Telescope detected none of the high-energy gamma-ray light those particles should produce. On May 18, 2023, a supernova erupted in the nearby […]
Learn about and revise irradiation, contamination and the uses and dangers or radiation with GCSE Bitesize Physics.