- Random article
- Teaching guide
- Privacy & cookies
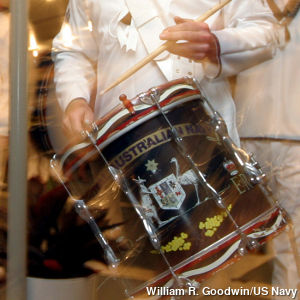
by Chris Woodford . Last updated: July 23, 2023.
Photo: Sound is energy we hear made by things that vibrate. Photo by William R. Goodwin courtesy of US Navy and Wikimedia Commons .
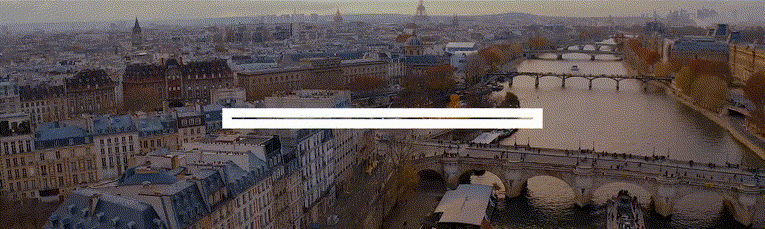
What is sound?
Photo: Sensing with sound: Light doesn't travel well through ocean water: over half the light falling on the sea surface is absorbed within the first meter of water; 100m down and only 1 percent of the surface light remains. That's largely why mighty creatures of the deep rely on sound for communication and navigation. Whales, famously, "talk" to one another across entire ocean basins, while dolphins use sound, like bats, for echolocation. Photo by Bill Thompson courtesy of US Fish and Wildlife Service .
Robert Boyle's classic experiment
Artwork: Robert Boyle's famous experiment with an alarm clock.
How sound travels
Artwork: Sound waves and ocean waves compared. Top: Sound waves are longitudinal waves: the air moves back and forth along the same line as the wave travels, making alternate patterns of compressions and rarefactions. Bottom: Ocean waves are transverse waves: the water moves back and forth at right angles to the line in which the wave travels.
The science of sound waves
Picture: Reflected sound is extremely useful for "seeing" underwater where light doesn't really travel—that's the basic idea behind sonar. Here's a side-scan sonar (reflected sound) image of a World War II boat wrecked on the seabed. Photo courtesy of U.S. National Oceanographic and Atmospheric Administration, US Navy, and Wikimedia Commons .
Whispering galleries and amphitheaters
Photos by Carol M. Highsmith: 1) The Capitol in Washington, DC has a whispering gallery inside its dome. Photo credit: The George F. Landegger Collection of District of Columbia Photographs in Carol M. Highsmith's America, Library of Congress , Prints and Photographs Division. 2) It's easy to hear people talking in the curved memorial amphitheater building at Arlington National Cemetery, Arlington, Virginia. Photo credit: Photographs in the Carol M. Highsmith Archive, Library of Congress , Prints and Photographs Division.
Measuring waves
Understanding amplitude and frequency, why instruments sound different, the speed of sound.
Photo: Breaking through the sound barrier creates a sonic boom. The mist you can see, which is called a condensation cloud, isn't necessarily caused by an aircraft flying supersonic: it can occur at lower speeds too. It happens because moist air condenses due to the shock waves created by the plane. You might expect the plane to compress the air as it slices through. But the shock waves it generates alternately expand and contract the air, producing both compressions and rarefactions. The rarefactions cause very low pressure and it's these that make moisture in the air condense, producing the cloud you see here. Photo by John Gay courtesy of US Navy and Wikimedia Commons .
Why does sound go faster in some things than in others?
Chart: Generally, sound travels faster in solids (right) than in liquids (middle) or gases (left)... but there are exceptions!
How to measure the speed of sound
Sound in practice, if you liked this article..., find out more, on this website.
- Electric guitars
- Speech synthesis
- Synthesizers
On other sites
- Explore Sound : A comprehensive educational site from the Acoustical Society of America, with activities for students of all ages.
- Sound Waves : A great collection of interactive science lessons from the University of Salford, which explains what sound waves are and the different ways in which they behave.
Educational books for younger readers
- Sound (Science in a Flash) by Georgia Amson-Bradshaw. Franklin Watts/Hachette, 2020. Simple facts, experiments, and quizzes fill this book; the visually exciting design will appeal to reluctant readers. Also for ages 7–9.
- Sound by Angela Royston. Raintree, 2017. A basic introduction to sound and musical sounds, including simple activities. Ages 7–9.
- Experimenting with Sound Science Projects by Robert Gardner. Enslow Publishers, 2013. A comprehensive 120-page introduction, running through the science of sound in some detail, with plenty of hands-on projects and activities (including welcome coverage of how to run controlled experiments using the scientific method). Ages 9–12.
- Cool Science: Experiments with Sound and Hearing by Chris Woodford. Gareth Stevens Inc, 2010. One of my own books, this is a short introduction to sound through practical activities, for ages 9–12.
- Adventures in Sound with Max Axiom, Super Scientist by Emily Sohn. Capstone, 2007. The original, graphic novel (comic book) format should appeal to reluctant readers. Ages 8–10.
Popular science
- The Sound Book: The Science of the Sonic Wonders of the World by Trevor Cox. W. W. Norton, 2014. An entertaining tour through everyday sound science.
Academic books
- Master Handbook of Acoustics by F. Alton Everest and Ken Pohlmann. McGraw-Hill Education, 2015. A comprehensive reference for undergraduates and sound-design professionals.
- The Science of Sound by Thomas D. Rossing, Paul A. Wheeler, and F. Richard Moore. Pearson, 2013. One of the most popular general undergraduate texts.
Text copyright © Chris Woodford 2009, 2021. All rights reserved. Full copyright notice and terms of use .
Rate this page
Tell your friends, cite this page, more to explore on our website....
- Get the book
- Send feedback
Want to create or adapt books like this? Learn more about how Pressbooks supports open publishing practices.
Traveling waves
17 How sound moves
Speed of sound.
There’s a delay between when a sound is created and when it is heard. In everyday life, the delay is usually too short to notice. However, the delay can be noticeable if the distance between source and detector is large enough. You see lightning before you hear the thunder. If you’ve sat in the outfield seats in a baseball stadium, you’ve experienced the delay between seeing the player hit the ball and the sound of the “whack.” Life experiences tell us that sound travels fast, but not nearly as fast as light does. Careful experiments confirm this idea.
The speed of sound in air is roughly 340 m/s. The actual value depends somewhat on the temperature and humidity. In everyday terms, sound travels about the length of three and a half foot ball fields every second- about 50% faster than a Boeing 747 (roughly 250 m/s). This may seem fast, but it’s tiny compared to light, which travels roughly a million times faster than sound (roughly 300,000,000 m/s).
Sound requires some material in which to propagate (i.e. travel). This material sound travels through is called the medium . You can show that sound requires a medium by putting a cell phone inside a glass jar connected to a vacuum pump. As the air is removed from the jar, the cell phone’s ringer gets quieter and quieter. A youTube video (2:05 min) produced by the UNSW PhysClips project shows the demo with a drumming toy monkey [1] instead of a cell phone.
What affects the speed of sound?
Sound travels at different speeds though different materials. The physical properties of the medium are the only factors that affect the speed of sound- nothing else matters.
The speed of sound in a material is determined mainly by two properties- the stiffness of the material and the density of the material. Sound travels fastest through materials that are stiff and light. In general, sound travels fastest through solids, slower through liquids and slowest through gasses. (See the table on this page). This may seem backwards- after all, metals are quite dense. However, the high density of metals is more than offset by far greater stiffness (compared to liquids and solids).
The speed of sound in air depends mainly on temperature. The speed of sound is 331 m/s in dry air at 0 o Celsius and increases slightly with temperature- about 0.6 m/s for every 1 o Celsius for temperatures commonly found on Earth. Though speed of sound in air also depends on humidity, the effect is tiny- sound travels only about 1 m/s faster in air with 100% humidity air at 20 o C than it does in completely dry air at the same temperature.
Nothing else matters
The properties of the medium are the only factors that affect the speed of sound- nothing else matters.
Frequency of the sound does not matter- high frequency sounds travel at the same speed as low frequency sounds. If you’ve ever listened to music, you’ve witnessed this- the low notes and the high notes that were made simultaneously reach you simultaneously, even if you are far from the stage. If you’ve heard someone shout from across a field, you’ve noticed that the entire shout sound (which contains many different frequencies at once) reaches you at the same time. If different frequencies traveled at different rates, some frequencies would arrive before others.
The amplitude of the sound does not matter- loud sounds and quiet ones travel at the same speed. Whisper or yell- it doesn’t matter. The sound still takes the same amount of time to reach the listener. You’ve probably heard that you can figure out how far away the lightning by counting the seconds between when you see lightning and hear thunder. If the speed of sound depended on loudness, this rule of thumb would have to account for loudness- yet there is nothing in the rule about loud vs. quiet thunder. The rule of thumb works the same for all thunder- regardless of loudness . That’s because the speed of sound doesn’t depend on amplitude.
Stop to thinks
- Which takes longer to cross a football field: the sound of a high pitched chirp emitted by a fruit bat or the (relatively) low pitched sound emitted by a trumpet?
- Which sound takes longer to travel 100 meters: the sound of a snapping twig in the forest or the sound of a gunshot?
- Which takes longer to travel the distance of a football field: the low pitched sound of a whale or the somewhat higher pitched sound of a human being?
Constant speed
Sound travels at a constant speed. Sound does not speed up or slow down as it travels (unless the properties of the material the sound is going through changes). I know what you’re thinking- how is that possible? Sounds die out as they travel, right? True. That means sounds must slow down and come to a stop, right? Wrong. As sound travels, its amplitude decreases- but that’s not the same thing as slowing down. Sound (in air) covers roughly 340 meters each and every second, even as its amplitude shrinks. Eventually, the amplitude gets small enough that the sound is undetectable. A sound’s amplitude shrinks as it travels, but its speed remains constant.
The basic equation for constant speed motion (shown below) applies to sound.
[latex]d=vt[/latex]
In this equation, [latex]d[/latex] represents the distance traveled by the sound, [latex]t[/latex] represents the amount of time it took to go that distance and [latex]v[/latex] represents the speed.
Rule of thumb for lightning example
Example: thunder and lightning.
The rule of thumb for figuring out how far away a lightning strike is from you is this:
Count the number of seconds between when you see the lightning and hear the thunder. Divide the number of seconds by five to find out how many miles away the lightning hit.
According to this rule, what is the speed of sound in air? How does the speed of sound implied by this rule compare to 340 m/s?
Identify important physics concept : This question is about speed of sound.
List known and unknown quantities (with letter names and units):
At first glance, it doesn’t look like there’s enough information to solve the problem. We were asked to find speed, but not given either a time or a distance. However, the problem does allow us to figure out a distance if we know the time- “Divide the number of seconds by five to find out how many miles away the lightning hit.” So, let’s make up a time and see what happens; if the time is 10 seconds, the rule of thumb says that the distance should be 2 miles.
[latex]v= \: ?[/latex]
[latex]d=2 \: miles[/latex]
[latex]t=10 \: seconds[/latex]
You might ask “Is making stuff up OK here?” The answer is YES! If the rule of thumb is right, it should work no matter what time we choose. (To check if the rule is OK, we should probably test it with more than just one distance-time combination, but we’ll assume the rule is OK for now).
Do the algebra: The equation is already solved for speed. Move on to the next step.
Do unit conversions (if needed) then plug in numbers: If you just plug in the numbers, the speed comes out in miles per second:
[latex]v = \frac{2 \: miles} {10 \: seconds}=0.2 \: \frac{miles} {second}[/latex]
We are asked to compare this speed to 340 m/s, so a unit conversion is in order; since there are 1609 meters in a mile:
[latex]v =0.2 \: \frac{miles} {second}*\frac{1609 \: meters} {1 \:mile}=320 \frac{m}{s}[/latex]
Reflect on the answer:
- The answer for speed from the rule of thumb is less than 10% off the actual value of roughly 340 m/s- surprisingly close!
- At the beginning, we assumed a time of 10 seconds. Does the result hold up for other choices? A quick check shows that it does! For instance, if we use a time of 5 seconds, the rule of thumb gives a distance of 1 mile, and the math still gives a speed of 0.2 miles/second. The speed will be the same no matter what time we pick. The reason is this: The more time it takes the thunder to arrive, the farther away the lightning strike is, but the speed remains the same. In the equation for speed, both time and distance change by the same factor and the overall fraction remains unchanged.
Stop to think answers
- Both sounds take the same amount of time. (High and low pitched sounds travel at the same speed).
- Both sounds take the same amount of time. (Quiet sounds and loud sounds travel at the same speed).
- The sound of the whale travels the distance in less time- assuming sound from the whale travels in water and sound from the human travels in air. Sound travels faster in water than in air. (The info about frequency was given just to throw you off- frequency doesn’t matter).
- Wolfe, J. (2014, February 20). Properties of Sound. Retrieved from https://www.youtube.com/watch?v=P8-govgAffY ↵
Understanding Sound Copyright © by dsa2gamba and abbottds is licensed under a Creative Commons Attribution 4.0 International License , except where otherwise noted.
Share This Book
If you're seeing this message, it means we're having trouble loading external resources on our website.
If you're behind a web filter, please make sure that the domains *.kastatic.org and *.kasandbox.org are unblocked.
To log in and use all the features of Khan Academy, please enable JavaScript in your browser.
Physics library
Course: physics library > unit 8.
- Production of sound
- Sound Properties: Amplitude, period, frequency, wavelength
Speed of Sound
- Relative speed of sound in solids, liquids, and gases
- Mach numbers
- Decibel Scale
- Why do sounds get softer?
- Ultrasound medical imaging
Want to join the conversation?
- Upvote Button navigates to signup page
- Downvote Button navigates to signup page
- Flag Button navigates to signup page

Video transcript
17.2 Speed of Sound, Frequency, and Wavelength
Sound, like all waves, travels at a certain speed and has the properties of frequency and wavelength. You can observe direct evidence of the speed of sound while watching a fireworks display. The flash of an explosion is seen well before its sound is heard, implying both that sound travels at a finite speed and that it is much slower than light. You can also directly sense the frequency of a sound. Perception of frequency is called pitch . The wavelength of sound is not directly sensed, but indirect evidence is found in the correlation of the size of musical instruments with their pitch. Small instruments, such as a piccolo, typically make high-pitch sounds, while large instruments, such as a tuba, typically make low-pitch sounds. High pitch means small wavelength, and the size of a musical instrument is directly related to the wavelengths of sound it produces. So a small instrument creates short-wavelength sounds. Similar arguments hold that a large instrument creates long-wavelength sounds.
The relationship of the speed of sound, its frequency, and wavelength is the same as for all waves:
where v w v w size 12{v size 8{w}} {} is the speed of sound, f f size 12{f} {} is its frequency, and λ λ size 12{λ} {} is its wavelength. The wavelength of a sound is the distance between adjacent identical parts of a wave—for example, between adjacent compressions as illustrated in Figure 17.8 . The frequency is the same as that of the source and is the number of waves that pass a point per unit time.
Table 17.1 makes it apparent that the speed of sound varies greatly in different media. The speed of sound in a medium is determined by a combination of the medium’s rigidity (or compressibility in gases) and its density. The more rigid (or less compressible) the medium, the faster the speed of sound. For materials that have similar rigidities, sound will travel faster through the one with the lower density because the sound energy is more easily transferred from particle to particle. The speed of sound in air is low, because air is compressible. Because liquids and solids are relatively rigid and very difficult to compress, the speed of sound in such media is generally greater than in gases.
Earthquakes, essentially sound waves in Earth’s crust, are an interesting example of how the speed of sound depends on the rigidity of the medium. Earthquakes have both longitudinal and transverse components, and these travel at different speeds. The bulk modulus of granite is greater than its shear modulus. For that reason, the speed of longitudinal or pressure waves (P-waves) in earthquakes in granite is significantly higher than the speed of transverse or shear waves (S-waves). Both components of earthquakes travel slower in less rigid material, such as sediments. P-waves have speeds of 4 to 7 km/s, and S-waves correspondingly range in speed from 2 to 5 km/s, both being faster in more rigid material. The P-wave gets progressively farther ahead of the S-wave as they travel through Earth’s crust. The time between the P- and S-waves is routinely used to determine the distance to their source, the epicenter of the earthquake.
The speed of sound is affected by temperature in a given medium. For air at sea level, the speed of sound is given by
where the temperature (denoted as T T ) is in units of kelvin. The speed of sound in gases is related to the average speed of particles in the gas, v rms v rms size 12{v size 8{ ital "rms"}} {} , and that
where k k is the Boltzmann constant ( 1.38 × 10 −23 J/K 1.38 × 10 −23 J/K ) and m m is the mass of each (identical) particle in the gas. So, it is reasonable that the speed of sound in air and other gases should depend on the square root of temperature. While not negligible, this is not a strong dependence. At 0ºC 0ºC , the speed of sound is 331 m/s, whereas at 20.0ºC 20.0ºC it is 343 m/s, less than a 4% increase. Figure 17.9 shows a use of the speed of sound by a bat to sense distances. Echoes are also used in medical imaging.
One of the more important properties of sound is that its speed is nearly independent of frequency. This independence is certainly true in open air for sounds in the audible range of 20 to 20,000 Hz. If this independence were not true, you would certainly notice it for music played by a marching band in a football stadium, for example. Suppose that high-frequency sounds traveled faster—then the farther you were from the band, the more the sound from the low-pitch instruments would lag that from the high-pitch ones. But the music from all instruments arrives in cadence independent of distance, and so all frequencies must travel at nearly the same speed. Recall that
In a given medium under fixed conditions, v w v w is constant, so that there is a relationship between f f size 12{f} {} and λ λ size 12{λ} {} ; the higher the frequency, the smaller the wavelength. See Figure 17.10 and consider the following example.
Example 17.1
Calculating wavelengths: what are the wavelengths of audible sounds.
Calculate the wavelengths of sounds at the extremes of the audible range, 20 and 20,000 Hz, in 30.0ºC 30.0ºC air. (Assume that the frequency values are accurate to two significant figures.)
To find wavelength from frequency, we can use v w = fλ v w = fλ size 12{v size 8{w}=fλ} {} .
- Identify knowns. The value for v w v w size 12{v size 8{w}} {} , is given by v w = 331 m/s T 273 K . v w = 331 m/s T 273 K . size 12{v size 8{w}= left ("331"" m/s" right ) sqrt { { {T} over {"273"" K"} } } } {} 17.5
- Convert the temperature into kelvin and then enter the temperature into the equation v w = 331 m/s 303 K 273 K = 348 . 7 m/s . v w = 331 m/s 303 K 273 K = 348 . 7 m/s . 17.6
- Solve the relationship between speed and wavelength for λ λ size 12{λ} {} : λ = v w f . λ = v w f . size 12{λ= { {v size 8{w}} over {f} } } {} 17.7
- Enter the speed and the minimum frequency to give the maximum wavelength: λ max = 348 . 7 m/s 20 Hz = 17 m . λ max = 348 . 7 m/s 20 Hz = 17 m . size 12{λ size 8{"max"}= { {"348" "." 7" m/s"} over {"20 Hz"} } ="17"" m"} {} 17.8
- Enter the speed and the maximum frequency to give the minimum wavelength: λ min = 348 . 7 m/s 20 , 000 Hz = 0 . 017 m = 1 . 7 cm . λ min = 348 . 7 m/s 20 , 000 Hz = 0 . 017 m = 1 . 7 cm . size 12{λ size 8{"min"}= { {"348" "." 7" m/s"} over {"20" "." "000 Hz"} } =0 "." "0174"" m"=1 "." "74 cm"} {} 17.9
Because the product of f f size 12{f} {} multiplied by λ λ size 12{λ} {} equals a constant, the smaller f f size 12{f} {} is, the larger λ λ size 12{λ} {} must be, and vice versa.
The speed of sound can change when sound travels from one medium to another. However, the frequency usually remains the same because it is like a driven oscillation and has the frequency of the original source. If v w v w size 12{v size 8{w}} {} changes and f f size 12{f} {} remains the same, then the wavelength λ λ size 12{λ} {} must change. That is, because v w = fλ v w = fλ , the higher the speed of a sound, the greater its wavelength for a given frequency.
Making Connections: Take-Home Investigation—Voice as a Sound Wave
Suspend a sheet of paper so that the top edge of the paper is fixed and the bottom edge is free to move. You could tape the top edge of the paper to the edge of a table. Gently blow near the edge of the bottom of the sheet and note how the sheet moves. Speak softly and then louder such that the sounds hit the edge of the bottom of the paper, and note how the sheet moves. Explain the effects.
Check Your Understanding
Imagine you observe two fireworks explode. You hear the explosion of one as soon as you see it. However, you see the other firework for several milliseconds before you hear the explosion. Explain why this is so.
Sound and light both travel at definite speeds. The speed of sound is slower than the speed of light. The first firework is probably very close by, so the speed difference is not noticeable. The second firework is farther away, so the light arrives at your eyes noticeably sooner than the sound wave arrives at your ears.
You observe two musical instruments that you cannot identify. One plays high-pitch sounds and the other plays low-pitch sounds. How could you determine which is which without hearing either of them play?
Compare their sizes. High-pitch instruments are generally smaller than low-pitch instruments because they generate a smaller wavelength.
As an Amazon Associate we earn from qualifying purchases.
This book may not be used in the training of large language models or otherwise be ingested into large language models or generative AI offerings without OpenStax's permission.
Want to cite, share, or modify this book? This book uses the Creative Commons Attribution License and you must attribute OpenStax.
Access for free at https://openstax.org/books/college-physics/pages/1-introduction-to-science-and-the-realm-of-physics-physical-quantities-and-units
- Authors: Paul Peter Urone, Roger Hinrichs
- Publisher/website: OpenStax
- Book title: College Physics
- Publication date: Jun 21, 2012
- Location: Houston, Texas
- Book URL: https://openstax.org/books/college-physics/pages/1-introduction-to-science-and-the-realm-of-physics-physical-quantities-and-units
- Section URL: https://openstax.org/books/college-physics/pages/17-2-speed-of-sound-frequency-and-wavelength
© Mar 3, 2022 OpenStax. Textbook content produced by OpenStax is licensed under a Creative Commons Attribution License . The OpenStax name, OpenStax logo, OpenStax book covers, OpenStax CNX name, and OpenStax CNX logo are not subject to the Creative Commons license and may not be reproduced without the prior and express written consent of Rice University.
Module 7 – Wave Motion and Sound
Module 7 speed and frequency of sound, speed of sound, frequency, and wavelength, learning objectives.
By the end of this section, you will be able to:
- Define pitch.
- Describe the relationship between the speed of sound, its frequency, and its wavelength.
- Describe the effects on the speed of sound as it travels through various media.
- Describe the effects of temperature on the speed of sound.
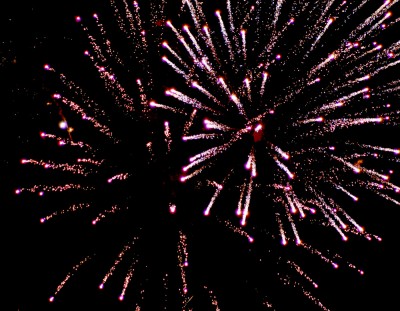
Figure 1. When a firework explodes, the light energy is perceived before the sound energy. Sound travels more slowly than light does. (credit: Dominic Alves, Flickr)
Sound, like all waves, travels at a certain speed and has the properties of frequency and wavelength. You can observe direct evidence of the speed of sound while watching a fireworks display. The flash of an explosion is seen well before its sound is heard, implying both that sound travels at a finite speed and that it is much slower than light. You can also directly sense the frequency of a sound. Perception of frequency is called pitch . The wavelength of sound is not directly sensed, but indirect evidence is found in the correlation of the size of musical instruments with their pitch. Small instruments, such as a piccolo, typically make high-pitch sounds, while large instruments, such as a tuba, typically make low-pitch sounds. High pitch means small wavelength, and the size of a musical instrument is directly related to the wavelengths of sound it produces. So a small instrument creates short-wavelength sounds. Similar arguments hold that a large instrument creates long-wavelength sounds.
The relationship of the speed of sound, its frequency, and wavelength is the same as for all waves: v w = fλ, where v w is the speed of sound, f is its frequency, and λ is its wavelength. The wavelength of a sound is the distance between adjacent identical parts of a wave—for example, between adjacent compressions as illustrated in Figure 2. The frequency is the same as that of the source and is the number of waves that pass a point per unit time.
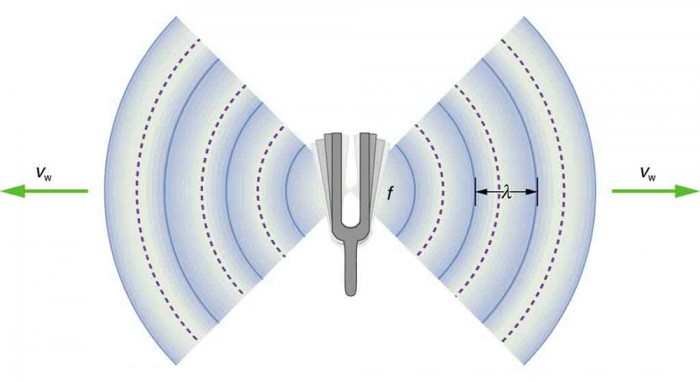
Figure 2. A sound wave emanates from a source vibrating at a frequency f , propagates at V w , and has a wavelength λ .
Table 1 makes it apparent that the speed of sound varies greatly in different media. The speed of sound in a medium is determined by a combination of the medium’s rigidity (or compressibility in gases) and its density. The more rigid (or less compressible) the medium, the faster the speed of sound. This observation is analogous to the fact that the frequency of a simple harmonic motion is directly proportional to the stiffness of the oscillating object. The greater the density of a medium, the slower the speed of sound. This observation is analogous to the fact that the frequency of a simple harmonic motion is inversely proportional to the mass of the oscillating object. The speed of sound in air is low, because air is compressible. Because liquids and solids are relatively rigid and very difficult to compress, the speed of sound in such media is generally greater than in gases.
Earthquakes, essentially sound waves in Earth’s crust, are an interesting example of how the speed of sound depends on the rigidity of the medium. Earthquakes have both longitudinal and transverse components, and these travel at different speeds. The bulk modulus of granite is greater than its shear modulus. For that reason, the speed of longitudinal or pressure waves (P-waves) in earthquakes in granite is significantly higher than the speed of transverse or shear waves (S-waves). Both components of earthquakes travel slower in less rigid material, such as sediments. P-waves have speeds of 4 to 7 km/s, and S-waves correspondingly range in speed from 2 to 5 km/s, both being faster in more rigid material. The P-wave gets progressively farther ahead of the S-wave as they travel through Earth’s crust. The time between the P- and S-waves is routinely used to determine the distance to their source, the epicenter of the earthquake.
The speed of sound is affected by temperature in a given medium. For air at sea level, the speed of sound is given by
[latex]v_{\text{w}}=\left(331\text{ m/s}\right)\sqrt{\frac{T}{273\text{ K}}}\\[/latex],
where the temperature (denoted as T ) is in units of kelvin. The speed of sound in gases is related to the average speed of particles in the gas, v rms , and that
[latex]v_{\text{rms}}=\sqrt{\frac{3kT}{m}}\\[/latex],
where k is the Boltzmann constant (1.38 × 10 −23 J/K) and m is the mass of each (identical) particle in the gas. So, it is reasonable that the speed of sound in air and other gases should depend on the square root of temperature. While not negligible, this is not a strong dependence. At 0ºC , the speed of sound is 331 m/s, whereas at 20.0ºC it is 343 m/s, less than a 4% increase. Figure 3 shows a use of the speed of sound by a bat to sense distances. Echoes are also used in medical imaging.
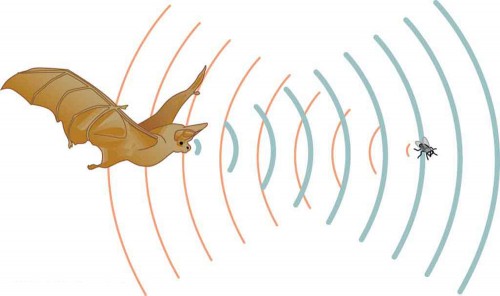
Figure 3. A bat uses sound echoes to find its way about and to catch prey. The time for the echo to return is directly proportional to the distance.
One of the more important properties of sound is that its speed is nearly independent of frequency. This independence is certainly true in open air for sounds in the audible range of 20 to 20,000 Hz. If this independence were not true, you would certainly notice it for music played by a marching band in a football stadium, for example. Suppose that high-frequency sounds traveled faster—then the farther you were from the band, the more the sound from the low-pitch instruments would lag that from the high-pitch ones. But the music from all instruments arrives in cadence independent of distance, and so all frequencies must travel at nearly the same speed. Recall that
v w = fλ.
In a given medium under fixed conditions, v w is constant, so that there is a relationship between f and λ ; the higher the frequency, the smaller the wavelength. See Figure 4 and consider the following example.
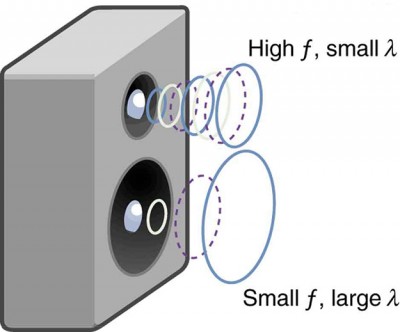
Figure 4. Because they travel at the same speed in a given medium, low-frequency sounds must have a greater wavelength than high-frequency sounds. Here, the lower-frequency sounds are emitted by the large speaker, called a woofer, while the higher-frequency sounds are emitted by the small speaker, called a tweeter.
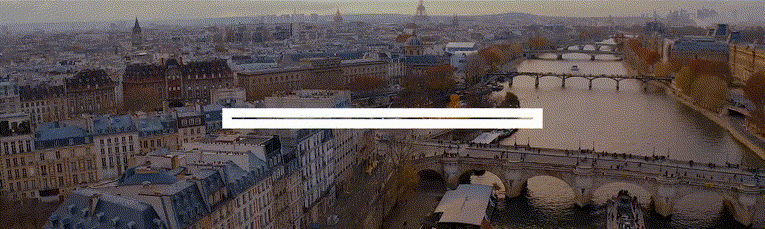
Example 1. Calculating Wavelengths: What Are the Wavelengths of Audible Sounds?
Calculate the wavelengths of sounds at the extremes of the audible range, 20 and 20,000 Hz, in 30.0ºC air. (Assume that the frequency values are accurate to two significant figures.)
To find wavelength from frequency, we can use v w = fλ .
1. Identify knowns. The value for v w , is given by
[latex]v_{\text{w}}=\left(331\text{ m/s}\right)\sqrt{\frac{T}{273\text{ K}}}\\[/latex].
2. Convert the temperature into kelvin and then enter the temperature into the equation
[latex]v_{\text{w}}=\left(331\text{ m/s}\right)\sqrt{\frac{303\text{ K}}{273\text{ K}}}=348.7\text{ m/s}\\[/latex].
3. Solve the relationship between speed and wavelength for λ :
[latex]\lambda=\frac{v_{\text{w}}}{f}\\[/latex].
4. Enter the speed and the minimum frequency to give the maximum wavelength:
[latex]\lambda_{\text{max}}=\frac{348.7\text{ m/s}}{20\text{ Hz}}=17\text{ m}\\[/latex].
5. Enter the speed and the maximum frequency to give the minimum wavelength:
[latex]\lambda_{\text{min}}=\frac{348.7\text{ m/s}}{20,000\text{ Hz}}=0.017\text{ m}=1.7\text{ cm}\\[/latex].
Because the product of f multiplied by λ equals a constant, the smaller f is, the larger λ must be, and vice versa.
The speed of sound can change when sound travels from one medium to another. However, the frequency usually remains the same because it is like a driven oscillation and has the frequency of the original source. If v w changes and f remains the same, then the wavelength λ must change. That is, because v w = fλ , the higher the speed of a sound, the greater its wavelength for a given frequency.
Making Connections: Take-Home Investigation—Voice as a Sound Wave
Suspend a sheet of paper so that the top edge of the paper is fixed and the bottom edge is free to move. You could tape the top edge of the paper to the edge of a table. Gently blow near the edge of the bottom of the sheet and note how the sheet moves. Speak softly and then louder such that the sounds hit the edge of the bottom of the paper, and note how the sheet moves. Explain the effects.
Check Your Understanding
Imagine you observe two fireworks explode. You hear the explosion of one as soon as you see it. However, you see the other firework for several milliseconds before you hear the explosion. Explain why this is so.
Sound and light both travel at definite speeds. The speed of sound is slower than the speed of light. The first firework is probably very close by, so the speed difference is not noticeable. The second firework is farther away, so the light arrives at your eyes noticeably sooner than the sound wave arrives at your ears.
You observe two musical instruments that you cannot identify. One plays high-pitch sounds and the other plays low-pitch sounds. How could you determine which is which without hearing either of them play?
Compare their sizes. High-pitch instruments are generally smaller than low-pitch instruments because they generate a smaller wavelength.
Section Summary
- The relationship of the speed of sound v w , its frequency f , and its wavelength λ is given by v w fλ , which is the same relationship given for all waves.
- In air, the speed of sound is related to air temperature T by [latex]v_{\text{w}}=\left(\text{331}\text{m/s}\right)\sqrt{\frac{T}{\text{273}\text{K}}}\\[/latex]. v w is the same for all frequencies and wavelengths.
Conceptual Questions
- How do sound vibrations of atoms differ from thermal motion?
- When sound passes from one medium to another where its propagation speed is different, does its frequency or wavelength change? Explain your answer briefly.
Problems & Exercises
- When poked by a spear, an operatic soprano lets out a 1200-Hz shriek. What is its wavelength if the speed of sound is 345 m/s?
- What frequency sound has a 0.10-m wavelength when the speed of sound is 340 m/s?
- Calculate the speed of sound on a day when a 1500 Hz frequency has a wavelength of 0.221 m.
- (a) What is the speed of sound in a medium where a 100-kHz frequency produces a 5.96-cm wavelength? (b) Which substance in Table 1 is this likely to be?
- Show that the speed of sound in 20.0ºC air is 343 m/s, as claimed in the text.
- Air temperature in the Sahara Desert can reach 56.0ºC (about 134ºF). What is the speed of sound in air at that temperature?
- Dolphins make sounds in air and water. What is the ratio of the wavelength of a sound in air to its wavelength in seawater? Assume air temperature is 20.0ºC.
- A sonar echo returns to a submarine 1.20 s after being emitted. What is the distance to the object creating the echo? (Assume that the submarine is in the ocean, not in fresh water.)
- (a) If a submarine’s sonar can measure echo times with a precision of 0.0100 s, what is the smallest difference in distances it can detect? (Assume that the submarine is in the ocean, not in fresh water.) (b) Discuss the limits this time resolution imposes on the ability of the sonar system to detect the size and shape of the object creating the echo.
- A physicist at a fireworks display times the lag between seeing an explosion and hearing its sound, and finds it to be 0.400 s. (a) How far away is the explosion if air temperature is [latex]\text{24.0\textordmasculine C}[/latex] and if you neglect the time taken for light to reach the physicist? (b) Calculate the distance to the explosion taking the speed of light into account. Note that this distance is negligibly greater.
- Suppose a bat uses sound echoes to locate its insect prey, 3.00 m away. (See Figure 3.) (a) Calculate the echo times for temperatures of 5.00ºC and 35.0ºC. (b) What percent uncertainty does this cause for the bat in locating the insect? (c) Discuss the significance of this uncertainty and whether it could cause difficulties for the bat. (In practice, the bat continues to use sound as it closes in, eliminating most of any difficulties imposed by this and other effects, such as motion of the prey.)
pitch: the perception of the frequency of a sound
Selected Solutions to Problems & Exercises
5. [latex]\begin{array}{lll}{v}_{\text{w}}& =& \left(\text{331 m/s}\right)\sqrt{\frac{T}{\text{273 K}}}=\left(\text{331 m/s}\right)\sqrt{\frac{\text{293 K}}{\text{273 K}}}\\ & =& \text{343 m/s}\end{array}\\[/latex]
9. (a) 7.70 m; (b) This means that sonar is good for spotting and locating large objects, but it isn’t able to resolve smaller objects, or detect the detailed shapes of objects. Objects like ships or large pieces of airplanes can be found by sonar, while smaller pieces must be found by other means.
11. (a) 18.0 ms, 17.1 ms; (b) 5.00%; (c) This uncertainty could definitely cause difficulties for the bat, if it didn’t continue to use sound as it closed in on its prey. A 5% uncertainty could be the difference between catching the prey around the neck or around the chest, which means that it could miss grabbing its prey.
- College Physics. Authored by : OpenStax College. Located at : http://cnx.org/contents/031da8d3-b525-429c-80cf-6c8ed997733a/College_Physics . License : CC BY: Attribution . License Terms : Located at License
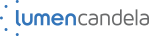
Privacy Policy
NOTIFICATIONS
Fundamentals of waves.
- + Create new collection
Seeing, hearing, feeling warmth, surfing, tuning the radio, using a cellphone – these and many more activities involve waves. But what is a wave? In this article, we get to grips with the different kinds of waves and look at the key characteristics of all waves – their wavelength, period, frequency, speed and amplitude. These concepts are important for describing waves of all kinds.
Different waves, same properties
Water waves are the focus of our tsunamis and surf resources, but many other kinds of wave exist. These include sound waves, light waves, radio waves, microwaves and others. All kinds of waves have the same fundamental properties of reflection, refraction, diffraction and interference, and all waves have a wavelength, frequency, speed and amplitude.
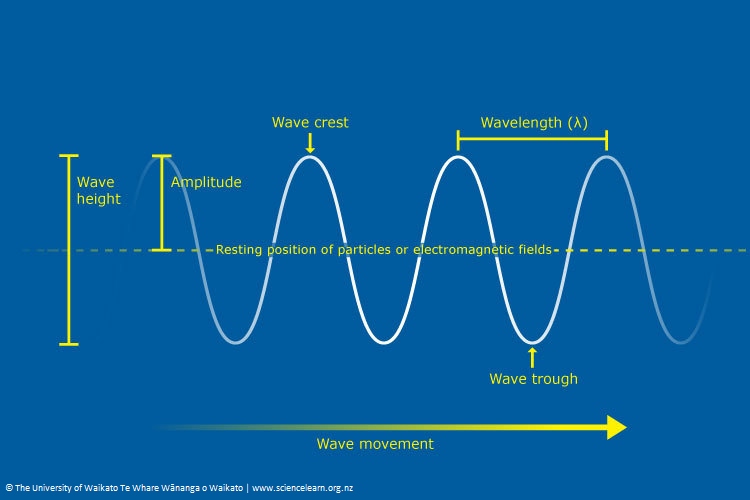
Wave length, height and frequency
A wave can be described by its length, height (amplitude) and frequency.
All waves can be thought of as a disturbance that transfers energy.
Some waves (water waves and sound waves) are formed through the vibration of particles. Waves form because water molecules are disturbed, and sound waves are formed by the disturbance of air particles or particles in an object through which sound is travelling, like a door.
Electromagnetic waves (such as light waves, UV radiation, microwaves and others) are formed through oscillating electric and magnetic fields.
Waves have a defined wavelength
Every wave has a specific wavelength. This is defined as the length from one wave crest to the next.
Different kinds of waves have widely varying wavelengths. In water, surf waves have wavelengths of 30–50 m, and tsunamis have much longer wavelengths (about 100km). Sound waves vary in wavelength according to the pitch of the sound – humans can hear sound with wavelengths between 70 mm and 70 m. Different kinds of electromagnetic waves vary greatly in wavelength, from the long wavelength of radio waves (about 10 m) to the much shorter wavelengths of visible light (less than a millionth of a metre – usually described as hundreds of nanometres) and X-rays (less than a billionth of a metre).
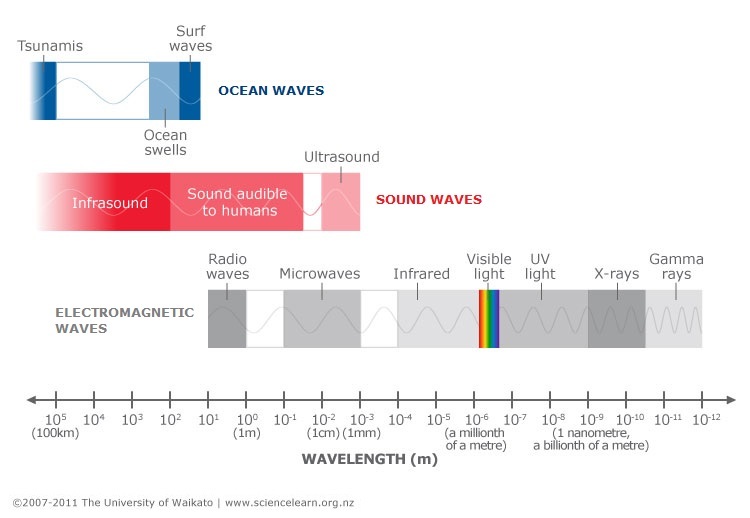
Wavelengths of different wave types
Every wave has a specific wavelength, but these vary widely between wave types – from hundreds of kilometres (tsunamis) to approximately a hundredth of a billionth of a metre (gamma rays).
Waves have a defined frequency
As well as a defined speed, every wave has a frequency. This is the number of wavelengths that travel past a point in 1 second of time. Frequency is measured in hertz (waves per second). For each type of wave, a longer wavelength means a lower frequency – for electromagnetic waves, for example, microwaves have a much lower frequency than UV waves.
Waves also have a defined period. This is the time it takes for the wave to undergo one complete oscillation or one complete motion. The wave period is inversely proportional to the frequency – the longer the period, the lower the frequency.
Wave speed is related to frequency and wavelength
Every wave travels at a particular speed. Water waves are unusual because waves can have different speeds – wave speed depends on how the wave is formed, which is why tsunamis travel much faster than surf waves. Unlike water waves, electromagnetic waves always travel at the same speed (3 hundred million metres per second) and sound waves all travel at the same speed in a given medium (for example, approximately 340 metres per second in air).
The speed of a wave is related to both its frequency and wavelength. The equation v = f x λ (speed = frequency x wavelength) describes this relationship and is useful for predicting the unknown characteristics of a wave.
For waves that always travel at the same speed (like electromagnetic waves), the equation can be used to work out the frequency or wavelength. High-frequency EM waves will have a short wavelength, and low-frequency waves will have longer wavelengths. For waves with a variable speed (such as water waves), the equation can be used to work out the speed from the frequency and wavelength.
All waves have an amplitude
The amplitude of a wave is normally defined as the maximum displacement of the particles within the wave from their normal equilibrium positions. For water waves, the amplitude of a wave is the distance between the wave crest and the normal water level.
People sometimes talk about the wave height, which is the vertical distance from the wave crest to the wave trough, instead of amplitude.
Nature of science
Common scientific concepts can connect seemingly disparate areas of science. For instance, water waves, sound waves and electromagnetic radiation all exhibit similar characteristics and behaviours. Some of the scientific knowledge obtained in one of these areas can therefore be applied to the others.
Related content
For a more simplified look at waves, see Waves and energy – wave basics .
Activity ideas
Use a Mexican wave to demonstrate how waves transfer energy and to help your students visualise the wave behaviours of reflection, constructive interference and shoaling.
This interactive or paper-based Venn diagram can be used to illustrate the key similarities and differences between tsunami waves and surf waves .
In the electromagnetic spectrum – picture dictation activity, students use a range of skills to investigate some ways humans use the waves on the electromagnetic spectrum.
See our newsletters here .
Would you like to take a short survey?
This survey will open in a new tab and you can fill it out after your visit to the site.

- Science Notes Posts
- Contact Science Notes
- Todd Helmenstine Biography
- Anne Helmenstine Biography
- Free Printable Periodic Tables (PDF and PNG)
- Periodic Table Wallpapers
- Interactive Periodic Table
- Periodic Table Posters
- How to Grow Crystals
- Chemistry Projects
- Fire and Flames Projects
- Holiday Science
- Chemistry Problems With Answers
- Physics Problems
- Unit Conversion Example Problems
- Chemistry Worksheets
- Biology Worksheets
- Periodic Table Worksheets
- Physical Science Worksheets
- Science Lab Worksheets
- My Amazon Books
Speed of Sound in Physics

In physics, the speed of sound is the distance traveled per unit of time by a sound wave through a medium. It is highest for stiff solids and lowest for gases. There is no sound or speed of sound in a vacuum because sound (unlike light ) requires a medium in order to propogate.
What Is the Speed of Sound?
Usually, conversations about the speed of sound refer to the speed of sound of dry air (humidity changes the value). The value depends on temperature.
- at 20 ° C or 68 ° F: 343 m/s or 1234.8 kph or 1125ft/s or 767 mph
- at 0 ° C or 32 ° F: 331 m/s or 1191.6 kph or 1086 ft/s or 740 mph
Mach Numher
The Mach number is the ratio of air speed to the speed of sound. So, an object at Mach 1 is traveling at the speed of sound. Exceeding Mach 1 is breaking the sound barrier or is supersonic . At Mach 2, the object travels twice the speed of sound. Mach 3 is three times the speed of sound, and so on.
Remember that the speed of sound depends on temperature, so you break sound barrier at a lower speed when the temperature is colder. To put it another way, it gets colder as you get higher in the atmosphere, so an aircraft might break the sound barrier at a higher altitude even if it does not increase its speed.
Solids, Liquids, and Gases
The speed of sound is greatest for solids, intermediate for liquids, and lowest for gases:
v solid > v liquid >v gas
Particles in a gas undergo elastic collisions and the particles are widely separated. In contrast, particles in a solid are locked into place (rigid or stiff), so a vibration readily transmits through chemical bonds.
Here are examples of the difference between the speed of sound in different materials:
- Diamond (solid): 12000 m/s
- Copper (solid): 6420 m/s
- Iron (solid): 5120 m/s
- Water (liquid) 1481 m/s
- Helium (gas): 965 m/s
- Dry air (gas): 343 m/s
Sounds waves transfer energy to matter via a compression wave (in all phases) and also shear wave (in solids). The pressure disturbs a particle, which then impacts its neighbor, and continues traveling through the medium. The speed is how quickly the wave moves, while the frequency is the number of vibrations the particle makes per unit of time.
The Hot Chocolate Effect
The hot chocolate effect describes the phenomenon where the pitch you hear from tapping a cup of hot liquid rises after adding a soluble powder (like cocoa powder into hot water). Stirring in the powder introduces gas bubbles that reduce the speed of sound of the liquid and lower the frequency (pitch) of the waves. Once the bubbles clear, the speed of sound and the frequency increase again.
Speed of Sound Formulas
There are several formulas for calculating the speed of sound. Here are a few of the most common ones:
For gases these approximations work in most situations:
For this formula, use the Celsius temperature of the gas.
v = 331 m/s + (0.6 m/s/C)•T
Here is another common formula:
v = (γRT) 1/2
- γ is the ratio of specific heat values or adiabatic index (1.4 for air at STP )
- R is a gas constant (282 m 2 /s 2 /K for air)
- T is the absolute temperature (Kelvin)
The Newton-Laplace formula works for both gases and liquids (fluids):
v = (K s /ρ) 1/2
- K s is the coefficient of stiffness or bulk modulus of elasticity for gases
- ρ is the density of the material
So solids, the situation is more complicated because shear waves play into the formula. There can be sound waves with different velocities, depending on the mode of deformation. The simplest formula is for one-dimensional solids, like a long rod of a material:
v = (E/ρ) 1/2
- E is Young’s modulus
Note that the speed of sound decreases with density! It increases according to the stiffness of a medium. This is not intuitively obvious, since often a dense material is also stiff. But, consider that the speed of sound in a diamond is much faster than the speed in iron. Diamond is less dense than iron and also stiffer.
Factors That Affect the Speed of Sound
The primary factors affecting the speed of sound of a fluid (gas or liquid) are its temperature and its chemical composition. There is a weak dependence on frequency and atmospheric pressure that is omitted from the simplest equations.
While sound travels only as compression waves in a fluid, it also travels as shear waves in a solid. So, a solid’s stiffness, density, and compressibility also factor into the speed of sound.
Speed of Sound on Mars
Thanks to the Perseverance rover, scientists know the speed of sound on Mars. The Martian atmosphere is much colder than Earth’s, its thin atmosphere has a much lower pressure, and it consists mainly of carbon dioxide rather than nitrogen. As expected, the speed of sound on Mars is slower than on Earth. It travels at around 240 m/s or about 30% slower than on Earth.
What scientists did not expect is that the speed of sound varies for different frequencies. A high pitched sound, like from the rover’s laser, travels faster at around 250 m/s. So, for example, if you listened to a symphony recording from a distance on Mars you’d hear the various instruments at different times. The explanation has to do with the vibrational modes of carbon dioxide, the primary component of the Martian atmosphere. Also, it’s worth noting that the atmospheric pressure is so low that there really isn’t any much sound at all from a source more than a few meters away.
Speed of Sound Example Problems
Find the speed of sound on a cold day when the temperature is 2 ° C.
The simplest formula for finding the answer is the approximation:
v = 331 m/s + (0.6 m/s/C) • T
Since the given temperature is already in Celsius, just plug in the value:
v = 331 m/s + (0.6 m/s/C) • 2 C = 331 m/s + 1.2 m/s = 332.2 m/s
You’re hiking in a canyon, yell “hello”, and hear an echo after 1.22 seconds. The air temperature is 20 ° C. How far away is the canyon wall?
The first step is finding the speed of sound at the temperature:
v = 331 m/s + (0.6 m/s/C) • T v = 331 m/s + (0.6 m/s/C) • 20 C = 343 m/s (which you might have memorized as the usual speed of sound)
Next, find the distance using the formula:
d = v• T d = 343 m/s • 1.22 s = 418.46 m
But, this is the round-trip distance! The distance to the canyon wall is half of this or 209 meters.
If you double the frequency of sound, it double the speed of its waves. True or false?
This is (mostly) false. Doubling the frequency halves the wavelength, but the speed depends on the properties of the medium and not its frequency or wavelength. Frequency only affects the speed of sound for certain media (like the carbon dioxide atmosphere of Mars).
- Everest, F. (2001). The Master Handbook of Acoustics . New York: McGraw-Hill. ISBN 978-0-07-136097-5.
- Kinsler, L.E.; Frey, A.R.; Coppens, A.B.; Sanders, J.V. (2000). Fundamentals of Acoustics (4th ed.). New York: John Wiley & Sons. ISBN 0-471-84789-5.
- Maurice, S.; et al. (2022). “In situ recording of Mars soundscape:. Nature. 605: 653-658. doi: 10.1038/s41586-022-04679-0
- Wong, George S. K.; Zhu, Shi-ming (1995). “Speed of sound in seawater as a function of salinity, temperature, and pressure”. The Journal of the Acoustical Society of America . 97 (3): 1732. doi: 10.1121/1.413048
Related Posts
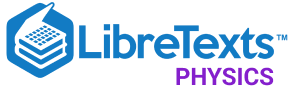
- school Campus Bookshelves
- menu_book Bookshelves
- perm_media Learning Objects
- login Login
- how_to_reg Request Instructor Account
- hub Instructor Commons
- Download Page (PDF)
- Download Full Book (PDF)
- Periodic Table
- Physics Constants
- Scientific Calculator
- Reference & Cite
- Tools expand_more
- Readability
selected template will load here
This action is not available.
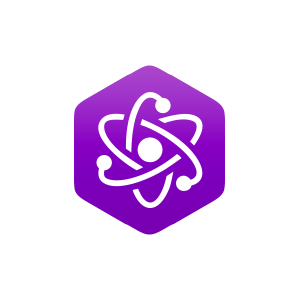
5.1.1: Speeds of Different Types of Waves
- Last updated
- Save as PDF
- Page ID 26167

- Kyle Forinash and Wolfgang Christian
The speed of a wave is fixed by the type of wave and the physical properties of the medium in which it travels. An exception is electromagnetic waves which can travel through a vacuum. For most substances the material will vibrate obeying a Hooke's law force as a wave passes through it and the speed will not depend on frequency. Electromagnetic waves in a vacuum and waves traveling though a linear medium are termed linear waves and have constant speed. Examples:
- For sound waves in a fluid (for example air or water) the speed is determined by \(v=(B/\rho )^{1/2}\) where \(B\) is the bulk modulus or compressibility of the fluid in newtons per meter squared and \(\rho\) is the density in kilograms per cubic meter.
- For sound waves in a solid the speed is determined by \(v= (Y/\rho )^{1/2}\) where \(Y\) is Young's modulus or stiffness in Newtons per meter squared and \(\rho\) is the density in kilograms per meter cubed.
- For waves on a string the speed is determined by \(v=(T/\mu )^{1/2}\) where \(T\) is the tension in the string in Newtons and \(\mu\) is the mass per length in kilograms per meter.
- Although electromagnetic waves do not need a medium to travel (they can travel through a vacuum) their speed in a vacuum, \(c = (1/\mu _{o} ε_{o})^{1/2} = 3.0\times 10^{8}\text{ m/s}\) is governed by two physical constants, the permeability \(\mu_{o}\) and the permittivity, \(ε_{o}\) of free space (vacuum).
Table \(\PageIndex{1}\)
Here is a more comprehensive list of the speed of sound in various materials .
As we saw in the previous chapter, there is a relationship between the period, wavelength and speed of the wave. The period of a cork floating in the water is affected by how fast the wave passes (wave speed) and the distance between peaks (wavelength). The relationship between speed, period and wavelength of a sine wave is given by \(v=\lambda /T\) where wavelength and period for a sine wave were defined previously. This can also be written as \(v=\lambda f\) since frequency is the inverse of period and is true for all linear waves. Notice that, since wave speed is normally a fixed quantity the frequency and wavelength will be inversely proportion; higher frequencies mean shorter wavelengths.
Often it is easier to write \(ω = 2πf\) where \(\omega\) is the angular frequency in radians per second instead of having to write \(2\pi f\) everywhere. Likewise it is easier to write \(k=2\pi /\lambda \) where \(k\) is the wave number in radians per meter rather than having to write \(2\pi /\lambda\) a lot. (Note that \(k\) is not a spring constant here.) Using these new definitions the speed of a wave can also be written as \(v=f\lambda =\omega /k\).
If the medium is uniform the speed of a wave is fixed and does not change. There are circumstances where the speed of a particular wave does change, however. Notice that the speed of sound in air depends on the density of the air (mass per volume). But the density of air changes with temperature and humidity. So the speed of sound can be different on different days and in different locations. The temperature dependence of the speed of sound in air is given by \(v = 344 + 0.6 (T - 20)\) in meters per second where \(T\) is the temperature in Celsius (\(T\) here is temperature, not period). Notice that at room temperature (\(20^{\circ}\text{C}\)) sound travels at \(344\text{ m/s}\).
The speed of sound can also be affected by the movement of the medium in which it travels. For example, wind can carry sound waves further (i.e. faster) if the sound is traveling in the same direction or it can slow the sound down if the sound is traveling in a direction opposite to the wind direction.
Electromagnetic waves travel at \(\text{c} = 3.0\times 10^{8}\text{ m/s}\) in a vacuum but slow down when they pass through a medium (for example light passing from air to glass). This occurs because the material has a different value for the permittivity and/or permeability due to the interaction of the wave with the atoms of the material. The amount the speed changes is given by the index of refraction \(n=c/v\) where \(c\) is the speed of light in a vacuum and \(v\) is the speed in the medium. The frequency of the wave does not change when it slows down so, since \(v=\lambda f\), the wavelength of electromagnetic waves in a medium must be slightly smaller.
Video/audio examples:
- What is the speed of sound in a vacuum? Buzzer in a bell jar . Why is there no sound when the air is removed from the jar?
- Demonstration of speed of sound in different gasses . Why is there no sound when the air is removed from the jar?
- These two videos demonstrate the Allasonic effect. The speed of sound is different in a liquid with air bubbles because the density is different. As the bubbles burst, the speed of sound changes, causing the frequency of sound waves in the liquid column to change, thus changing the pitch. Example: one , two . What do you hear in each case?
- The Zube Tube is a toy that has a spring inside attached to two plastic cups on either end. Vibrations in the spring travel at different speeds so a sound starting at one end (for example a click when you shake the tube and the spring hits the cup) ends up changing pitch at the other end as the various frequencies arrive. In other words this is a nonlinear system. See if you can figure out from the video which frequencies travel faster, high frequencies or low.
Mini-lab on measuring the speed of sound .
Questions on Wave Speed:
\(f=1/T,\quad v=f\lambda ,\quad v=\omega /k,\quad k=2\pi /\lambda,\quad \omega =2\pi f,\quad y(x,t)=A\cos (kx-\omega t+\phi ),\quad v=\sqrt{B/Q}\)
- Light travels at \(3.0\times 10^{8}\text{ m/s}\) but sound waves travel at about \(344\text{ m/s}\). What is the time delay for light and sound to arrive from a source that is \(10,000\text{ m}\) away (this can be used to get an approximate distance to a thunderstorm)?
- What two mistakes are made in science fiction movies where you see and hear an explosion in space at the same time?
- Consult the table for the speed of sound in various substances. If you have one ear in the water and one ear out while swimming in a lake and a bell is rung that is half way in the water some distance away, which ear hears the sound first?
- At \(20\text{C}\) the speed of sound is \(344\text{ m/s}\). How far does sound travel in \(1\text{ s}\)? How far does sound travel in \(60\text{ s}\)?
- Compare the last two answers with the distance traveled by light which has a speed of \(3.0\times 10^{8}\text{ m/s}\). Why do you see something happen before you hear it?
- The speed of sound in water is \(1482\text{ m/s}\). How far does sound travel under water in \(1\text{ s}\)? How far does sound travel under water in \(60\text{ s}\)?
- What happens to the speed of sound in air as temperature increases?
- Using the equation for the speed of sound at different temperatures, what is the speed of sound on a hot day when the temperature is \(30^{\circ}\text{C}\)? Hint: \(v = 344\text{ m/s} + 0.6 (T - 20)\) where \(T\) is the temperature in Celsius.
- Using the speed of sound at \(30^{\circ}\text{C}\) from the last question, recalculate the distance traveled for the cases in question four.
- Suppose on a cold day the temperature is \(-10^{\circ}\text{C}\: (14^{\circ}\text{F}\)). You are playing in the marching band outside. How long does it take the sound from the band to reach the spectators if they are \(100\text{ m}\) away?
- What is the difference in the speed of sound in air on a hot day (\(40^{\circ}\text{C}\)) and a cold day (\(0^{\circ}\text{C}\))?
- What would an orchestra sound like if different instruments produced sounds that traveled at different speeds?
- The speed of a wave is fixed by the medium it travels in so, for a given situation, is usually constant. What happens to the frequency of a wave if the wavelength is doubled?
- What happens to the wavelength of a wave if the frequency is doubled and has the same speed?
- Suppose a sound wave has a frequency \(200\text{ Hz}\). If the speed of sound is \(343\text{ m/s}\), what wavelength is this wave?
- What factors determine the speed of sound in air?
- Why do sound waves travel faster through liquids than air?
- Why do sound waves travel faster through solids than liquids?
- The speed of sound in a fluid is given by \(v=\sqrt{B/Q}\) where \(B\) is the Bulk Modulus (compressibility) and \(Q\) is the density. What happens to the speed if the density of the fluid increases?
- What must be true about the compressibility, \(B\), of water versus air, given that sound travels faster in water and water is denser than air?
- The speed of sound in a fluid is given by \(v=\sqrt{B/Q}\) where \(B\) is the Bulk Modulus (compressibility) and \(Q\) is the density. Can you think of a clever way to measure the Bulk Modulus of a fluid if you had an easy way to measure the speed of sound in a fluid? Explain.
- The speed of sound on a string is given by \(v=\sqrt{T/\mu}\) where \(T\) is the tension in Newtons and \(\mu\) is the linear density (thickness) in \(\text{kg/m}\). You also know that \(v=f\lambda\). Give two ways of changing the frequency of vibration of a guitar string based on the knowledge of these two equations.
- For the previous question, increasing the tension does what to the frequency? What does using a denser string do to the frequency?
- The following graph is of a wave, frozen in time at \(t = 0\). The equation describing the wave is \(y(x,t)=A\cos (kx-\omega t+\phi )\). Sketch the effect of doubling the amplitude, \(A\).
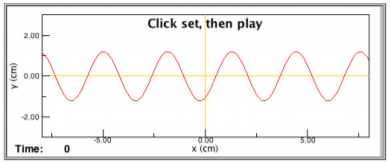
Figure \(\PageIndex{1}\)
- For the following graph of a wave, sketch the effect of doubling the wavelength.
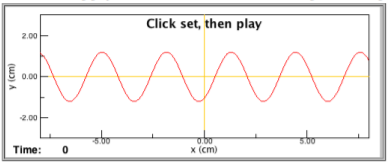
Figure \(\PageIndex{2}\)
- The mathematical description of a sine wave is given by \(y(x,t)=A\cos (kx-\omega t+\phi )\). Explain what each of the terms \((A, k, \omega, \phi )\) represent.
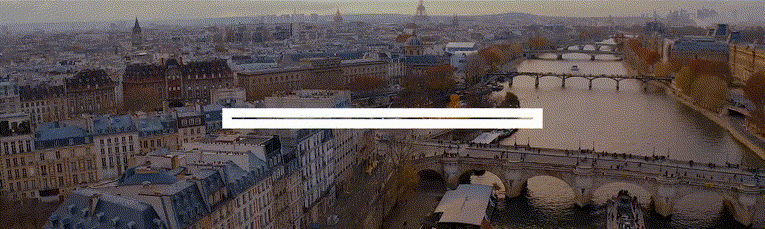
IMAGES
VIDEO
COMMENTS
The relationship of the speed of sound, its frequency, and wavelength is the same as for all waves: vw = fλ, (17.2.1) (17.2.1) v w = f λ, where vw v w is the speed of sound, f f is its frequency, and λ λ is its wavelength. The wavelength of a sound is the distance between adjacent identical parts of a wave—for example, between adjacent ...
14.1. where v is the speed of sound (in units of m/s), f is its frequency (in units of hertz), and λ λ is its wavelength (in units of meters). Recall that wavelength is defined as the distance between adjacent identical parts of a wave. The wavelength of a sound, therefore, is the distance between adjacent identical parts of a sound wave.
4 Answers. Star Wars. In fact, assuming an ideal gas, the speed of sound c depends on temperature only, not on the pressure or density (since these change in lockstep for a given temperature and cancel out). Air is almost an ideal gas. The temperature of the air varies with altitude, giving the following variations in the speed of sound using ...
Figure 17.10 Because they travel at the same speed in a given medium, low-frequency sounds must have a greater wavelength than high-frequency sounds. ... The speed of sound can change when sound travels from one medium to another, but the frequency usually remains the same. This is similar to the frequency of a wave on a string being equal to ...
The speed of the waves is determined by the properties of the water itself. The shorter wavelength sound has the higher frequency. Both sounds travel at the same speed. When the sound goes from cooler to warmer air, its speed increases (because sound travels faster in warmer air). The frequency doesn't change (unless the source changes).
Measuring waves. All sound waves are the same: they travel through a medium by making atoms or molecules shake back and forth. But all sound waves are different too. There are loud sounds and quiet sounds, high-pitched squeaks and low-pitched rumbles, and even two instruments playing exactly the same musical note will produce sound waves that are quite different.
As sound travels, its amplitude decreases- but that's not the same thing as slowing down. Sound (in air) covers roughly 340 meters each and every second, even as its amplitude shrinks. Eventually, the amplitude gets small enough that the sound is undetectable. A sound's amplitude shrinks as it travels, but its speed remains constant.
We can find the speed of sound by looking at the speed of this compressed region as it travels through the medium. In non-humid air at 20 degrees Celsius, the speed of sound is about 343 meters per second or 767 miles per hour. We can also watch the speed of sound of a repeating simple harmonic wave.
Figure 17.10 Because they travel at the same speed in a given medium, low-frequency sounds must have a greater wavelength than high-frequency sounds. Here, the lower-frequency sounds are emitted by the large speaker, called a woofer, while the higher-frequency sounds are emitted by the small speaker, called a tweeter.
Section Summary. The relationship of the speed of sound vw, its frequency f, and its wavelength λ is given by vwfλ , which is the same relationship given for all waves. In air, the speed of sound is related to air temperature T by vw = (331m/s)√ T 273K v w = ( 331 m/s) T 273 K . vw is the same for all frequencies and wavelengths.
No, it's not. Not at all. Doubling the density of air increases its speed of sound by around 0.14%. Cooling air to half of its temperature, which also doubles the density, reduces the speed of sound by over 30%. So the impact of temperature is the opposite of the impact of density, and between the two the impact of temperature is way more ...
speed of sound changes slightly at different temperatures, and dramatically in different materials (for example, in steel alloy, it travels 6000 m/s and in fresh water at 25 degrees F, it travels at 1497 m/s). As long as a sound wave is moving through the same medium, it maintains a constant speed. Figure 1: Longitudinal Waves
The speed of sound is the distance travelled per unit of time by a sound wave as it propagates through an elastic medium. At 20 °C (68 °F), the speed of sound in air is about 343 m/s (1,125 ft/s; 1,235 km/h; 767 mph; 667 kn), or one km in 2.91 s or one mile in 4.69 s.It depends strongly on temperature as well as the medium through which a sound wave is propagating.
Unlike water waves, electromagnetic waves always travel at the same speed (3 hundred million metres per second) and sound waves all travel at the same speed in a given medium (for example, approximately 340 metres per second in air). The speed of a wave is related to both its frequency and wavelength. The equation v = f x λ (speed = frequency ...
The speed of sound in dry air at room temperature is 343 m/s or 1125 ft/s. In physics, the speed of sound is the distance traveled per unit of time by a sound wave through a medium. It is highest for stiff solids and lowest for gases. There is no sound or speed of sound in a vacuum because sound (unlike light) requires a medium in order to ...
The analogous terms for sound waves are ultrasound, acoustic and infrasound. A gamma waves oscillates faster than a radio wave but propagates at the same speed. It is possible for some sound and EM waves to have the same frequency, but their speed (and therefore wavelengths) will be different because they do not propagate in the same medium.
The speed of sound can also be affected by the movement of the medium in which it travels. For example, wind can carry sound waves further (i.e. faster) if the sound is traveling in the same direction or it can slow the sound down if the sound is traveling in a direction opposite to the wind direction.
The odd angle collisions limit the speed of far away particles and so the speed at which these waves travel, but the number of new pressure zones per second must remain more or less constant. Essentially, the frequency of vibrations has increased and the frequency of a pressure wave is its pitch.
$\begingroup$ It makes all the difference if this vehicle is open, like in the drawing, or closed, like a car. Sound waves travel on a medium, and it is relative to the average speed of that medium. If the vehicle is closed, the air moves with it, so the speed of sound is relative to the vehicle.