CPT Changes
Current book and archives back to 2000 Easy-to-read online book format Linked to and from code details
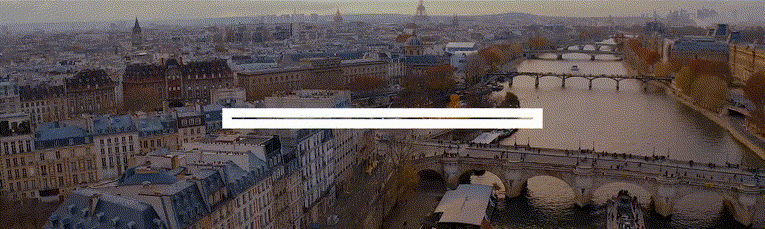
21. Factors influencing health status and contact with health services (Z00-Z99)
Z77-z99 persons with potential health hazards related to family and personal history and certain conditions influencing health status, z91 personal risk factors, not elsewhere classified, z91.8 other specified personal risk factors, not elsewhere classified, z91.83 wandering in diseases classified elsewhere....
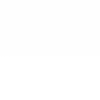
ICD-10-CM Diagnosis Codes
Z91.83 - Wandering in diseases classified elsewhere The above description is abbreviated. This code description may also have Includes , Excludes , Notes, Guidelines, Examples and other information. Access to this feature is available in the following products: Find-A-Code Essentials HCC Plus Find-A-Code Elite Find-A-Code Premium Find-A-Code Professional Find-A-Code Facility Base Find-A-Code Facility Complete Find-A-Code Facility Plus The above description is abbreviated. This code description may also have Includes , Excludes , Notes, Guidelines, Examples and other information. sign IN sign UP

- Find-A-Code Elite
- Find-A-Code Premium
- Find-A-Code Professional
- Find-A-Code Facility Base
- Find-A-Code Facility Complete
- Find-A-Code Facility Plus
Thank you for choosing Find-A-Code, please Sign In to remove ads.

Click through the PLOS taxonomy to find articles in your field.
For more information about PLOS Subject Areas, click here .
Loading metrics
Open Access
Peer-reviewed
Research Article
Prevalence of Sleepwalking: A Systematic Review and Meta-Analysis
* E-mail: [email protected]
Affiliation Centre for Sleep Research, University of South Australia, Adelaide, South Australia, 5001, Australia

- Helen M. Stallman,
- Mark Kohler
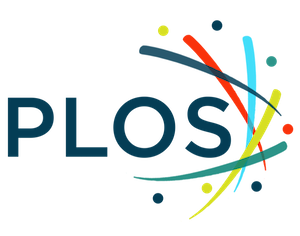
- Published: November 10, 2016
- https://doi.org/10.1371/journal.pone.0164769
- Reader Comments
Sleepwalking is thought to be a common arousal disorder; however, the epidemiology of this disorder has not yet been systematically examined. A systematic search of MEDLINE, CINAHL, EMBASE, PsycINFO, PubMed, and ScienceDirect was conducted for ‘sleepwalking’ OR ‘somnambulism’ in any field, to identify studies that reported the epidemiology of sleepwalking or sleepwalking disorders. Fifty-one studies assessed the prevalence rates of sleepwalking in a total sample of 100 490. The meta-analysis showed the estimated lifetime prevalence of sleepwalking was 6.9% (95% CI 4.6%–10.3%). The current prevalence rate of sleepwalking—within the last 12 months—was significantly higher in children 5.0% (95% CI 3.8%–6.5%) than adults 1.5% (95% CI 1.0%–2.3%). There was no evidence of developmental trends in sleepwalking across childhood. The significant risk of bias across all studies suggests these results should be used cautiously. Further epidemiological research that addresses methodological problems found in studies of sleepwalking to date is needed.
Citation: Stallman HM, Kohler M (2016) Prevalence of Sleepwalking: A Systematic Review and Meta-Analysis. PLoS ONE 11(11): e0164769. https://doi.org/10.1371/journal.pone.0164769
Editor: Ana Paula Arez, Universidade Nova de Lisboa Instituto de Higiene e Medicina Tropical, PORTUGAL
Received: May 31, 2016; Accepted: September 30, 2016; Published: November 10, 2016
Copyright: © 2016 Stallman, Kohler. This is an open access article distributed under the terms of the Creative Commons Attribution License , which permits unrestricted use, distribution, and reproduction in any medium, provided the original author and source are credited.
Data Availability: As this is a systematic review, all data is listed in the publication.
Funding: The authors received no specific funding for this work.
Competing interests: The authors have declared that no competing interests exist.
Introduction
Sleepwalking is a behavior characterized by partial arousal during slow wave sleep (N4) [ 1 ]. The potential adverse health outcomes of sleepwalking are injury to the sleepwalker themselves or to others as a result of impaired perception, characteristic of sleepwalking. The most sensationalized of these adverse events come to the public’s attention (e.g.[ 2 ]), otherwise sleepwalking largely goes unnoticed and may not get routinely reported to any health service. An absence of sleepwalking being recorded as a cause of significant injury requiring hospitalization or death (e.g.[ 3 , 4 – 6 ]) may be: 1) indicative of very low prevalence rates of sleepwalking; 2) a reflection of the low rates of adverse events from sleepwalking; and/or 3) represent inadequate identification, reporting, or assessment of sleepwalking as the cause of injuries. Understanding the epidemiology of sleepwalking is important to public health, individual decision-making and clinical management. It can inform optimal allocation of health resources for this largely neglected behavior. General population screening is needed to understand the potential health implications [ 7 ].
Challenges in epidemiological research for sleepwalking
The definition of sleepwalking varies considerably within the literature. The behavioral event is similar to the proverbial tree falling in the forest—if it is not observed, did it make a noise? Studies of children frequently rely on observation, typically using parent-report that their child sleepwalks, as the operationalization of sleepwalking. This reduces prevalence rates to those where the child captures the parents attention (e.g. such as by leaving their bedroom), are observed by parents, and the episode is later recalled by the parent. Some studies with older children use self-report, as do studies with adults. These are used to obtain lifetime and point prevalence rates, despite amnesia for the event being a common feature of the behavior. The classification of sleepwalking as a disorder rather than just a behavior, requires recurrent episodes, contact with others during the event, and amnesia for the event [ 8 ]. The American Psychiatric Association classifies sleepwalking as a mental illness if, in addition to the ICD-10 CM [ 8 ] characteristics, the events cause clinically significant distress or impairment in social, occupational or other important areas of functioning [ 9 ]. The increasing complexity of the definitions would be expected to result in decreasing prevalence rates, with sleepwalking behavior being the more prevalent and the mental illness of sleepwalking least likely to occur.
These differing levels of operationalizing sleepwalking necessarily result in different measurement strategies. Polysomnography (PSG) is the only measure that can accurately confirm the neurological event of sleepwalking—demonstrated by ambulant behavior during a maintained sleep state. However, PSG can be impractical to do on a large scale and may miss sleepwalking episodes that are usually infrequent. Fallible measures of sleepwalking include actigraphy, video monitoring, direct observation, self-report, and significant other report. Actigraphy is sensitive in detecting unique sleep patterns associated with specific sleep disorders [ 10 ]. It can provide an objective measure of sleep fragmentation due to movement, as a proxy measure of nocturnal wandering. Immediate parent-report relies on the child being observable to parents. Self-report relies on at least partial awareness of the event by the individual, or being told about their sleepwalking by someone who has observed it. Given that amnesia is a common feature of sleepwalking, sleepwalkers who are observed (e.g. children) would be more likely to be aware of sleepwalking than those who live alone. This most likely explains higher rates of sleepwalking in adults who are married compared with those who are single [ 11 ].
Retrospective recall is reliant on encoding the event as significant and long-term recall of the episode [ 12 ]. Distinctively different sleepwalking experiences would be more likely to be remembered by both sleepwalkers and their family members [ 13 ]. The distinctiveness of the episode constrains processing at the time of recall and thus reduces the incidence of false recall [ 14 ]. The measurement of the incidence of sleepwalking is likely to be more accurate than period prevalence, as a new experience of sleepwalking would be more distinctive to both parents/significant others and sleepwalkers than historical occurrences. Self-report could be based on memory for the event, distinctive features of an event such as injury or waking somewhere unusual, or reliant on what others have told the sleepwalker—each contains inherent measurement error. There is also the potential that individuals and observers could incorrectly classify all nocturnal wandering as sleepwalking.
Partially validated datasets are recommended in cases where the outcome variable is difficult or costly to measure [ 15 ], as in the case of sleepwalking. This involves all data points being classified by fallible tests and some of the data points being validated by also being classified by an accurate gold-standard test. To accurately assess the prevalence of sleepwalking, this would involve all participants being classified by fallible tests (such as self-, and parent-reports, actigraphy, or video monitoring) and some data points being validated by polysomnography. A partially validated dataset enables the systematic error that is included in each fallible measure to be quantified and taken into account in determining the true prevalence of sleepwalking.
Prevalence rates of sleepwalking frequented quoted in the literature typically relate to a single study (e.g. [ 16 ] or provide no reference at all e.g. [ 17 , 18 ]). The operationalization of sleepwalking is rarely mentioned. By combining studies, taking into account the differing conceptualization and measurement of sleepwalking and assigning the individual studies different weights according to their sample size, the potentially troublesome role of individual studies is minimized. The aim of this study is to systematically examine the epidemiology of sleepwalking in general population samples of children and adults.
This study was registered with PROSPERO (#CRD42016036296). PRISMA guidelines were followed in conducting and reporting the results of this systematic review and meta-analysis [ 19 ].
Search strategy
The following databases were included in the identification of relevant studies: MEDLINE, CINAHL, EMBASE, PsycINFO, PubMed, and ScienceDirect. Search terms were ‘sleepwalking’ OR ‘somnambulism’ in any field (e.g. PubMed search terms (sleepwalk*) OR somnambulism). The combined lists were screened for relevant titles and abstracts and full texts of all potentially relevant titles were examined. Studies were included if: 1) they reported the prevalence or incidence of sleepwalking; and 2) they were submitted to a peer-reviewed publication. All ages were included. The search was conducted in English; however, studies identified in other languages were included. Studies were excluded if: a) sleepwalking incidence or prevalence was not reported separately from other sleep disorders; b) participants were forensic cases or sleep-clinic samples; c) the study included drug-induced sleepwalking; or d) adults participants were psychiatric patients. Adult psychiatric patients were excluded because sleepwalking has been identified as a potential side-effect of psychotropic medications [ 20 ]. We identified other pertinent studies through citation tracking, review of reference lists in retrieved articles, Google Scholar, and our knowledge of the literature. The searches were from the beginning of each database through to 15 March 2016. All initial searches were conducted by the first author. The articles were then independently examined by the second author—there were no disagreements between authors.
Data extraction
Data were extracted independently by both authors. For each paper, we documented authors, year, country, study design (e.g. cross-sectional, longitudinal), setting (e.g. school, general population), participants (e.g. adults, children), sample size, response rate, age range, data collection procedure, sleepwalking measure, and results.
Data evaluation
We applied published guidelines for evaluating prevalence studies [ 21 ], using eight critical appraisal criteria across three domains, sampling, measurement, and data analysis. These are consistent with STROBE guidelines for the reporting observational studies in epidemiology [ 22 ]. Sampling items assessed whether the survey design yielded a sample of respondents’ representative of a defined target population. The items were whether: 1) the target population was clearly defined; 2) probability sampling was used to identify potential respondents; and 3) the characteristics of the respondents matched the target population. Measurement items assessed whether survey instruments yielded reliable and valid measures of sleepwalking. The items were whether: 4) the data collection methods were standardized; 5) the instruments were reliable; and 6) the instruments were valid. Data analysis assessed whether special features of the sampling design were accounted for in the analysis (Criterion 7). Confidence intervals, essential to produce frequency estimates within the population overall, were calculated for each study (Criterion 8). Evaluations was conducted independently by each author.
The prevalence of sleepwalking was calculated for each study with the number of reported sleepwalkers in the sample as the numerator and the total sample size as the denominator. All rates were calculated as the rate of sleepwalkers per 100 people, with the total sample being the summation of sleepwalker and non-sleepwalkers. An aggregate effect size, weighted by sample size, was computed to provide an overall effect size across the studies to identify the lifetime and current prevalence rates in children and adults. A random-effects model was used to aggregate individual effect sizes to create a pooled prevalence of sleepwalking. Random-effects models are based on the assumption that the true effect could vary between studies [ 23 ].
Homogeneity across studies was tested with the I 2 index, which provides the percentage of variation in prevalence attributable to between-study heterogeneity. An I 2 value of >75% is interpreted as high heterogeneity [ 24 ]. Post-hoc sensitivity analyses were conducted for the different study populations (child and adult) and measures (sleepwalking behavior and sleepwalking diagnoses) to investigate possible sources of heterogeneity. A forest plot was created to illustrate the prevalence of each study or current and lifetime sleepwalking, with 95% confidence intervals that contributed to the analysis along with the pooled prevalence estimate. Meta-regression was used to identify any developmental trends in current sleepwalking rates across childhood. Funnel plots [ 25 ] and Egger’s test of asymmetry [ 26 ] were used to formally detect bias within the results. All analyses were performed with Comprehensive Meta-Analysis Version 3 [ 27 ].
Description of studies
A total of 801 hits were produced in the database search. Review of titles resulted in 96 potentially relevant papers that were reviewed. Fig 1 displays the flow of information through the different phases of the systematic review and meta-analysis. From the original 64 sourced papers, 56 papers describing 51 studies met the inclusion criteria and were subsequently included for review. The eight full-text papers that were excluded were for the following reasons: not an empirical study ( n = 1), not a general population sample ( n = 5), not measuring sleepwalking epidemiology ( n = 1), and not reporting sleepwalking separately from other sleep problems ( n = 1).
- PPT PowerPoint slide
- PNG larger image
- TIFF original image
https://doi.org/10.1371/journal.pone.0164769.g001
A summary of the participant characteristics from the included studies is shown in Tables 1 and 2 . They span more than seven decades of research and include 20 countries—representing an international and cross-cultural sample. There were 15 studies of adults comprising 31 108 participants and 36 pediatric samples comprising 69 382 children. Sample sizes ranged from 100 to 15 929 participants. All used an observational study design using questionnaires or interviews. The majority of studies measured sleepwalking behavior ( n = 43). Eight studies measured sleepwalking using a diagnosis—five using DSM-IV [ 28 ] and three ICSD [ 29 ]. Several studies reported assessing ICD [ 30 ] and DSM diagnoses; however, as DSM has an additional criterion compared with ICD, that is ‘episodes cause clinically significant distress or impairment’ ([ 9 ]; p. 399) and only one result is reported in all studies, it is assumed the result refers to DSM. With child samples, six used child self-report and 26 used parent-report of sleepwalking behavior. Where children were asked to complete questionnaires with their parents, the results are included as parent-report.
https://doi.org/10.1371/journal.pone.0164769.t001
https://doi.org/10.1371/journal.pone.0164769.t002
Risk of bias.
The risk of bias analyses are presented in Table 3 . There were no studies without risk of bias and all failed to control for bias across multiple criterion.
https://doi.org/10.1371/journal.pone.0164769.t003
Criterion 1—Target population clearly defined: The majority of studies defined their target population (n = 44), six had risk of bias and one was unclear. Criterion 2 -Probability sampling used to identify potential respondents: More than half of the studies used probability sampling (n = 30), 12 had clear risk of bias, and 9 had an unclear risk. Criterion 3—Representativeness of the sample: There was significant heterogeneity in regards to how representative the samples were. For studies that reported or provided data to calculate response rates (n = 44), they ranged from 4.96% to 99.95%. Twenty-three studies met the minimum criteria of 70% response rate to be considered representative of the population [ 21 ]. Overall, only 15 papers had no risk of bias for sampling, 17 had risk of bias and 19 had unclear risk.
Measurement.
Criterion 4—Standardized data collection procedures: More than half of the studies had no risk of bias related to data collection procedures ( n = 29), three had risk of bias, 19 had unclear risk. Criterion 5—Reliable measurement: No studies reported the reliability of the measure they used to assess sleepwalking. Criterion 6—Valid measurement: No measures of sleepwalking used in any study have been validated. Measurement of sleepwalking varied considerably between studies. All studies used interview or questionnaires to measure sleepwalking—no studies included objective measures. Questions ranged from a single question to diagnostic interviews. Studies used either self-reported behavior ( n = 15), or parent report of behavior ( n = 27), self-report to form a diagnosis ( n = 4), or parent-report diagnosis ( n = 4), One study used more than one assessment measure of sleepwalking, collecting both parent and child reports [ 31 ]. Three studies encouraged participants to consult with a parent or family member, but did not assess or report the extent that was done [ 11 , 32 , 33 ].
Criterion 7—Sampling design accounted for in the analysis: Thirteen studies has no risk of bias, 28 had risk of bias and 10 had unclear risk. Criterion 8—Confidence intervals for statistical estimates: The confidence intervals were calculated for each study and are summarized in Table 3 .
As a result of the significant risk of bias in all studies and the great variations between studies including definitions of sleepwalking, data collection, measuring and reporting of results, a series of a priori decisions were made with respect to combining data. All studies were included and no sensitivity analyses were able to be conducted. For lifetime prevalence, the highest rate reported in a study was used as the lifetime prevalence rate. As the true lifetime prevalence rate cannot decrease over time, differences at different time points in the one study are likely to reflect attrition and/or recall bias. Period prevalence rates varied considerably between studies—previous week, previous one month, 3 months, 6 months, 12 months, or period not specified. The small sample size for any given period and the significant risk of bias within studies make it problematic to ascertain the prevalence at each point. Consequently the studies that reported period prevalence rates between one week and 12 months were combined to provide an estimate of the current prevalence of sleepwalking, defined here as sleepwalking within the past year. Where studies reported multiple time points within the previous 12 months, the highest rate is taken to reflect the greatest proportion of participants sleepwalking within the previous 12 months. Where multiple papers for a single study reported the current prevalence rate at different ages, all that did not overlap in the age range were included in the calculation of current prevalence rate. When studies reported that parents and children completed interviews or questionnaires together, these were recorded as parent-reported prevalence rates.
Current prevalence of sleepwalking
Mixed effects analysis showed a significant difference between the current prevalence rates for adult and child samples, Q = 22.25, p < .001, indicating the need to consider these populations separately. Thirty-one studies reported rates for various periods to provide current prevalence rates for sleepwalking behavior in children. A mixed effects analysis showed no significant difference between studies using child self-report behavior, parent-report behavior, and sleepwalking diagnoses, Q = 1.49, p = .48, indicating that they could be analyzed together. The event rates and 95% confidence interval across ages are shown in Fig 2 . The combined effect for the current prevalence of sleepwalking during childhood was 5.0% (95% CI 3.8–6.5). There was high and significant heterogeneity between studies ( I 2 = 98%; Q value = 969.45, p < .001), indicating great variability in effect size estimates.
https://doi.org/10.1371/journal.pone.0164769.g002
A mixed effects analysis showed no significant difference between self-reported sleepwalking behavior and diagnoses in adult studies ( Q = 3.57, p = .06). The event rates and 95% confidence interval across ages are shown in Fig 3 . The combined effect for the current prevalence rate of sleepwalking in adults from nine studies was 1.5% (95% CI 1.0%–2.3%). There was high and significant heterogeneity between studies ( I 2 = 93%; Q value = 108.65, p < .001), indicating great variability in effect size estimates.
https://doi.org/10.1371/journal.pone.0164769.g003
Lifetime prevalence of sleepwalking
There was no significant difference for lifetime prevalence rates of sleepwalking between adults and children, Q = 1.65 p = .20, indicating that the 20 studies could be analyzed together. The event rates and 95% confidence interval across ages are shown in Fig 4 . The combined effect for lifetime prevalence of sleepwalking was 6.9% (95% CI 4.6%–10.3%). There was high and significant heterogeneity between studies ( I 2 = 98%; Q value = 1238.95, p < .001), indicating great variability in effect size estimates.
https://doi.org/10.1371/journal.pone.0164769.g004
Developmental trends in sleepwalking
Pediatric studies of sleepwalking in childhood included children aged between two and 18 years. The ages varied considerably between studies. The reporting of prevalence rates was diverse and included prevalence at a given age, prevalence within the sample’s age range and only reporting the mean age of the sample and a prevalence rate. In order to assess the developmental trend of sleepwalking across childhood, the mean age for each sample was calculated for each study. The event rates and 95% confidence interval across ages are shown in Fig 5 . Meta-regression showed no significant relationship between the mean age of children reported in studies and the current prevalence rate of sleepwalking ( Q value = 0.34, p = .56, R 2 = 0). There was high and significant heterogeneity between studies ( I 2 = 98%; Q value = 1943, p < .001) indicating great variability in effect size estimates.
https://doi.org/10.1371/journal.pone.0164769.g005
Bias and heterogeneity
Inspection of the funnel plots showed significant bias with positive skew for both current and lifetime prevalence rates. The Egger’s test was significant for bias for current child ( t (29) = -8.66, p < .001) and adult analyses ( t (7) = -4.36, p < .001) and lifetime analyses ( t (18) = -7.99, p < .001). The Classic fail-safe N shows no evidence of publication bias with 4247 missing lifetime studies needed to bring p -value to non-significance, 8 010 adult current and 8 707 child current studies.
This systematic review included more than 100 000 people from 51 studies to identify the prevalence rate of sleepwalking in adults and children. Sleepwalking has been reported in children as young as two years and throughout adulthood. The lifetime prevalence for sleepwalking was 6.9% (95% CI 4.6%–10.3%). This does not vary significantly between childhood and adulthood, suggesting that relatively few people start sleepwalking later in life. This is consistent with adult onset of sleepwalking being associated with medications [ 20 ] and neurodegenerative diseases (e.g.[ 34 ]). This finding highlights the importance for detailed clinical evaluations of patients presenting with sleepwalking for the first time in adulthood. The current rate of sleepwalking was higher in children than adults 5.0% (95% CI 3.8–6.5) than in adults 1.5% (95% CI 1.0%– 2.3%). This difference may be the result of methodological issues or the decrease in slow wave sleep evident between childhood and adulthood [ 35 ].
The strengths of this study are its large sample size, both for children and adults, and the large number of studies included in the analyses. The limitations relate to the methodological problems within studies that are reflected in the high risk of bias across all studies and the consequent high heterogeneity across all analyses. Sleepwalking research is hampered by the very nature of the phenomenon. It occurs at night while the individual is sleeping. No studies included in this review used objective measures of sleepwalking; all relying on self- or parent-reports of sleepwalking behavior. Sleepwalkers typically have poor memory of sleepwalking episodes, because like other NREM dreams, sleepwalking actions appear less bizarre and novel than REM dreams [ 36 ]. The identification of sleepwalking episodes is therefore heavily dependent on the behavior being observed by others or the sleepwalker suspecting it because of injuries or other occurrences, such as noticing that things in the house have been moved, when they awaken. This is supported by research that included relationship status, showing that sleepwalking is more prevalent in married people than single people [ 11 ]. It may also mean that the significant difference in current sleepwalking rates between children and adults is an artifact of not being observed, rather than a true effect. It is important that future research measure who participants live with and how they or the informant know the person has been sleepwalking, and then control for these factors when determining prevalence rates. When using parent-report in older children, it would be important to ascertain whether parents are awake and able to observe adolescents sleepwalking in order to better determine the validity of the measure.
Reliability and validity have not been evaluated for self-, or parent-report measures of sleepwalking. This likely accounts for the very high heterogeneity in the results of this study. Because the definition of sleepwalking varied considerably across studies, random effects modelling was used in this study as it does not assume one underlying true effect across measures. In contrast, clinical diagnostic measures, such as the DSM, have diagnostic criteria that include recurrent episodes, observed sleepwalking behavior with impaired functioning [ 9 ]. Prevalence rates would have therefore been expected to be lower than for sleepwalking behavior. However, this was not evident in the current study, likely due to the enormous heterogeneity in the sample. It is recommended that single behaviors, in addition to frequency or recurrent sleepwalking be assessed in future epidemiological studies to overcome this problem. The presence of impairment or distress is a consequence of sleepwalking behavior and should not be used to calculate prevalence rates. Few studies that assessed current period prevalence of sleepwalking, assessed incidence. This is an important component for future studies to include in order to be able to understand the developmental trajectory of sleepwalking.
There was also inconsistency across studies in recall periods. It would be sensible for future research to use the last 12 months to capture current sleepwalking, in addition to a recent period—such as previous two weeks—that is less affected by recall bias. Previous year period prevalence and incidence measurement would also readily enable the developmental trajectory of sleepwalking to be better observed. Other periods such as one, three and six months do not add anything of importance to our understanding of sleepwalking.
Prevalence rates of sleepwalking are challenging to measure due to methodological limitations in identifying and accurately measuring the behavior. These include: a) the event happening during sleep when an individual is least likely to be witnessed; b) the individual having little or no memory for the event; c) reliance on recall of retrospective events by the sleepwalker or the informant; and d) differences in the conceptual understanding and measurement of the behavior. Any estimates, therefore, are likely to underestimate the true prevalence rates and represent episodes that are known to either caregivers (in relation to child studies) or the participant (in the case of adult studies). The summary prevalence rates reported here have significant limitations associated with systematic measurement error and should be used cautiously. The combined child and adult data supports the notion that sleepwalking behavior is a relatively common occurrence sometime during the lifespan. This review highlights the need for further epidemiological research to accurately explore the prevalence and incidence of sleepwalking across the lifespan. Outcomes would be strengthened by methodologies that: a) concurrently assess sleepwalking behavior and sleepwalking disorders; b) triangulate results using subjective and objective measures; c) assess how individuals know they sleepwalk; and d) assess injuries to sleepwalker and others.
Supporting Information
S1 table. prisma checklist..
https://doi.org/10.1371/journal.pone.0164769.s001
Author Contributions
- Conceptualization: HS MK.
- Data curation: HS.
- Formal analysis: HS.
- Investigation: HS MK.
- Methodology: HS MK.
- Project administration: HS.
- Supervision: HS.
- Validation: MK.
- Visualization: HS.
- Writing – original draft: HS.
- Writing – review & editing: HS MK.
- View Article
- PubMed/NCBI
- Google Scholar
- 2. Xie Q. Naked sleepwalker found in Manchester city centre at 4am by police—who returned him to his hotel after posing for a selfie. Daily Mail Australia. 2016;Monday, May 30th 2016.
- 3. Australian Bureau of Statistics. 3303.0—Causes of Death, Australia, 2013, Table 1. Underlying cause of death, All causes, Australia, 2013, ata cube: Excel spreadsheet, viewed 7 September 2015, http://www.abs.gov.au/AUSSTATS/[email protected]/DetailsPage/3303.02013?OpenDocument . 2015.
- 4. Australian Bureau of Statistics. 3303.0—Causes of Death, Australia, 2010, Table 1. Underlying cause of death, All causes, Australia, 2010, ata cube: Excel spreadsheet, viewed 7 September 2015, http://www.abs.gov.au/AUSSTATS/[email protected]/DetailsPage/3303.02010?OpenDocument . 2012.
- 5. Australian Bureau of Statistics. 3303.0—Causes of Death, Australia, 2012, Table 1. Underlying cause of death, All causes, Australia, 2012, ata cube: Excel spreadsheet, viewed 7 September 2015, http://www.abs.gov.au/AUSSTATS/[email protected]/DetailsPage/3303.02012?OpenDocument . 2014.
- 6. Australian Bureau of Statistics. 3303.0—Causes of Death, Australia, 2011, Table 1. Underlying cause of death, All causes, Australia, 2011, ata cube: Excel spreadsheet, viewed 7 September 2015, http://www.abs.gov.au/AUSSTATS/[email protected]/DetailsPage/3303.02011?OpenDocument . 2013.
- 7. Woodward M. Epidemiology: study design and data analysis. 3rd ed. Boca Raton: Taylor & Francis; 2014.
- 8. American Academy of Sleep Medicine. International classification of sleep disorders–Third Edition (ICSD-3) 3rd ed. Westchester, IL: AASM; 2014.
- 9. American Psychiatric Association. Diagnostic and Statistical Manual of Mental Disorders, Fifth Edition. Washington, D.C.: American Psychiatric Publishing; 2013.
- 12. Baddeley A, Eysenck MW, Anderson MC. Memory. 2nd ed. London: Psychology Press; 2015.
- 13. Eysenck MW. Depth, elaboration, and distinctiveness. In: Cermack LS, Craik FIM, editors. Levels of processing in human memory. Hillsdale, NJ: Lawrence Erlbaum Associates; 1979.
- 17. Wilson S, Nutt D. Sleep Disorders. Oxford: Oxford University Press; 2008.
- 18. Sheldon SH. Disorders of development and maturation of sleep, and sleep disorders in infancy, childhood, and cerebral palsy. In: Culebras A, editor. Sleep Disorders and Neurological Disease. Hoboken: Informa Healthcare; 2007. p. 59–82.
- 23. Borenstein M, Hedges LV, Higgins JPT, Rothstein HR. Introduction to meta-analysis. Chichester, UK: John Wiley & Sons Ltd; 2009.
- 25. Light RJ, Pillemer DB. Summing up: The Science of Reviewing Research. Cambridge, Massachusetts: Harvard University Press; 1984.
- 27. Borenstein M, Hedges L, Higgins JPT, Rothstein HR. Comprehensive meta-analysis version 3. Englewood NJ: Biostat, Inc; 2005.
- 28. American Psychiatric Association. Diagnostic and statistical manual of mental disorders (4th ed, TR). Washington, DC: Author; 2000.
- 29. American Academy of Sleep Medicine. The International Classification of Sleep Disorders, Second Edition (ICSD-2). Darien, IL: Author; 2005.
- 30. World Health Organization. The ICD-10 classification of mental and behavioural disorders: Clinical descriptions and diagnostic guidelines. Version 2016. Geneva: World Health Organization; 1992.
- 56. Klackenberg G. Incidence of parasomnias in children in a general population. In: Guilleminault C, editor. Sleep and its disorders in children. New York: Raven Press; 1987. p. 99–113.
- 69. Anders TF, Eiben L. Sleep Disorders. In: Zeanah C, editor. Handbook of infant mental health. New York: The Guilford Press; 2000.

An official website of the United States government
The .gov means it’s official. Federal government websites often end in .gov or .mil. Before sharing sensitive information, make sure you’re on a federal government site.
The site is secure. The https:// ensures that you are connecting to the official website and that any information you provide is encrypted and transmitted securely.
- Publications
- Account settings
Preview improvements coming to the PMC website in October 2024. Learn More or Try it out now .
- Advanced Search
- Journal List
- J Clin Sleep Med

Differentiating Parasomnias from Nocturnal Seizures
Lana jeradeh boursoulian.
1 Department of Neurology, Vanderbilt University, Nashville TN
Carlos H. Schenck
2 Minnesota Regional Sleep Disorders Center, Minneapolis, MN
3 Department of Psychiatry
Mark W. Mahowald
4 Department of Neurology University of Minnesota Medical School, Minneapolis, MN
Andre H. Lagrange
Case presentation.
Patient with nocturnal events; referral diagnosis is “atypical night terrors.”
History of Present Illness
A 32-year-old woman presented to a sleep disorders center with a history of spells arising from sleep. The spells occurred on a nightly basis and were described as screaming with bilateral thrashing and flailing movements of the arms and legs. They frequently caused injury both to herself and her husband, who sleeps with her. Eyes can be open or closed. These events were also associated with loud screaming. There was no confusion or neurological deficit immediately afterward, and she was later able to remember some of the events. The spells usually lasted from 15 seconds to 1 minute, occurring 2-4 times a night, beginning soon after falling asleep. The patient was unable to recall any specific precipitators. She has tried multiple medications including carbamazepine, primidone, valproic acid, zonisamide, and clonazepam. Following placement of a vagal nerve stimulator, she became convinced that none of the treatments helped, and she stopped all medications on her own, with no change in the frequency of her spells.
Sleep History
The patient had a regular bedtime and wakeup time; she denied snoring. She did, however, complain of excessive daytime sleepiness, with an Epworth Sleepiness Scale score of 11, but denied symptoms of cataplexy, hypnagogic or hypnopompic hallucinations, or sleep paralysis. She had a few episodes of somnambulism as an adult, including one episode in which she defecated in the living room. There was no childhood history of sleepwalking, sleep terrors, or other parasomnia.
Past Medical History
The patient had a history of seizures as a child. However, those events were semiologically very different from her current spells. They began with burning paresthesias in the left face, a sensation of falling, and brief left face and arm movements with loss of awareness. The seizures eventually ceased with anti-epileptic drug therapy. The currently reported nocturnal spells with 4-extremity movements began some time after a sexual assault and a prolonged abusive relationship (which ended several years before she was married). She also has a history of depression with suicidal ideation but has never sought therapy.
Family History
There was no family history of seizures or of parasomnias.
Physical Examination
Patient presented in no acute distress, and had a blunted and at times negative affect. Tonsils are not enlarged, nares were patent. BMI was 28.1. Friedman palate position was 2 (allows visualization of the uvula and soft palate but not the tonsils). Respiratory, cardiac, and neurological examinations were all normal.
The patient had a 3-minute sleep onset latency. REM onset latency was moderately prolonged. REM sleep had normal muscular atonia, without excessive twitching or behaviors. Mild snoring was observed, but her respiratory disturbance index was 0.6 events per hour, with a minimum oxygen saturation level of 94%. The patient had 6 spells arising from NREM sleep (usually N2 sleep) that appeared stereotypical and were characterized by violent right leg kicking, right arm extension, generalized thrashing, and prolonged screaming lasting less than a minute, followed by rapid orientation and appropriate interaction with the sleep technologist in which some awareness of the episode was described, along with apologetic comments about “doing it again.” The final spell occurred with the termination of sleep. There was no electrical seizure activity, although movement artifact often obscured the EEG during these spells.
- Brain MRI and routine EEG: read as normal
A total of 43 events were recorded (average of 8 events per night). Each event lasted for about a minute and a half on average. Exemplar EEG tracings are shown in Figures 1 and 2 2 . Pictures from the events are shown in Figure 3 .

EEG recording during one of the typical events

EEG recording at the beginning of another typical event
At the clinical onset there is the appearance of multiple EEG artifacts, including muscle activity, movement artifacts, and a single electrode “pop” at Fp2 that creates a very large, extremely brief potential at the middle of the figure.

Sequence pictures of the patient during an event.
There was a slow, large amplitude circular movement at the right shoulder and hip. Tonic leg extension on the left is obscured by the blanket.
QUESTION 1: What do you see on the EEG on Figure 1 ?
QUESTION 2: Was the patient awake or asleep at the beginning of the event in Figure 2 ?
QUESTION 3: What is the diagnosis?
ANSWER 1: No EEG changes are seen during event except for muscle artifact and movement artifact (note that the artifact signal is also seen on EKG signal). A similar event previously recorded at an outside institution was interpreted as being nonepileptic, probably psychogenic.
ANSWER 2: Patient was in stage N2 sleep when the event started, as evidenced by the presence of sleep spindles. If you look carefully at Figure 4 , you should see transitional sharp wave preceding the event. 1 This is followed by a subtle faster rhythm seen in the midline frontocentral channels, most prominently on the right. This activity was only apparent on a small portion of her events, due to muscle artifact.

Black arrows demonstrate high amplitude sharp waves with a phase reversals at C4/P4 followed by subtle rhythmic activity (white arrow) in the right frontocentral region that evolves in frequency and amplitude, before becoming obscured by muscle artifact on subsequent pages
Sharp waves are defined as sharply contoured electrical activity of < 200 ms duration and asymmetric rates of rise and fall. In comparison, the large amplitude activity immediately preceding the second sharp wave is slow, rounded, and has relatively symmetric positive (up) and negative (down) phases, and therefore represents normal interictal sleep activity.
ANSWER 3: Nocturnal frontal lobe epilepsy (NFLE)
We present a challenging case of a 32-year-old woman with nocturnal spells who presents with some of the classic features for frontal seizures, but with enough confounding factors to allow a very broad differential diagnosis. There are several clues from the history that suggest the epileptic nature of the events: the brief duration, the stereotypical presentation, and the history of childhood seizures. Moreover, frontal lobe seizures are known to occur predominantly during sleep. 2 These seizures may be associated with bizarre hypermotor activity without a clear loss of consciousness and may be unwitnessed by a groggy bed partner.
The differential diagnosis of paroxysmal events during sleep also includes:
- Parasomnias
- Parasomnias associated with REM sleep (REM sleep behavior disorder [RBD])
- Sleep related movement disorders
- Psychogenic nonepileptic seizures
- Sleep related dissociative disorders
NREM arousal disorders are common in childhood, can persist into adulthood, and at times can even begin in adulthood. 3 The duration and complexity of those events can vary from brief and simple confusional arousals to more prolonged and complex somnambulism. Sleep terrors are usually distinguished by their strong associated autonomic features, including tachycardia and diaphoresis, and lack of recall the next day. Loud and prolonged screaming can occur, along with violent thrashing and bolting from bed in a terrified state. However, it is very rare, if not unprecedented, for sleep terrors to emerge multiple times nightly during virtually every night for a period of months or years.
Differentiating between nocturnal seizures and NREM parasomnias can be challenging, especially in regards to nocturnal frontal lobe epilepsy (NLFE) because of the typically unusual, bizarre presentation of NLFE seizures, along with its common association with a normal EEG. Features supporting an epileptic etiology of paroxysmal events are: (1) stereotyped nature of the spells; (2) high frequency and tendency to cluster 4 ; (3) timing of the events (NREM parasomnias usually emerge from slow wave sleep, which typically occurs within 2 h of sleep onset, 5 whereas frontal lobe seizures may occur during any sleep stage, but are common shortly after falling asleep); (4) semiology of events (although frontal lobe seizures may have variable manifestations, the occurrence of prominent unilateral tonic stiffening favors an epileptic origin rather than a parasomnia); (5) duration of events (parasomnias are usually relatively prolonged events, whereas epileptic seizures, especially frontal lobe seizures, tend to be very brief, lasting < 2 min on average) 4 ; and (6) presenting age (NREM parasomnias are often limited to childhood, but can occur in adulthood, whereas NFLE often persists into adulthood). In retrospect, the childhood seizures may well have been from the same focus, although it is unclear why the semiology changed with age. Finally, the reported episodes of “somnambulism” during adulthood in this patient, including one episode with defecation, suggests “episodic nocturnal wandering” of epileptic origin more than bona fide somnambulism as a parasomnia. 6
RBD can be ruled out because there was preservation of the normal REM sleep atonia on polysomnography 6 ; also RBD typically affects middle-aged or older males, although females of any age can develop medication-induced RBD. Drug-induced RBD is typically caused by SSRI and most other antidepressants (apart from bupropion or trazadone), which does not apply to this patient who never received an antidepressant. In addition, RBD would likely occur toward the end of the sleep cycle, which has not been seen in our patient.
There are several sleep related movement disorders according to the International Classification of Sleep Disorders (ICSD-2), 7 including restless legs syndrome, sleep related leg cramps, periodic limb movement disorder, sleep related bruxism, and sleep related rhythmic movement disorders. However, the specific historical features for these conditions were absent, along with the lack of complexity and clustering pattern that was evident in our patient.
Although many seizures are easily distinguished from nonepileptic events on the basis of the history, this can be difficult in the case of NFLE. In our patient, the lack of a clear EEG correlate, the dramatic/complex movements, and her psychiatric/social history contributed to an earlier diagnosis of psychogenic events. However, it is important to note that EEG can frequently be normal in NFLE for multiple reasons, including deep generators, few electrodes, and poor field coverage with the international 10-20 system. In addition, the EEG can be frequently obscured by muscle artifact. Moreover, even when epileptiform activity is recorded, it may difficult to correctly lateralize the onset, especially from a parasagital focus in which the near-midline ictal focus can create an electrical dipole directed toward the contralateral electrodes. There are several semiological features that can be seen in both NFLE and psychogenic nonepileptic seizures: eye closure, hypermotor activity, and partial recall during the events.
On the other hand, while the fact that the events arose out of sleep also support the diagnosis of seizures, it is quite common for patients with psychogenic nonepileptic seizures to describe the events as coming out of sleep; however, these usually arise from “pseudo-sleep,” whereby the patient awakens shortly before the event. Sleep related dissociative disorders typically affect females subjected to physical and/or sexual abuse, which applies to this patient. However, episodes occur during EEG wakefulness after sustained arousals from sleep, and the behaviors are not stereotypical. 3
With more prolonged monitoring of this patient at our institution, we were able to confirm the stereotypical nature of the events on video, record several seizures in which the episodes progressed to left eye deviation and rhythmic left face twitching, and then a single episode with onset of fast activity in the right posterior quadrant that then evolved prior to clinical onset.
In diagnostically difficult cases, more prolonged monitoring can be very helpful for two reasons. First, by recording multiple events, the reviewing physician may begin to appreciate subtle but consistent findings in either the seizure semiology or EEG changes. Second, recording multiple events increases the odds of either capturing an event without motion artifact or an event in which electrographic activity builds to the point of being recordable on the scalp.
Diagnostic alternative approaches: when the anatomic imaging is normal, the addition of functional imaging can be very helpful. Ictal single-photon emission computed tomography (SPECT) can be useful to detect subtle areas of increased blood flow, especially when using subtraction ictal SPECT co-registered to MRI (SISCOM) analysis. 8 Newer technologies, including magnetoencephalography, function resting state, and diffusion tensor imaging, are being explored as alternative approaches to define a seizure focus when clinical suspicion of epilepsy is high. 9
While her MRI was initially read as normal, there was indeed a slight asymmetry in the cortical gyri in the right paramedian posterior area. While this was not definitely abnormal, ictal SPECT with SISCOM analysis showed very clear increased perfusion during a seizure ( Figure 5A ). She subsequently underwent intracranial recording, which confirmed ictal onset in this region. Following resection of this small area ( Figure 5B ) she became seizure free and had a much better mood and energy level during the day. Her family commented on the disappearance of her longstanding blunted affect. She initially had some left foot weakness that resolved to the point that she was able to take up running. She has returned to work and recently celebrated the birth of her first child.

SISCOM analysis of her preoperative ictal SPECT

Postoperative MRI FLAIR sequence images, white arrows refer to the area of resection
DISCLOSURE STATEMENT
This was not an industry supported study. The author has indicated no financial conflicts of interest.
Boursoulian LJ; Schenck CH; Mahowald MW; Lagrange AH. Differentiating parasomnias from nocturnal seizures. J Clin Sleep Med 2012;8(1):108-112.
Disability and Safety: Information on Wandering (Elopement)
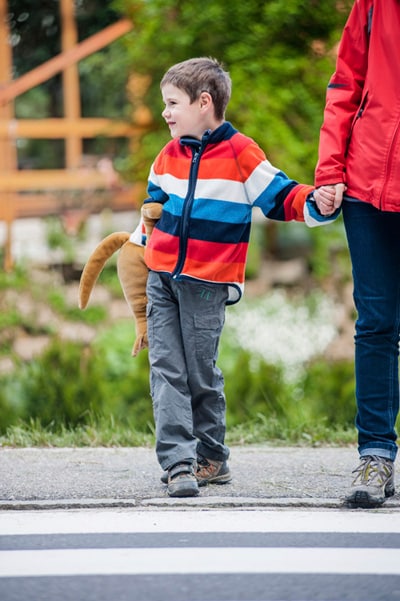
Wandering, also called elopement, is an important safety issue that affects some people with disabilities, their families, and the community. There are steps that parents, teachers, healthcare providers, and others can take to help keep children safe.
Wandering is when someone leaves a safe area or a responsible caregiver. This typically includes situations where the person may be injured or harmed as a result. 1 Wandering goes beyond the brief time that a typical toddler might run off from a caregiver. Some children and youth with disabilities, such as those with autism spectrum disorder (ASD) or intellectual disability (ID), have challenges understanding safety issues and communicating with others. For example, such a child might run off from home to play in the pond down the street- and be unable to respond to his name or say where he lives. This can happen quickly, even under constant supervision. The child’s parents are left searching desperately for him or her.
Based on a survey of parents external icon , about half of children and youth with ASD were reported to wander. Of those children, 1 in 4 were missing long enough to cause concern and were most commonly in danger of drowning or traffic injury. Children wandered most often from their own home or another home, stores, and classrooms or schools. The primary reasons for wandering included:
- Enjoyment of running or exploring
- To get to a place he or she enjoys (like a pond)
- To get out of a situation that causes stress (for example, being asked to do something at school or getting away from a loud noise)
- To go see something interesting (for example, running to the road to see a road sign)
Although many examples of dangerous wandering have focused on children with ASD, we know that challenges with communication, social interaction, attention, and learning can put many children and youth with developmental disabilities at risk for becoming lost or injured due to wandering.
What Can We Do to Keep Children Safe Who Might Wander?
Parents, teachers, and other caregivers.
- Watch the child’s behaviors
- Have an emergency plan to respond
- Keep information about the child up-to-date (picture, description)
- Secure your home (fences, door locks)
- Keep identification on the child (ID bracelet or information card)
- Notice signs that the child may wander off before it happens (for example, child makes a certain sound or looks towards the door)
- Be alert about the child’s location
- Provide a safe location
- Inform neighbors and school workers
- Alert first responders
Teach Safety Skills
- Responding to safety commands (“stop”)
- Stating name and phone number (or showing ID)
- Swimming, crossing the street
First Responders
First responders are vital for maintaining the health and safety of members of our communities. They are likely to be called upon in the event of a missing child or youth. It is important for first responders to be prepared by knowing which children in the community might wander, having family contact information, and having a plan to respond. Tools and training materials are available through the AWAARE organization external icon and through the National Center for Missing and Exploited Children external icon .
Healthcare Providers and Other Professionals
Healthcare and other professionals need to be aware of wandering as a safety issue. Their role includes discussing safety issues and helping caregivers come up with prevention and response plans. Tools and information to help are listed in the “Resources” section below.
The ICD-10-CM code Z91.83 (wandering in diseases classified elsewhere) helps document wandering and to prompt important discussions about safety among caregivers, individuals, and providers. This code is not linked to a specific diagnosis, nor is it part of the diagnostic codes used for ASD or intellectual disabilities. Wandering should be coded if documented in the medical record by the provider (i.e., physician).
CDC’s Work
CDC works to keep people safe and healthy and this includes addressing the special needs that people with disabilities may have. CDC has partnered with others to understand how common wandering or elopement is among children with ASD and other developmental disabilities. CDC has served on the Interagency Autism Coordinating Committee (IACC) Safety Subcommittee, assisted in data collection on wandering, and worked with partners to raise awareness of wandering as an important safety issue.
The AAP provides information and resources for professionals and families on the identification and healthcare management of children and youth with an autism spectrum disorder (ASD).
AWAARE.org external icon This site has information and resources to prevent and respond to potentially dangerous wandering. This includes The Big Red Safety Toolkit for caregivers pdf icon [PDF – 6.03 MB] external icon and first responders pdf icon [PDF – 2.18 MB] external icon related to wandering.
iancommunity.org external icon The Interactive Autism (IAN) Network has surveyed caregivers about the occurrence of wandering among children with ASD and their siblings.
Autism Speaks’ Safety Project external icon This site includes information and tips on safety for people with ASD and their families.
Autism Society external icon The Autism Society of America has been improving the lives of all affected by autism for over 50 years and envisions a world where individuals and families living with autism are able to maximize their quality of life, are treated with the highest level of dignity, and live in a society in which their talents and skills are appreciated and valued.
The National Center for Missing and Exploited Children external icon This organization has put together tools and guides for first responder and search terms to help in training and response to missing children with special needs.
- Anderson C external icon , Law JK external icon , Daniels A external icon , Rice C external icon , Mandell DS external icon , Hagopian L external icon , Law PA external icon . Occurrence and family impact of elopement in children with autism spectrum disorders. external icon Pediatrics . external icon 2012 Nov;130(5):870-7. doi: 10.1542/peds.2012-0762. Epub 2012 Oct 8.
- Policy Makers
- CDC Employees and Reasonable Accommodations (RA)
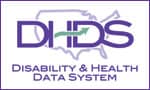
Exit Notification / Disclaimer Policy
- The Centers for Disease Control and Prevention (CDC) cannot attest to the accuracy of a non-federal website.
- Linking to a non-federal website does not constitute an endorsement by CDC or any of its employees of the sponsors or the information and products presented on the website.
- You will be subject to the destination website's privacy policy when you follow the link.
- CDC is not responsible for Section 508 compliance (accessibility) on other federal or private website.
REVIEW article
Potential pathways for circadian dysfunction and sundowning-related behavioral aggression in alzheimer’s disease and related dementias.
- Program in Neuroscience, Department of Zoology and Physiology, University of Wyoming, Laramie, WY, United States
Patients with Alzheimer’s disease (AD) and related dementias are commonly reported to exhibit aggressive behavior and other emotional behavioral disturbances, which create a tremendous caretaker burden. There has been an abundance of work highlighting the importance of circadian function on mood and emotional behavioral regulation, and recent evidence demonstrates that a specific hypothalamic pathway links the circadian system to neurons that modulate aggressive behavior, regulating the propensity for aggression across the day. Such shared circuitry may have important ramifications for clarifying the complex interactions underlying “sundowning syndrome,” a poorly understood (and even controversial) clinical phenomenon in AD and dementia patients that is characterized by agitation, aggression, and delirium during the late afternoon and early evening hours. The goal of this review is to highlight the potential output and input pathways of the circadian system that may underlie circadian dysfunction and behavioral aggression associated with sundowning syndrome, and to discuss possible ways these pathways might inform specific interventions for treatment. Moreover, the apparent bidirectional relationship between chronic disruptions of circadian and sleep-wake regulation and the pathology and symptoms of AD suggest that understanding the role of these circuits in such neurobehavioral pathologies could lead to better diagnostic or even preventive measures.
Introduction
Behavioral aggression and circadian dysfunction are both prevalent in several neural disorders ( Todd and Machado, 2019 ), including Alzheimer’s disease (AD) and related dementias, and there has been an abundance of work over the last decade highlighting the general importance of circadian function on the regulation of mood and emotional behavior, including aggression ( Bronsard and Bartolomei, 2013 ; Hood and Amir, 2018 ; Taylor and Hasler, 2018 ; Logan and McClung, 2019 ; Ketchesin et al., 2020 ). For example, circadian disruptions such as rotating shift work and jet lag due to transmeridian travel have been shown to precipitate or exacerbate mood symptoms ( Asaoka et al., 2013 ; Kalmbach et al., 2015 ; Inder et al., 2016 ). More specifically, social jet lag (defined as a discrepancy between the body’s internal circadian clock and the actual sleep schedule) has been associated with increased physical and verbal aggression ( Randler and Vollmer, 2013 ; Lin and Yi, 2015 ). Converging evidence also supports the notion that evening chronotypes exhibit a greater predisposition for behavioral aggression ( Schlarb et al., 2014 ; Deibel et al., 2020 ). Recent work in transgenic mice also suggests that the master circadian pacemaker, located within the suprachiasmatic nucleus (SCN) of the anterior hypothalamus, directly modulates a rhythm in the propensity for aggressive behavior via a polysynaptic pathway contained entirely in the hypothalamus ( Todd et al., 2018 ). Todd et al. (2018) showed that a functionally connected circuit from the SCN, through the nearby subparaventricular zone (SPZ), gates the activity of neurons within the ventromedial hypothalamus (VMH) that drive aggressive behavior (the SCN → SPZ → VMH pathway, see Figure 1 ). This pathway may be a substrate through which circadian dysfunction can lead to increased aggression, both acutely and chronically in disorders that are characterized by circadian disruption and high levels of aggression and agitation.
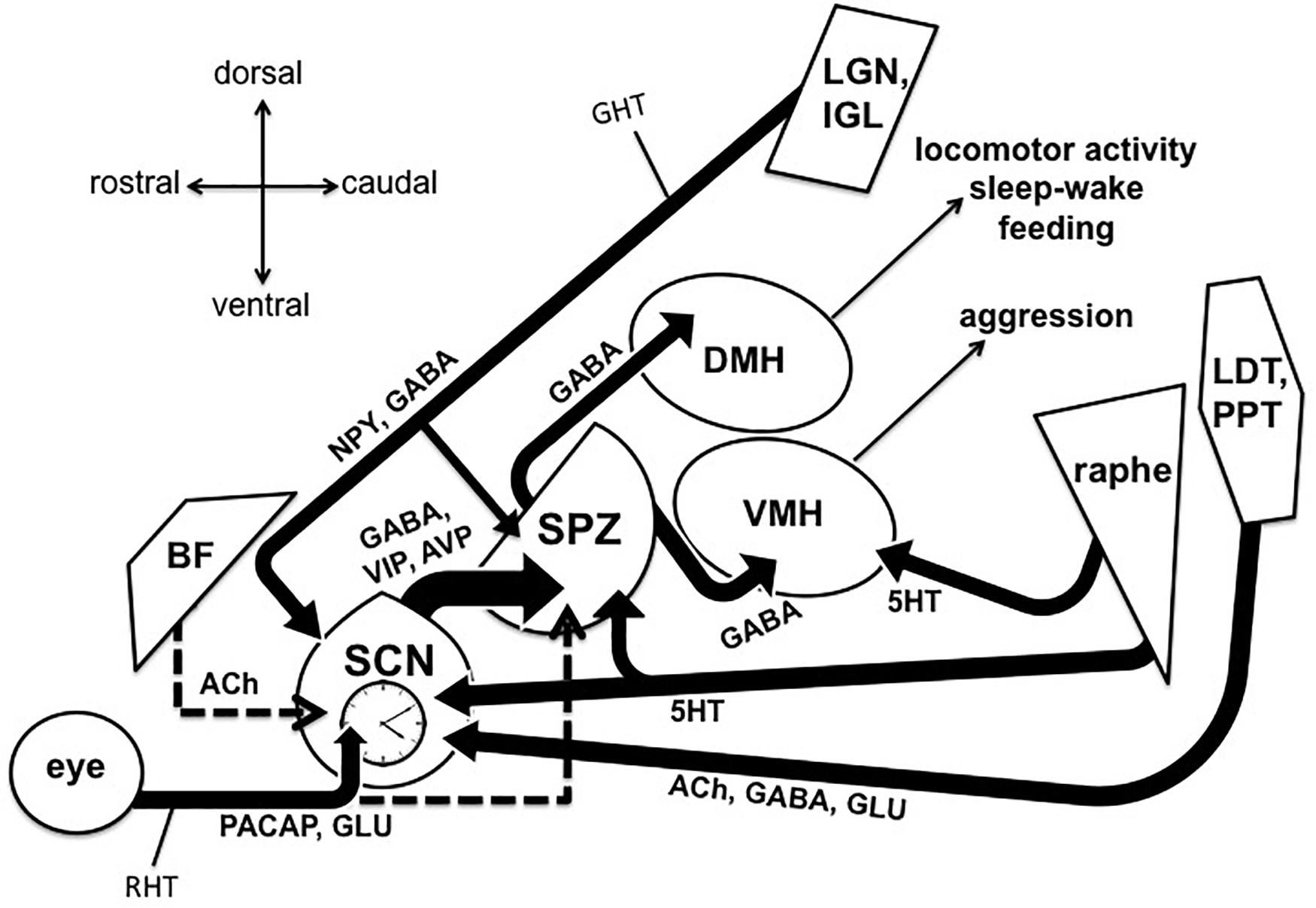
Figure 1. Output and input pathways of the central circadian timing system in the mammalian brain that may be involved sundowning–related behavioral aggression and circadian dysfunction in Alzheimer’s disease and related dementias. The master circadian pacemaker, is the suprachiasmatic nucleus (SCN) of the hypothalamus. The SCN releases the fast neurotransmitter GABA, as well as several peptides including vasoactive intestinal peptide (VIP) and argine vasopressin (AVP) from its major axonal output pathway to the nearby subparaventricular zone (SPZ). The GABAergic SPZ regulates rhythms of locomotor activity, sleep-wake, and feeding via pathway to the dorsomedial hypothalamus (DMH), and regulates rhythms of aggression propensity via a pathway to the ventromedial hypothalamus (VMH). The SCN is entrained to the daily light-dark cycle by input from intrinsically photosensitive retinal ganglion cells, which release pituitary adenylate cyclase activating polypeptide (PACAP) and glutamate (GLU) via the retinohypothalamic tract (RHT). The RHT also densely innervates the SPZ in most nocturnal mammals, but provides little or no innervation of the SPZ in many diurnal mammals, including humans (indicated by dashed line). A cholinergic (ACh) input to the SCN from the basal forebrain has been suggested in rats, but is absent in mice (indicated by dashed line). Cholinergic input to the SCN has also been reported from the laterodorsal tegmentum (LDT), pedunculopontine tegmentum (PPT) complex, which also releases GABA and GLU. Serotonergic (5HT) inputs to both the SCN and SPZ have been reported from the midbrain raphe complex. Finally, the geniculo-hypothalamic tract (GHT), originating from the retinoreceipient (not shown here) ventral lateral geniculate nucleus (LGN) and intergeniculate leaflet (IGL) of the thalamus, provides an input of GABA and neuropeptide Y (NPY) to both the SCN and SPZ. Structures are not drawn to scale.
Agitation and aggression, circadian dysfunction, and several other non-cognitive symptoms of AD and dementia seem to point to an underlying disruption in the hypothalamus ( Ishii and Iadecola, 2015 ; Hiller and Ishii, 2018 ), even though brainstem and cortical structures are normally the foci of most neuropathological investigations concerning these disorders. While circadian disruption of sleep-wake and other rhythms is a typical component of normal healthy aging, such dysfunction is greatly exacerbated in neurodegenerative disorders such as AD and dementia. Indeed, a growing body of evidence suggests a bidirectional interaction between the circadian system, AD pathology, and the progression of the disease ( Musiek, 2015 ; Videnovic and Zee, 2015 ; Musiek and Holtzman, 2016 ; Duncan, 2020 ). Since such neurodegenerative disorders clearly disrupt the circadian rhythmicity of sleep-wake, it is likely that they also disrupt the circadian regulation of emotional processing and aggression propensity as well. Indeed, the interaction between the circadian system and processes modulating aggression may be a key contributor to the clinical phenomenon known as “sundowning syndrome”, which is commonly reported in AD and dementia patients. Sundowning is characterized by increased confusion and emotional behavioral disruptions, such as agitation and aggression, particularly during the late afternoon and early evening hours ( Bachman and Rabins, 2006 ; Khachiyants et al., 2011 ; Bedrosian and Nelson, 2013 ; Canevelli et al., 2016 ). This syndrome can create a major burden on both patients and caretakers, with organizations such as the Alzheimer’s Association and the National Institute on Aging providing online caretaker resources to help them better cope with sundowning symptoms ( Aging, 2017 ; Association, 2020 ). Indeed, sundowning symptoms have been cited as among the most important factors leading to the decision to seek institutionalization ( Pollak and Perlick, 1991 ; Hope et al., 1998 ).
Sundowning was first described in the medical literature over 80 years ago as “senile nocturnal delirium” ( Cameron, 1941 ), when D. Ewen Cameron noted an exacerbation of delirium and agitation that occurred within an hour of placing dementia patients into a darkened room. The term “sundowning syndrome,” due to the phenomenon’s association with the onset of daily darkness, was first coined in the late 1980s by Lois K. Evans, who described it as a recurring condition among institutionalized older adults similar to delirium, but lasting much longer ( Evans, 1987 ). However, since that time, the relevant literature on sundowning has been relatively scarce, and the underlying pathophysiology of the syndrome remains enigmatic. Perhaps one of the primary reasons sundowning remains poorly understood is that the symptoms and criteria used to define it have differed widely across groups ( Bachman and Rabins, 2006 ; Canevelli et al., 2016 ). For instance, some groups have focused more on the emotional components of the syndrome, some more on the increased nocturnal locomotor activity such as wandering, whereas fewer have described sundowning as primarily a sleep-related disturbance ( Boronat et al., 2019 ). It is also important to note that sundowning is not an official diagnosis (it does not appear in the DSM-5), but rather a loose grouping of symptoms. These challenges probably contribute to the wide range of prevalence reported for sundowning across studies, with some studies reporting as high as 60%, while others reporting as low as 2.5% for dementia patients depending on the setting ( Khachiyants et al., 2011 ; Canevelli et al., 2016 ). However, more recent work suggests a more narrow prevalence between 20 and 27.8% ( Angulo Sevilla et al., 2018 ; Pyun et al., 2019 ).
Research done during the 1990s and 2000s led some to question whether sundowning represents an actual time-dependent worsening of behavioral disturbances, or instead an increase in caretakers’ perceptions of the stress caused by these disruptions at a particular time of day ( Gallagher-Thompson et al., 1992 ; Bliwise et al., 1993 ; Cohen-Mansfield, 2007 ). Additionally, some studies did not find support for an exacerbation of behavioral symptoms occurring specifically around sunset ( Bliwise et al., 1993 ; Friedman et al., 1997 ), with one suggesting that peak agitation actually occurs during the early afternoon ( Martin et al., 2000 ). However, in a later discussion of diagnostic criteria for sleep disorders in AD ( Yesavage et al., 2003 ), several of these same authors noted that “other research does support the notion that the nocturnal hours or the period of sunset (ranging from 4:00 to 8:00 PM depending on the study) are vulnerable to agitation,” and that “(t)aken together, these results lend support to the existence of a circadian rhythm for agitated behaviors in many AD patients that peaks late in the day, although its precise delineation in real time and its association with sunset, sleep, and patient and/or disease characteristics remain unclear.” Yesavage et al. (2003) further stressed the important point that “although there is mixed evidence for the existence of sundowning and it may be useful descriptively, the term, when used to define sleep disturbance, is too broad to be of practical diagnostic value.” Altogether, this raises the possibility that the sundowning phenomenon reflects a time-dependent disturbance in emotional regulation rather than a direct sleep disturbance. And, its occurrence may be more generally tied to a 4-h window within the late afternoon and early evening instead of being directly tied to sunset.
Indeed, during this same time, numerous more groups reported disturbances in AD and dementia patients that temporally and qualitatively match the traditional description of sundowning-related agitation and aggression ( Martino-Saltzman et al., 1991 ; Cohen-Mansfield et al., 1992 ; O’Leary et al., 1993 ; Burgio et al., 1994 ; Sloane et al., 1998 ). Even more recently, several observational studies defining sundowning as an increase in neuropsychiatric behaviors (including agitation and aggression) in the late afternoon and early evening have observed this phenomenon in AD patients in association with important circadian or AD-related factors ( Silva et al., 2017 ; Angulo Sevilla et al., 2018 ; Menegardo et al., 2019 ; Pyun et al., 2019 ; Shih et al., 2019 ). For instance, Menegardo et al. (2019) associated the aggressiveness and irritability of sundowning with increased nocturnal behavior such as wandering. Silva et al. (2017) also associated sundowning with increased depressive and cognitive symptoms, suggesting that multiple emotional systems are disrupted in this syndrome and that these become even more compromised as AD progresses with more associated cognitive decline. Angulo Sevilla et al. (2018) also noted an association of such sundowning symptoms with an increased severity of dementia, but also in association with insomnia and hypersomnia. Interestingly, Pyun et al. (2019) found a strong association between these sundowning symptons and the presence of the apolipoprotein E (APOE) ε4 allele, an important genetic risk factor in the development of late-onset AD that promotes amyloid pathology ( Corder et al., 1993 ).
A recent scoping review across 23 studies focused on sundowning found that temporal periodicity was the most prevalent finding, with 90.0% of the studies that met their criteria for inclusion reporting an onset of behavioral disturbances occurring during the middle afternoon and early night ( Boronat et al., 2019 ). The symptoms examined across these studies most commonly clustered into “psychomotor disturbances” at 83.3%, and included agitation, aggression, and restlessness, followed by a cluster of symptoms categorized as “cognitive disturbances” at 66.7% including confusion, disorientation, and wandering. Importantly, these studies also largely support the notion that sundowning may reflect a time-dependent disturbance in emotional behaviors, rather than a sleep disturbance per se . Therefore, in order to better understand and treat sundowning symptoms, it is important to recognize the interacting neural components that modulate the production and daily timing of emotional behavioral states. Interestingly, Todd et al. (2018) found that disrupting the SCN → SPZ → VMH pathway led to increased behavioral aggression specifically during the early resting phase (the light phase for nocturnal mice), a time which appears to be temporally analogous to when AD and dementia patients have traditionally been reported to display sundowning symptoms ( Todd et al., 2018 ). Such shared neural pathways may be promising targets for treatments that could greatly reduce sundowning and other symptoms associated with circadian dysfunction. This review examines the existing literature on specific pathways emanating from the circadian system and the behaviors they regulate, in addition to pathways that provide input to the circadian system and influence its function ( Figure 1 ). It also focuses on the evidence concerning whether AD-related disruption of these circuits might underlie sundowning symptoms, as well as how these pathways might inform potential treatments options.
The Extended Mammalian Circadian Timing System
The SCN (see Figure 1 ) is required for daily rhythms of physiology and behavior ( Moore and Eichler, 1972 ; Stephan and Zucker, 1972 ), and SCN neurons function as individual oscillators with rhythms of electrical activity that have period lengths of about 24 h ( Welsh et al., 1995 ). This electrical activity becomes highly coupled across SCN cells, resulting in an emergent ensemble circadian period ( Herzog et al., 1998 ). The electrical activity rhythms within individual SCN neurons are under the control of canonical “clock genes,” via a transcriptional-translational-post-translational negative feedback loop ( Gekakis et al., 1998 ; Jin et al., 1999 ). This genetic machinery has been found to be present in cells throughout the brain and body, however, the integrity of the SCN is necessary to synchronize these peripheral oscillators and maintain rhythmic behavior ( Mohawk et al., 2012 ). Specifically, SCN neuronal activity has been shown to be required for such circadian output, as the application of tetrodotoxin to the SCN in vivo reversibly disrupts circadian behavior, even while proper circadian timekeeping within the SCN remains intact ( Schwartz et al., 1987 ).
Suprachiasmatic nucleus neurons are predominately GABAergic ( Liu and Reppert, 2000 ), with subpopulations that differentially release several neuropeptides, including vasoactive intestinal peptide (VIP), arginine vasopressin (AVP), gastrin-releasing peptide (GRP), neuromedin S (NMS), and cholecystokinin (CCK). Some of these neuropeptides are arranged somatotopically, as the SCN is composed of “core” and “shell” subregions that express VIP and AVP, respectively ( Abrahamson and Moore, 2001 ). The VIP neurons within the SCN core receive direct retinal input and are required for normal circadian rhythmicity ( Harmar et al., 2002 ; Aton et al., 2005 ; Maywood et al., 2006 ). These VIP core neurons then appear to entrain the rhythmicity of AVP shell neurons and other SCN neuronal cell types in order to establish SCN-level synchrony ( Aton et al., 2005 ; Maywood et al., 2006 ). The subpopulation of SCN neurons expressing NMS have also been implicated as playing a crucial role in circadian pacemaking ( Lee et al., 2015 ), however, more recent work suggests that the critical neurons in this role belong to a molecularly distinct subpopulation that expresses both NMS and VIP together ( Todd et al., 2020 ). Interestingly, Todd et al. (2020) found that SCN VIP neurons that also contain NMS are enriched with the transcript Per2 associated with a core clock gene, whereas the non-NMS subpopulation of SCN VIP neurons that also contain GRP did not have such transcripts. Altogether, this suggests that SCN VIP neurons are composed of both pacemaker and non-pacemaker subpopulations, which is supported by previous work demonstrating that SCN VIP neurons can be divided into two groups based on the light-inducibility of clock genes, innervation of retinal afferents, day-night variability of VIP mRNA, and coexpression of GRP ( Kawamoto et al., 2003 ).
Suprachiasmatic nucleus neurons have been suggested to synchronize downstream molecular clocks and coordinate circadian rhythms via the release of humoral factors, as encapsulated implants of fetal tissue (which prevent the establishment of new neural connections) into SCN-ablated animals have been shown to restore modest behavioral rhythms ( Silver et al., 1996 ). Identified humoral factors that are released by the SCN and have been shown to modulate behavioral and physiological rhythms include transforming growth factor alpha and prokineticin 2 ( Cheng et al., 2002 ; Li et al., 2006 ; Gilbert and Davis, 2009 ). However, developmental work suggests that the influence of SCN humoral factors may decrease during the early postnatal period as axonal connections develop between the circadian system and downstream areas regulating behavioral state ( Gall et al., 2012 ; Blumberg et al., 2014 ). Overall, the SCN’s major axonal output pathway through the SPZ (see below) appears to be the primary method for synchronizing downstream oscillators and maintaining circadian rhythms of behavior ( Saper, 2013 ).
As also depicted in Figure 1 , the majority of axons emanating from the SCN synapse onto neurons within the SPZ, an adjacent region of GABAergic cells located just dorsal to the SCN and ventral to the paraventricular hypothalamus (PV) ( Watts and Swanson, 1987 ; Watts et al., 1987 ; Vujovic et al., 2015 ). Like the SCN, the SPZ displays circadian rhythms of multiunit activity in vivo ( Nakamura et al., 2008 ), and this output pathway has been hypothesized to be the primary circuit by which the SCN synchronizes organismal-level circadian rhythmicity ( Saper, 2013 ). Specifically, studies in rats have shown that circadian rhythms of sleep-wake, locomotor activity, and feeding behavior are regulated by a pathway from the SCN, through the SPZ, to the dorsomedial nucleus of the hypothalamus (DMH) ( Lu et al., 2001 ; Chou et al., 2003 ). As mentioned previously, it was recently demonstrated that rhythms of aggression propensity in male mice are regulated by SPZ neurons that project to VMH neurons known to promote attack behavior ( Todd et al., 2018 ). These SPZ neurons were found to be active during the early light phase, the resting phase for nocturnal mice, and disrupting their GABAergic transmission resulted in a time-dependent increase in behavioral aggression. Importantly, this time point is temporally analogous to the early resting phase in humans, when sundowning symptoms are most commonly reported in AD patients ( Boronat et al., 2019 ). In addition to aggression, neurons within the VMH have also been associated with the regulation of fear and anxiety ( Silva et al., 2013 ; Kunwar et al., 2015 ), raising the interesting possibility that the SCN → SPZ → VMH pathway may also influence circadian aspects of these emotional processes in a circadian fashion ( Bilu and Kronfeld-Schor, 2013 ; Albrecht and Stork, 2017 ). Given the wide range of rhythms that the SPZ appears to influence, this structure appears to be a likely candidate for which its dysfunction, or dysfunction of its inputs (from the SCN or elsewhere), could affect multiple aspects of circadian physiology and behavior that are seen in conditions such as sundowning syndrome.
Dysfunction Within Major Circadian Structures Associated With Aging and AD Pathology
Interestingly, separate studies have reported conflicting results regarding the direct impact of AD pathology on SCN VIP and AVP neurons in humans. Such studies are often complicated by the fact that researchers often do not have access to both hypothalamic tissue and the profile of circadian behavior of the same patients. However, one study examined hypothalamic tissue containing the SCN in aged patients that had at least 1 week of actigraphy data within 18 months of their death, and found that an age-related decline in VIP, but not AVP, SCN neurons was associated with increased circadian dysfunction ( Wang et al., 2015 ). A group of AD patients examined within this study, however, did not show a significantly greater loss of VIP SCN neurons compared to controls, even though they showed delayed acrophases of the locomotor activity rhythms. This led Wang et al. (2015) to suggest that structures that supply input to the SCN may instead be affected by AD pathology, therefore leading to a disruption of phase-setting and resulting in the delay found in their patients. Indeed, similar phase delays are a common report in AD and dementia patients from several other studies ( Harper et al., 2005 ; Schlosser Covell et al., 2012 ; Manni et al., 2019 ). One other study using actigraphy reported that a loss in AVP neurons in the SCN in AD patients was associated with fragmented rhythmicity compared to healthy aged-matched controls ( Harper et al., 2008 ), however, this group used a ratio of AVP neurons to glial cells in only a few selected fields of the SCN, whereas Wang et al. (2015) used a stereological rigorous method to quantify AVP and VIP throughout the entire nucleus. Finally, one other group reported reduced levels of AVP mRNA in the SCN but they did not count AVP mRNA-expressing neurons ( Liu et al., 2000 ), and the same group had previously reported no change in AVP-expressing SCN neurons in elderly dementia patients compared to healthy age-matched controls ( Swaab et al., 1985 ).
Evidence in healthy aging wild-type mice suggests an age-related decline in circadian output from the SCN to the SPZ ( Nakamura et al., 2011 ). These researchers saw a reduction in the circadian amplitude of multi-unit activity rhythms (MUA) in the SCN with age, as well as a similar reduction in the amplitude of MUA rhythms within the SPZ. While the interpretation of these findings as a dysfunction in SCN output is sound, it is possible that these results might also reflect an age-related dysfunction in the SPZ neurons’ ability to maintain rhythms as well (instead of only a decline in SCN output). Indeed, the SPZ has been largely overlooked as a possible locus for the circadian dysfunction that has been reported in neurobehavioral pathologies. However, since it regulates both sleep-wake and locomotor rhythms via its projections to the DMH, and also regulates the propensity for aggression via its projections to the VMH, the SPZ is in a logical position to underlie multiple symptoms associated with sundowning should its function become disrupted by AD pathology. It is interesting that, in both rats and mice, the SPZ has been shown to be composed of distinct subregions that differentially project to the DMH or VMH ( Vujovic et al., 2015 ; Todd et al., 2018 ). Therefore, differences in the degree of dysfunction caused by AD pathology in different SPZ subregions might explain the reported differences in sundowning symptoms across patients, as some studies have reported sundowning to mainly be composed of mood related disturbances such as agitation and aggression, as compared to sleep disturbances, whereas other studies have reported both ( Boronat et al., 2019 ).
Cholinergic Inputs to the Circadian System
Multiple studies have shown a cholinergic innervation of the SCN ( Ichikawa and Hirata, 1986 ; Kiss and Halasz, 1996 ; Castillo-Ruiz and Nunez, 2007 ), and that acetylcholine modulates the function of SCN neurons and circadian rhythmicity ( Liu and Gillette, 1996 ; Liu et al., 1997 ; Hut and Van der Zee, 2011 ; Gritton et al., 2013 ). In the field of AD research, the so-called “cholinergic hypothesis” has long posited that neurodegeneration of neurons in the basal forebrain (BF) expressing acetylcholine, which extensively project to cortical areas, underlie much of the memory and cognitive decline seen during the progression of the disease ( Contestabile, 2011 ; Craig et al., 2011 ; Pinto et al., 2011 ). Indeed, there is ample evidence in AD patients that the cholinergic neurons of sub-regions within the BF, such as the nucleus basalis of Meynert (NBM) ( Cummings and Benson, 1987 ; Vogels et al., 1990 ; Mesulam, 2013 ; Liu et al., 2015 ), are a major site of neurodegeneration in AD. Several groups have proposed that a cholinergic BF input to the SCN may also be disrupted in AD, leading to sundowning or other observed circadian deficits ( Klaffke and Staedt, 2006 ; Hut and Van der Zee, 2011 ; Bedrosian and Nelson, 2013 ). Lending some support to this hypothesis, acetylcholinesterase inhibitors including donepezil, have been found to ameliorate neuropsychiatric symptoms such as agitation and aggression ( Mega et al., 1999 ; Paleacu et al., 2002 ; Cummings et al., 2006 ; Carrasco et al., 2011 ). One case study also reported that donepezil reduced agitation and restlessness specifically in a sundowning dementia patient ( Skjerve and Nygaard, 2000 ). Interestingly, donepezil has also been shown to enhance rapid eye movement (REM) sleep in AD patients ( Moraes Wdos et al., 2006 ), but has also been associated with an increased prevalence of nightmares ( Ridha et al., 2018 ).
However, the evidence for the existence of a cholinergic pathway from the BF to the SCN comes from only one study in rats using non-specific retrograde tracing from the SCN, and then co-labeling for the cholinergic transporter (CHAT) in BF neurons ( Bina et al., 1993 ). Another study in rats suggested that chemical lesions of the NBM were associated with a reduction in VIP and AVP synthesis and expression in the SCN, however, anatomical tracing was not done in these experiments ( Madeira et al., 2004 ). Importantly, recent work using more selective genetically targeted tracing from the BF in CHAT-IRES-Cre mice reported no evidence of such a cholinergic BF to SCN pathway ( Agostinelli et al., 2019 ). While it is possible that this findings represent a species difference between mice and rats, the conservation of a BF to SCN pathway across mammalian species warrants further investigation ( Figure 1 ). Yet, this does not discredit a cholinergic input to the SCN from other areas, such as the brainstem. Indeed, the same authors who reported the cholinergic BF to SCN in rats also reported retrogradedly labeled CHAT cells in the laterodorsal tegmentum (LDT) and pedunculopontine tegmentum (PPT) complex of the brainstem ( Bina et al., 1993 ). The LDT/PPT has been shown to display tau pathology in AD patients, but interestingly, not cholinergic cell loss ( Mufson et al., 1988 ; Dugger et al., 2012 ; Kotagal et al., 2012 ). It may be possible that aging and tau pathology could disrupt the function of the LDT/PPT to SCN pathway, even without causing the loss of cholinergic cells, as synaptic changes associated with high levels of soluble of amyloid-β or tau have been reported to appear well before the insoluble plaques or tangles themselves ( Scheff et al., 2006 ; D’Amelio et al., 2011 ).
In addition to cholinergic neurons, however, the LDT/PPT complex also contains GABAergic and glutamatergic neurons that are known to play different roles in sleep-wake regulation ( Kroeger et al., 2017 ). It is unclear whether these cell populations also project to the SCN and influence circadian function or aggression; a cell type-specific approach to examine the presence of such pathways and their function would be greatly informative. And, although cholinergic cells appear to be spared in the LDT/PPT of AD patients, the presence of tau pathology in this region could instead lead to neurodegeneration of these GABAergic and glutamaterigic populations, which does not appear to have been previously examined. Interestingly, Bedrosian et al. (2011) showed reduced global c-Fos expression (a marker of neuronal activation) in PPT neurons in aged mice compared to healthy adult mice, which was also associated with temporal changes in anxiety behavior. These authors also found similar time-dependent changes in anxiety behavior in APP mice (which bear amyloid-β pathology), but at even earlier ages (however, it does not appear that c-Fos was examined in the PPT in these APP mice).
Serotonergic Inputs to the Circadian System
There is also substantial evidence for a role in the disruption of serotonergic neurons in AD ( Rodriguez et al., 2012 ; Vakalopoulos, 2017 ; Chakraborty et al., 2019 ), and serotonin is also known to play role in circadian regulation ( Ciarleglio et al., 2011 ; Daut and Fonken, 2019 ). Several studies have suggested a dense serotonergic input from the midbrain raphe complex to the SCN and SPZ (see Figure 1 ). In hamsters, these serotonergic inputs appear to arise primarily from the median raphe nucleus (MRN) ( Meyer-Bernstein and Morin, 1996 ; Leander et al., 1998 ; Yamakawa and Antle, 2010 ), while studies in rats have revealed serotonergic inputs from both the MRN and dorsal raphe nucleus (DRN) ( Kawano et al., 1996 ; Moga and Moore, 1997 ). Such serotonergic inputs to the SCN appear to play a role in setting circadian phase, as administration of serotonin or serotonergic agonists into the SCN has been shown to produce phase shifts during certain parts of the light-dark cycle ( Lovenberg et al., 1993 ; Ehlen et al., 2001 ; Sprouse et al., 2004 ). Additionally, developmental disruption of the serotonin transcription factor Pet-1 disrupts locomotor activity rhythms and in vitro SCN activity ( Ciarleglio et al., 2014 ). Serotonergic function is also highly implicated in the direct regulation of aggression ( Nautiyal et al., 2015 ; Niederkofler et al., 2016 ), and serotonergic neurons have been shown to project from the raphe complex to the VMH ( Kanno et al., 2008 ). So, its possible that AD-related disruptions in serotonergic signaling also underlie overall levels of aggression, as well as differences at certain times of the day. Indeed, several groups have reported serotonergic deficiencies in AD that were associated with either increased circadian dysfunction or behavioral aggression ( Lai et al., 2003 ; Vermeiren et al., 2014 ; Chakraborty et al., 2019 ).
Serotonergic drugs have commonly been administered to AD patients in order to treat aggression, anxiety, and other emotional behavioral disturbances, as well as to treat sleep-wake and circadian disruption. Citalopram, a selective serotonin reuptake inhibitor (SSRI) widely used as an antidepressant, has been found to reduce irritability, anxiety, and aggression in moderately agitated AD patients ( Leonpacher et al., 2016 ; Schneider et al., 2016 ), however, it was much less effective in the severely agitated patients. Interestingly, patients categorized as the most severely agitated actually showed an increase in nighttime behavioral or sleep disruptions when treated with citalopram ( Leonpacher et al., 2016 ). An intriguing body of work also suggests that early administration of SSRIs may also slow the progression of mild cognitive impairment to AD, perhaps through a mechanism by which serotonin affects the amyloid-β precursor protein, thereby reducing the accumulation of amyloid-β ( Elsworthy and Aldred, 2019 ). It is also possible that serotonin’s modulation of the circadian system could indirectly play a role in slowing or expediting this progression, as chronic circadian dysfunction exacerbates AD pathology ( Musiek, 2015 ). Indeed, trazodone, a serotonin antagonist and reuptake inhibitor (SARI) that is also commonly used as an antidepressant, has been shown to improve circadian function and sleep-wake rhythms in AD patients ( Camargos et al., 2014 ; Grippe et al., 2015 ). Risperidone and olanzapine, both atypical antipsychotics and antagonists for serotonin (as well as for dopamine), have been shown to have differential effects in AD patients. Risperidone, but not olanzapine, was found to reduce aggression and other neuropsychiatric symptoms in AD patients ( Nagata et al., 2017 ), whereas a separate study in AD patients found olanzapine to reduce anxiety ( Mintzer et al., 2001 ). While serotonin has historically been implicated in sleep-wake regulation, a recent study demonstrated that DRN serotonergic neurons actually promote sleep through anxiolysis, further highlighting the critical role of serotonin in mood and emotional regulation ( Venner et al., 2020 ).
The Retinohypothalamic Tract
Perhaps the most extensively studied input to the SCN comes from a distinct set of retinal ganglion cells (RCGs) (see Figure 1 ), via the retinohypothalamic tract (RHT) ( Moore et al., 1995 ). This pathway is required for circadian photoentrainment, as shown by enucleation studies where removing both eyes results in free-running rhythms under a light-dark cycle ( Nelson and Zucker, 1981 ; Foster et al., 1991 ). A subset set of RGCs are intrinsically photosensitive and contain the photopigment melanopsin ( Berson et al., 2002 ; Hattar et al., 2002 ; Sekaran et al., 2003 ). These melanopsin cells themselves comprise 5 different subtypes (M1–M5-type) ( Ecker et al., 2010 ), and evidence suggests that a molecularly distinct subpopulation of M1-type RGCs, defined by their lack of expression of the transcription factor Brn3b and numbering around only 200 cells, are sufficient for driving entrainment of the SCN ( Chen et al., 2011 ). To enable such photoentrainment, the RHT releases glutamate and pituitary adenylate cyclase activating polypeptide (PACAP) onto the SCN ( Hannibal et al., 2000 ). While light during the day is required for proper circadian photoentrainment, light exposure at night has been shown to be deleterious to mood regulation and overall circadian function ( Fonken and Nelson, 2014 ; Bedrosian and Nelson, 2017 ). Evidence in AD patients suggests altered function of melanopsin RGCs in preclinical AD ( Oh et al., 2019 ), and post mortem studies suggest an AD-related loss of melanopsin RGCs ( La Morgia et al., 2016 ). Bright light therapy has already been shown to improve circadian rhythmicity and mood in AD patients ( Figueiro et al., 2014 ; Munch et al., 2017 ; Wahnschaffe et al., 2017 ). Additionally, one study reported that morning light exposure shifted the peak of agitated behavior in patients with severe AD ( Ancoli-Israel et al., 2003 ).
Another interesting possibility for a future treatment of sundowning via this pathway could be using intravitrial injections of chemogenetic vectors into the eye and driving activity of RGCs via peripheral injection of the chemogenetic ligand. A similar strategy has been suggested to show promise as a potential therapy for other mood-related disorders ( Bowrey et al., 2017 ; Venner et al., 2019 ). Interestingly, the RHT has been shown to densely project to the SPZ in some species, but not in others ( Figure 1 ), and this pathway has been suggested to play a role in modifying nocturnal versus diurnal sleep-wake behavior in a species typical manner ( Todd et al., 2012 ). Understanding how such species differences impact circadian function will be vital for teasing apart the underlying factors contributing to circadian phase preference (diurnality versus nocturnality), which will be critical for properly translating the findings of AD-related research in nocturnal rodents into potential treatment applications in diurnal AD patients.
The Geniculohypothalamic Tract
As also depicted in Figure 1 , the SCN and SPZ are also known to receive input from photo receipient structures in the thalamus, the ventral lateral geniculate nucleus (LGN) and the adjacent intergeniculate leaflet (IGL), via the geniculohypothalamic tract (GHT) ( Moore et al., 2000 ). Importantly, AD patients have been reported to show significant amyloid-β pathology in the LGN ( Erskine et al., 2016 ). The GHT pathway releases GABA and neuropeptide Y (NPY), and this input has been shown to influence the response of SCN neurons to light, as well as to play a critical role in non-photic entrainment ( Mrosovsky, 1996 ; Harrington, 1997 ). For instance, giving rodents time-dependent access to a novel running wheel leads to non-photic phase advances and ultimately entrainment of the circadian system ( Reebs and Mrosovsky, 1989 ). NPY released from the IGL neurons that make up the GHT appears to underlie these non-photic effects on the SCN, as novelty-induced wheel running induces c-Fos expression in the IGL NPY neurons ( Janik and Mrosovsky, 1992 ), and infusions of NPY directly into the SCN produce similar phase shifts ( Albers and Ferris, 1984 ; Huhman and Albers, 1994 ). To further support this view, electrolytic lesions of the IGL ( Janik and Mrosovsky, 1994 ), and SCN infusion of NPY antiserum ( Biello et al., 1994 ), both block the phase-advancing effect produced by novelty-induce wheel running. Interestingly, these results may suggest a pathway by which daily exercise at a consistent time could improve circadian function and reduce possible sundowning symptoms in AD and dementia patients, which has previously been suggested as a strategy to counteract attenuation of circadian rhythms that come with normal aging and AD ( Duncan, 2020 ). Lending support to this idea, timed access to a running wheel has already been shown to increase the robustness of circadian behavioral rhythms in mice lacking the VIP receptor, VPAC2 ( Power et al., 2010 ). Additionally, two separate studies have indicated that daily exercise enhances circadian cortisol rhythms in patients with AD or mild cognitive impairment ( Tortosa-Martinez et al., 2015 ; Venturelli et al., 2016 ). Moreover, one group found that routine walking at certain times of the day ameliorated sundowning symptoms in AD patients in two separate studies ( Shih et al., 2017 ; Shih et al., 2019 ).
Other Neurotransmitter Inputs to the SCN: Dopamine and Orexin
While less is known about their potential role in AD and related dementias, there is some evidence to suggest that dopaminergic and orexinergic inputs to the SCN may could also be comprised in these neurodegenerative diseases. For instance, recent work has implicated an important role for dopaminergic input to the SCN from the ventral tegmental area (VTA) ( Grippo et al., 2017 ). Grippo et al. (2017) demonstrated that this dopaminergic input to the SCN is important for resynchronizing locomotor activity rhythms to shifts of the light-dark cycle, and that elevating levels of dopamine in the SCN actually accelerates photoentrainment. Interestingly, work in a transgenic mouse model of AD pathology revealed degeneration of VTA dopaminergic neurons ( Nobili et al., 2017 ), and a imaging study in prodromal AD patients suggest a decrease in VTA volume ( De Marco and Venneri, 2018 ).
Additionally, the neurotransmitter orexin, located in the lateral hypothalamus and perifornical region ( de Lecea et al., 1998 ), is known for its role in maintaining consolidated wakefulness as the degeneration of orexinergic neurons results in the sleep disorder narcolepsy ( Lin et al., 1999 ). Orexinergic fibers have also been shown to project to the SCN ( Backberg et al., 2002 ), and have been shown to modulate SCN activity ( Belle et al., 2014 ). Belle et al. (2014) found that orexin is upregulated at dusk in nocturnal mice, and suppresses the activity of SCN neurons that specifically express the clock gene Per1 . These authors also demonstrated that orexin enhances the resetting ability of NPY in the SCN (that has been released from the IGL), highlighting how multiple input pathways may act together to modulate circadian rhythmicity. Interestingly, AD patients with high levels of neuropsychiatric symptoms, including agitation and aggression, have been found to have higher overall levels of orexinergic tone and fragmented sleep ( Liguori et al., 2018 ). Indeed, other studies have found that similarly high cerebrospinal fluid (CSF) levels of orexin in AD patients are also associated with increased amyloid-β levels ( Gabelle et al., 2017 ). While promising, more work is needed to better understand the role of orexinergic and dopaminergic influence on the circadian system in AD, in order to delineate their respective potential contributions to sundowning symptoms.
Several characteristic non-cognitive symptoms of AD and related dementias involve behavioral and physiological processes known to be regulated by the hypothalamus ( Ishii and Iadecola, 2015 ; Hiller and Ishii, 2018 ). These include, among others, circadian and sleep-wake dysfunction, and emotional behavioral disruptions such as agitation and aggression. These particular non-cognitive symptoms are comorbid in the clinical phenomenon known as sundowning syndrome. Whether this term is an appropriate descriptor is debatable, given that the direct linkage of this phenomenon to sunset is not always supported ( Yesavage et al., 2003 ). However, the weight of the evidence does suggest that a time-dependent exacerbation of emotional behavioral disturbances, including agitation and aggression, is prevalent in AD and dementia patients during the late afternoon and early evening ( Boronat et al., 2019 ). Interestingly, this phenomenon seems to be less connected to sleep disruption, per se , and more directly tied to disturbances in emotional state.
Although sundowning has been studied for several decades, its cause remains unclear. Evidence from basic research ( Bedrosian and Nelson, 2013 ; Todd et al., 2018 ), along with pathological findings from AD patients ( Wang et al., 2015 ; Erskine et al., 2016 ; Chakraborty et al., 2019 ), suggests several pathways that might be involved in the circadian dysfunction, and agitation and aggression, underlying sundowning. There is a strong association between circadian rhythms and emotional regulation ( Hood and Amir, 2018 ; Ketchesin et al., 2020 ), and the shared circuitry between these two systems presents potential candidates for such pathology-related dysfunction. These include the major circadian structures themselves, the SCN, SPZ and its output pathways to the DMH and VMH ( Venner et al., 2019 ), as well as several structures that project to circadian system.
Disrupting GABAergic transmission from SPZ cells that project to the VMH has been shown to cause increased behavioral aggression during the early resting phase, when these cells have been shown to be active in a time dependent manner ( Todd et al., 2018 ). There is also some evidence for LDT/PPT dysfunction associated with a time-dependent change in anxiety in aged mice ( Bedrosian et al., 2011 ) (but it is unclear whether the affected cells are cholinergic, glutamatergic, or GABAergic), and LDT/PPT neurons also to project to the SCN ( Bina et al., 1993 ). Serotonergic function seems to be dysregulated in AD ( Rodriguez et al., 2012 ; Vakalopoulos, 2017 ), and serotonergic neurons of the midbrain raphe complex project to and modulate the circadian system and are highly involved in mood regulation and aggression ( Ciarleglio et al., 2011 ; Niederkofler et al., 2016 ; Daut and Fonken, 2019 ). Direct retinal input to the SCN, as well as the direct NPY input from the retinorecipient IGL, are also possible candidates as pathology and dysfunction have been reported in these structures in AD ( Erskine et al., 2016 ; La Morgia et al., 2016 ), and properly timed light exposure improves circadian rhythms and mood whereas ill-timed light exposure has deleterious effects ( Bedrosian and Nelson, 2017 ). Altogether, a better understanding of the role of these pathways in behavioral and emotional timing will be important for treating circadian dysfunction and sundowning-related symptoms in AD and dementia patients and may also lead to the identification of important early indicators of the progression of AD. For instance, agitation and aggression have been found to be important predictors of the progression from mild cognitive impairment to probable AD, suggesting such behavior could be an important early indicator that could inform treatment options ( Dietlin et al., 2019 ). Similarly, circadian dysfunction of locomotor activity has been shown to be present in preclinical AD patients, well before the cognitive and amnesiac symptoms appear ( Musiek et al., 2018 ). Thus, such interventions hold the promise of improving quality of life for both patient and caregiver, and may even slow the progression of the disease.
Author Contributions
The author confirms being the sole contributor of this work and has approved it for publication.
Funding was provided by the National Institute on Aging (R03 AG062883-01).
Conflict of Interest
The author declares that the research was conducted in the absence of any commercial or financial relationships that could be construed as a potential conflict of interest.
Acknowledgments
I sincerely thank Pooja Gupta, Aaron Wilke, Mikayla Carlson, and Daniel Kroeger for helpful discussions and comments regarding this manuscript.
Abrahamson, E. E., and Moore, R. Y. (2001). Suprachiasmatic nucleus in the mouse: retinal innervation, intrinsic organization and efferent projections. Brain Res 916, 172–191. doi: 10.1016/s0006-8993(01)02890-6
CrossRef Full Text | Google Scholar
Aging, N. I. O. (2017). Tips for Coping with Sundowning [Online]. Available: https://www.nia.nih.gov/health/tips-coping-sundowning (accessed May 1, 2020).
Google Scholar
Agostinelli, L. J., Geerling, J. C., and Scammell, T. E. (2019). Basal forebrain subcortical projections. Brain Struct. Funct. 224, 1097–1117. doi: 10.1007/s00429-018-01820-6
PubMed Abstract | CrossRef Full Text | Google Scholar
Albers, H. E., and Ferris, C. F. (1984). Neuropeptide Y: role in light-dark cycle entrainment of hamster circadian rhythms. Neurosci. Lett. 50, 163–168. doi: 10.1016/0304-3940(84)90480-4
Albrecht, A., and Stork, O. (2017). Circadian rhythms in fear conditioning: an overview of behavioral, brain system, and molecular interactions. Neural Plast. 2017:3750307.
Ancoli-Israel, S., Martin, J. L., Gehrman, P., Shochat, T., Corey-Bloom, J., Marler, M., et al. (2003). Effect of light on agitation in institutionalized patients with severe Alzheimer disease. Am. J. Geriatr. Psychiatry 11, 194–203. doi: 10.1097/00019442-200303000-00010
Angulo Sevilla, D., Carreras Rodriguez, M. T., Heredia Rodriguez, P., Fernandez Sanchez, M., Vivancos Mora, J. A., and Gago-Veiga, A. B. (2018). Is there a characteristic clinical profile for patients with dementia and sundown syndrome? J. Alzheimers Dis. 62, 335–346. doi: 10.3233/jad-170488
Asaoka, S., Aritake, S., Komada, Y., Ozaki, A., Odagiri, Y., Inoue, S., et al. (2013). Factors associated with shift work disorder in nurses working with rapid-rotation schedules in Japan: the nurses’ sleep health project. Chronobiol. Int. 30, 628–636. doi: 10.3109/07420528.2012.762010
Association, A. S. (2020). Sleep Issues and Sundowning [Online]. Available: https://www.alz.org/help-support/caregiving/stages-behaviors/sleep-issues-sundowning (accessed May 1, 2020).
Aton, S. J., Colwell, C. S., Harmar, A. J., Waschek, J., and Herzog, E. D. (2005). Vasoactive intestinal polypeptide mediates circadian rhythmicity and synchrony in mammalian clock neurons. Nat. Neurosci. 8, 476–483. doi: 10.1038/nn1419
Bachman, D., and Rabins, P. (2006). “Sundowning” and other temporally associated agitation states in dementia patients. Annu. Rev. Med. 57, 499–511. doi: 10.1146/annurev.med.57.071604.141451
Backberg, M., Hervieu, G., Wilson, S., and Meister, B. (2002). Orexin receptor-1 (Ox-R1) immunoreactivity in chemically identified neurons of the hypothalamus: focus on orexin targets involved in control of food and water intake. Eur. J. Neurosci. 15, 315–328. doi: 10.1046/j.0953-816x.2001.01859.x
Bedrosian, T. A., Herring, K. L., Weil, Z. M., and Nelson, R. J. (2011). Altered temporal patterns of anxiety in aged and amyloid precursor protein (APP) transgenic mice. Proc. Natl. Acad. Sci. U.S.A. 108, 11686–11691. doi: 10.1073/pnas.1103098108
Bedrosian, T. A., and Nelson, R. J. (2013). Sundowning syndrome in aging and dementia: research in mouse models. Exp. Neurol. 243, 67–73. doi: 10.1016/j.expneurol.2012.05.005
Bedrosian, T. A., and Nelson, R. J. (2017). Timing of light exposure affects mood and brain circuits. Transl. Psychiatry 7:e1017. doi: 10.1038/tp.2016.262
Belle, M. D., Hughes, A. T., Bechtold, D. A., Cunningham, P., Pierucci, M., Burdakov, D., et al. (2014). Acute suppressive and long-term phase modulation actions of orexin on the mammalian circadian clock. J. Neurosci. 34, 3607–3621. doi: 10.1523/jneurosci.3388-13.2014
Berson, D. M., Dunn, F. A., and Takao, M. (2002). Phototransduction by retinal ganglion cells that set the circadian clock. Science 295, 1070–1073. doi: 10.1126/science.1067262
Biello, S. M., Janik, D., and Mrosovsky, N. (1994). Neuropeptide Y and behaviorally induced phase shifts. Neuroscience 62, 273–279. doi: 10.1016/0306-4522(94)90331-x
Bilu, C., and Kronfeld-Schor, N. (2013). Effects of circadian phase and melatonin injection on anxiety-like behavior in nocturnal and diurnal rodents. Chronobiol. Int. 30, 828–836. doi: 10.3109/07420528.2013.773439
Bina, K. G., Rusak, B., and Semba, K. (1993). Localization of cholinergic neurons in the forebrain and brainstem that project to the suprachiasmatic nucleus of the hypothalamus in rat. J. Comp. Neurol. 335, 295–307. doi: 10.1002/cne.903350212
Bliwise, D. L., Carroll, J. S., Lee, K. A., Nekich, J. C., and Dement, W. C. (1993). Sleep and ”sundowning” in nursing home patients with dementia. Psychiatry Res. 48, 277–292. doi: 10.1016/0165-1781(93)90078-u
Blumberg, M. S., Gall, A. J., and Todd, W. D. (2014). The development of sleep-wake rhythms and the search for elemental circuits in the infant brain. Behav. Neurosci. 128, 250–263. doi: 10.1037/a0035891
Boronat, A. C., Ferreira-Maia, A. P., and Wang, Y. P. (2019). Sundown syndrome in older persons: a scoping review. J. Am. Med. Dir. Assoc. 20, 664.e5–671.e5.
Bowrey, H. E., James, M. H., and Aston-Jones, G. (2017). New directions for the treatment of depression: targeting the photic regulation of arousal and mood (PRAM) pathway. Depress. Anxiety 34, 588–595. doi: 10.1002/da.22635
Bronsard, G., and Bartolomei, F. (2013). Rhythms, rhythmicity and aggression. J. Physiol. Paris 107, 327–334. doi: 10.1016/j.jphysparis.2013.03.002
Burgio, L. D., Scilley, K., Hardin, J. M., Janosky, J., Bonino, P., Slater, S. C., et al. (1994). Studying disruptive vocalization and contextual factors in the nursing home using computer-assisted real-time observation. J. Gerontol. 49, 230–239.
Camargos, E. F., Louzada, L. L., Quintas, J. L., Naves, J. O., Louzada, F. M., and Nobrega, O. T. (2014). Trazodone improves sleep parameters in Alzheimer disease patients: a randomized, double-blind, and placebo-controlled study. Am. J. Geriatr. Psychiatry 22, 1565–1574. doi: 10.1016/j.jagp.2013.12.174
Cameron, D. E. (1941). Studies in senile nocturnal delirium. Psychiatr. Q. 15, 47–53. doi: 10.1007/bf01613953
Canevelli, M., Valletta, M., Trebbastoni, A., Sarli, G., D’antonio, F., Tariciotti, L., et al. (2016). Sundowning in dementia: clinical relevance, pathophysiological determinants, and therapeutic approaches. Front. Med. 3:73. doi: 10.3389/fmed.2016.00073
Carrasco, M. M., Aguera, L., Gil, P., Morinigo, A., and Leon, T. (2011). Safety and effectiveness of donepezil on behavioral symptoms in patients with Alzheimer disease. Alzheimer Dis. Assoc. Disord. 25, 333–340. doi: 10.1097/wad.0b013e318212ab7a
Castillo-Ruiz, A., and Nunez, A. A. (2007). Cholinergic projections to the suprachiasmatic nucleus and lower subparaventricular zone of diurnal and nocturnal rodents. Brain Res. 1151, 91–101. doi: 10.1016/j.brainres.2007.03.010
Chakraborty, S., Lennon, J. C., Malkaram, S. A., Zeng, Y., Fisher, D. W., and Dong, H. (2019). Serotonergic system, cognition, and Bpsd in Alzheimer’s disease. Neurosci. Lett. 704, 36–44. doi: 10.1016/j.neulet.2019.03.050
Chen, S. K., Badea, T. C., and Hattar, S. (2011). Photoentrainment and pupillary light reflex are mediated by distinct populations of ipRGCs. Nature 476, 92–95. doi: 10.1038/nature10206
Cheng, M. Y., Bullock, C. M., Li, C., Lee, A. G., Bermak, J. C., Belluzzi, J., et al. (2002). Prokineticin 2 transmits the behavioural circadian rhythm of the suprachiasmatic nucleus. Nature 417, 405–410. doi: 10.1038/417405a
Chou, T. C., Scammell, T. E., Gooley, J. J., Gaus, S. E., Saper, C. B., and Lu, J. (2003). Critical role of dorsomedial hypothalamic nucleus in a wide range of behavioral circadian rhythms. J. Neurosci. 23, 10691–10702. doi: 10.1523/jneurosci.23-33-10691.2003
Ciarleglio, C. M., Resuehr, H. E., Axley, J. C., Deneris, E. S., and Mcmahon, D. G. (2014). Pet-1 deficiency alters the circadian clock and its temporal organization of behavior. PLoS One 9:e97412. doi: 10.1371/journal.pone.0097412
Ciarleglio, C. M., Resuehr, H. E., and Mcmahon, D. G. (2011). Interactions of the serotonin and circadian systems: nature and nurture in rhythms and blues. Neuroscience 197, 8–16. doi: 10.1016/j.neuroscience.2011.09.036
Cohen-Mansfield, J. (2007). Temporal patterns of agitation in dementia. Am. J. Geriatr. Psychiatry 15, 395–405. doi: 10.1097/01.jgp.0000247162.59666.68
Cohen-Mansfield, J., Marx, M. S., Werner, P., and Freedman, L. (1992). Temporal patterns of agitated nursing home residents. Int. Psychogeriatr. 4, 197–206. doi: 10.1017/s1041610292001029
Contestabile, A. (2011). The history of the cholinergic hypothesis. Behav. Brain Res. 221, 334–340. doi: 10.1016/j.bbr.2009.12.044
Corder, E. H., Saunders, A. M., Strittmatter, W. J., Schmechel, D. E., Gaskell, P. C., Small, G. W., et al. (1993). Gene dose of apolipoprotein E type 4 allele and the risk of Alzheimer’s disease in late onset families. Science 261, 921–923. doi: 10.1126/science.8346443
Craig, L. A., Hong, N. S., and Mcdonald, R. J. (2011). Revisiting the cholinergic hypothesis in the development of Alzheimer’s disease. Neurosci. Biobehav. Rev. 35, 1397–1409. doi: 10.1016/j.neubiorev.2011.03.001
Cummings, J. L., and Benson, D. F. (1987). The role of the nucleus basalis of Meynert in dementia: review and reconsideration. Alzheimer Dis. Assoc. Disord. 1, 128–155.
Cummings, J. L., Mcrae, T., Zhang, R., and Donepezil-Sertraline Study, G. (2006). Effects of donepezil on neuropsychiatric symptoms in patients with dementia and severe behavioral disorders. Am. J. Geriatr. Psychiatry 14, 605–612. doi: 10.1097/01.jgp.0000221293.91312.d3
D’Amelio, M., Cavallucci, V., Middei, S., Marchetti, C., Pacioni, S., Ferri, A., et al. (2011). Caspase-3 triggers early synaptic dysfunction in a mouse model of Alzheimer’s disease. Nat. Neurosci. 14, 69–76. doi: 10.1038/nn.2709
Daut, R. A., and Fonken, L. K. (2019). Circadian regulation of depression: a role for serotonin. Front. Neuroendocrinol. 54:100746. doi: 10.1016/j.yfrne.2019.04.003
de Lecea, L., Kilduff, T. S., Peyron, C., Gao, X., Foye, P. E., Danielson, P. E., et al. (1998). The hypocretins: hypothalamus-specific peptides with neuroexcitatory activity. Proc. Natl. Acad. Sci. U.S.A. 95, 322–327. doi: 10.1073/pnas.95.1.322
De Marco, M., and Venneri, A. (2018). Volume and connectivity of the ventral tegmental area are linked to neurocognitive signatures of Alzheimer’s disease in humans. J. Alzheimers Dis. 63, 167–180. doi: 10.3233/jad-171018
Deibel, S. H., Mcdonald, R. J., and Kolla, N. J. (2020). Are owls and larks different when it comes to aggression? Genetics, neurobiology, and behavior. Front. Behav. Neurosci. 14:39. doi: 10.3389/fnbeh.2020.00039
Dietlin, S., Soto, M., Kiyasova, V., Pueyo, M., De Mauleon, A., Delrieu, J., et al. (2019). Neuropsychiatric symptoms and risk of progression to Alzheimer’s disease among mild cognitive impairment subjects. J. Alzheimers Dis. 70, 25–34. doi: 10.3233/jad-190025
Dugger, B. N., Murray, M. E., Boeve, B. F., Parisi, J. E., Benarroch, E. E., Ferman, T. J., et al. (2012). Neuropathological analysis of brainstem cholinergic and catecholaminergic nuclei in relation to rapid eye movement (REM) sleep behaviour disorder. Neuropathol. Appl. Neurobiol. 38, 142–152. doi: 10.1111/j.1365-2990.2011.01203.x
Duncan, M. J. (2020). Interacting influences of aging and Alzheimer’s disease on circadian rhythms. Eur. J. Neurosci. 51, 310–325. doi: 10.1111/ejn.14358
Ecker, J. L., Dumitrescu, O. N., Wong, K. Y., Alam, N. M., Chen, S. K., Legates, T., et al. (2010). Melanopsin-expressing retinal ganglion-cell photoreceptors: cellular diversity and role in pattern vision. Neuron 67, 49–60. doi: 10.1016/j.neuron.2010.05.023
Ehlen, J. C., Grossman, G. H., and Glass, J. D. (2001). In vivo resetting of the hamster circadian clock by 5-Ht7 receptors in the suprachiasmatic nucleus. J. Neurosci. 21, 5351–5357. doi: 10.1523/jneurosci.21-14-05351.2001
Elsworthy, R. J., and Aldred, S. (2019). Depression in Alzheimer’s disease: an alternative role for selective serotonin reuptake inhibitors? J. Alzheimers Dis. 69, 651–661. doi: 10.3233/jad-180780
Erskine, D., Taylor, J. P., Firbank, M. J., Patterson, L., Onofrj, M., O’brien, J. T., et al. (2016). Changes to the lateral geniculate nucleus in Alzheimer’s disease but not dementia with Lewy bodies. Neuropathol. Appl. Neurobiol. 42, 366–376. doi: 10.1111/nan.12249
Evans, L. K. (1987). Sundown syndrome in institutionalized elderly. J. Am. Geriatr. Soc. 35, 101–108. doi: 10.1111/j.1532-5415.1987.tb01337.x
Figueiro, M. G., Plitnick, B. A., Lok, A., Jones, G. E., Higgins, P., Hornick, T. R., et al. (2014). Tailored lighting intervention improves measures of sleep, depression, and agitation in persons with Alzheimer’s disease and related dementia living in long-term care facilities. Clin. Interv. Aging 9, 1527–1537.
Fonken, L. K., and Nelson, R. J. (2014). The effects of light at night on circadian clocks and metabolism. Endocr. Rev. 35, 648–670. doi: 10.1210/er.2013-1051
Foster, R. G., Provencio, I., Hudson, D., Fiske, S., De Grip, W., and Menaker, M. (1991). Circadian photoreception in the retinally degenerate mouse (rd/rd). J. Comp. Physiol. A 169, 39–50. doi: 10.1007/bf00198171
Friedman, L., Kraemer, H. C., Zarcone, V., Sage, S., Wicks, D., Bliwise, D. L., et al. (1997). Disruptive behavior and actigraphic measures in home-dwelling patients with Alzheimer’s disease: preliminary report. J. Geriatr. Psychiatry Neurol. 10, 58–62. doi: 10.1177/089198879701000204
Gabelle, A., Jaussent, I., Hirtz, C., Vialaret, J., Navucet, S., Grasselli, C., et al. (2017). Cerebrospinal fluid levels of orexin-A and histamine, and sleep profile within the Alzheimer process. Neurobiol. Aging 53, 59–66. doi: 10.1016/j.neurobiolaging.2017.01.011
Gall, A. J., Todd, W. D., and Blumberg, M. S. (2012). Development of Scn connectivity and the circadian control of arousal: a diminishing role for humoral factors? PLoS One 7:e45338. doi: 10.1371/journal.pone.0045338
Gallagher-Thompson, D., Brooks, J. O. III, Bliwise, D., Leader, J., and Yesavage, J. A. (1992). The relations among caregiver stress, ”sundowning” symptoms, and cognitive decline in Alzheimer’s disease. J. Am. Geriatr. Soc. 40, 807–810. doi: 10.1111/j.1532-5415.1992.tb01853.x
Gekakis, N., Staknis, D., Nguyen, H. B., Davis, F. C., Wilsbacher, L. D., King, D. P., et al. (1998). Role of the Clock protein in the mammalian circadian mechanism. Science 280, 1564–1569. doi: 10.1126/science.280.5369.1564
Gilbert, J., and Davis, F. C. (2009). Behavioral effects of systemic transforming growth factor-alpha in Syrian hamsters. Behav. Brain Res. 198, 440–448. doi: 10.1016/j.bbr.2008.11.046
Grippe, T. C., Goncalves, B. S., Louzada, L. L., Quintas, J. L., Naves, J. O., Camargos, E. F., et al. (2015). Circadian rhythm in Alzheimer disease after trazodone use. Chronobiol. Int. 32, 1311–1314. doi: 10.3109/07420528.2015.1077855
Grippo, R. M., Purohit, A. M., Zhang, Q., Zweifel, L. S., and Guler, A. D. (2017). Direct midbrain dopamine input to the suprachiasmatic nucleus accelerates circadian entrainment. Curr. Biol. 27:e3.
Gritton, H. J., Stasiak, A. M., Sarter, M., and Lee, T. M. (2013). Cognitive performance as a zeitgeber: cognitive oscillators and cholinergic modulation of the Scn entrain circadian rhythms. PLoS One 8:e56206. doi: 10.1371/journal.pone.0056206
Hannibal, J., Moller, M., Ottersen, O. P., and Fahrenkrug, J. (2000). PACAP and glutamate are co-stored in the retinohypothalamic tract. J. Comp. Neurol. 418, 147–155. doi: 10.1002/(sici)1096-9861(20000306)418:2<147::aid-cne2>3.0.co;2-#
Harmar, A. J., Marston, H. M., Shen, S., Spratt, C., West, K. M., Sheward, W. J., et al. (2002). The Vpac(2) receptor is essential for circadian function in the mouse suprachiasmatic nuclei. Cell 109, 497–508. doi: 10.1016/s0092-8674(02)00736-5
Harper, D. G., Stopa, E. G., Kuo-Leblanc, V., Mckee, A. C., Asayama, K., Volicer, L., et al. (2008). Dorsomedial SCN neuronal subpopulations subserve different functions in human dementia. Brain 131, 1609–1617. doi: 10.1093/brain/awn049
Harper, D. G., Volicer, L., Stopa, E. G., Mckee, A. C., Nitta, M., and Satlin, A. (2005). Disturbance of endogenous circadian rhythm in aging and Alzheimer disease. Am. J. Geriatr. Psychiatry 13, 359–368. doi: 10.1097/00019442-200505000-00004
Harrington, M. E. (1997). The ventral lateral geniculate nucleus and the intergeniculate leaflet: interrelated structures in the visual and circadian systems. Neurosci. Biobehav. Rev. 21, 705–727. doi: 10.1016/s0149-7634(96)00019-x
Hattar, S., Liao, H. W., Takao, M., Berson, D. M., and Yau, K. W. (2002). Melanopsin-containing retinal ganglion cells: architecture, projections, and intrinsic photosensitivity. Science 295, 1065–1070. doi: 10.1126/science.1069609
Herzog, E. D., Takahashi, J. S., and Block, G. D. (1998). Clock controls circadian period in isolated suprachiasmatic nucleus neurons. Nat. Neurosci. 1, 708–713. doi: 10.1038/3708
Hiller, A. J., and Ishii, M. (2018). Disorders of body weight, sleep and circadian rhythm as manifestations of hypothalamic dysfunction in Alzheimer’s disease. Front. Cell Neurosci. 12:471. doi: 10.3389/fncel.2018.00471
Hood, S., and Amir, S. (2018). Biological clocks and rhythms of anger and aggression. Front. Behav. Neurosci. 12:4. doi: 10.3389/fnbeh.2018.00004
Hope, T., Keene, J., Gedling, K., Fairburn, C. G., and Jacoby, R. (1998). Predictors of institutionalization for people with dementia living at home with a carer. Int. J. Geriatr. Psychiatry 13, 682–690. doi: 10.1002/(sici)1099-1166(1998100)13:10<682::aid-gps847>3.0.co;2-y
Huhman, K. L., and Albers, H. E. (1994). Neuropeptide Y microinjected into the suprachiasmatic region phase shifts circadian rhythms in constant darkness. Peptides 15, 1475–1478. doi: 10.1016/0196-9781(94)90126-0
Hut, R. A., and Van der Zee, E. A. (2011). The cholinergic system, circadian rhythmicity, and time memory. Behav. Brain Res. 221, 466–480. doi: 10.1016/j.bbr.2010.11.039
Ichikawa, T., and Hirata, Y. (1986). Organization of choline acetyltransferase-containing structures in the forebrain of the rat. J. Neurosci. 6, 281–292. doi: 10.1523/jneurosci.06-01-00281.1986
Inder, M. L., Crowe, M. T., and Porter, R. (2016). Effect of transmeridian travel and jetlag on mood disorders: evidence and implications. Aust. N. Z. J. Psychiatry 50, 220–227. doi: 10.1177/0004867415598844
Ishii, M., and Iadecola, C. (2015). Metabolic and non-cognitive manifestations of Alzheimer’s disease: the hypothalamus as both culprit and target of pathology. Cell. Metab. 22, 761–776. doi: 10.1016/j.cmet.2015.08.016
Janik, D., and Mrosovsky, N. (1992). Gene expression in the geniculate induced by a nonphotic circadian phase shifting stimulus. Neuroreport 3, 575–578. doi: 10.1097/00001756-199207000-00007
Janik, D., and Mrosovsky, N. (1994). Intergeniculate leaflet lesions and behaviorally-induced shifts of circadian rhythms. Brain Res. 651, 174–182. doi: 10.1016/0006-8993(94)90695-5
Jin, X., Shearman, L. P., Weaver, D. R., Zylka, M. J., De Vries, G. J., and Reppert, S. M. (1999). A molecular mechanism regulating rhythmic output from the suprachiasmatic circadian clock. Cell 96, 57–68. doi: 10.1016/s0092-8674(00)80959-9
Kalmbach, D. A., Pillai, V., Cheng, P., Arnedt, J. T., and Drake, C. L. (2015). Shift work disorder, depression, and anxiety in the transition to rotating shifts: the role of sleep reactivity. Sleep Med. 16, 1532–1538. doi: 10.1016/j.sleep.2015.09.007
Kanno, K., Shima, S., Ishida, Y., and Yamanouchi, K. (2008). Ipsilateral and contralateral serotonergic projections from dorsal and median raphe nuclei to the forebrain in rats: immunofluorescence quantitative analysis. Neurosci. Res. 61, 207–218. doi: 10.1016/j.neures.2008.03.001
Kawamoto, K., Nagano, M., Kanda, F., Chihara, K., Shigeyoshi, Y., and Okamura, H. (2003). Two types of Vip neuronal components in rat suprachiasmatic nucleus. J. Neurosci. Res. 74, 852–857. doi: 10.1002/jnr.10751
Kawano, H., Decker, K., and Reuss, S. (1996). Is there a direct retina-raphe-suprachiasmatic nucleus pathway in the rat? Neurosci. Lett. 212, 143–146. doi: 10.1016/0304-3940(96)12795-6
Ketchesin, K. D., Becker-Krail, D., and Mcclung, C. A. (2020). Mood-related central and peripheral clocks. Eur. J. Neurosci. 51, 326–345. doi: 10.1111/ejn.14253
Khachiyants, N., Trinkle, D., Son, S. J., and Kim, K. Y. (2011). Sundown syndrome in persons with dementia: an update. Psychiatry Investig. 8, 275–287.
Kiss, J., and Halasz, B. (1996). Synaptic contacts between cholinergic afferents and suprachiasmatic neurones of the rat. Neuroreport 7, 1961–1964. doi: 10.1097/00001756-199608120-00020
Klaffke, S., and Staedt, J. (2006). Sundowning and circadian rhythm disorders in dementia. Acta Neurol. Belg. 106, 168–175.
Kotagal, V., Muller, M. L., Kaufer, D. I., Koeppe, R. A., and Bohnen, N. I. (2012). Thalamic cholinergic innervation is spared in Alzheimer disease compared to parkinsonian disorders. Neurosci. Lett. 514, 169–172. doi: 10.1016/j.neulet.2012.02.083
Kroeger, D., Ferrari, L. L., Petit, G., Mahoney, C. E., Fuller, P. M., Arrigoni, E., et al. (2017). Cholinergic, glutamatergic, and gabaergic neurons of the pedunculopontine tegmental nucleus have distinct effects on sleep/wake behavior in mice. J. Neurosci. 37, 1352–1366. doi: 10.1523/jneurosci.1405-16.2016
Kunwar, P. S., Zelikowsky, M., Remedios, R., Cai, H., Yilmaz, M., Meister, M., et al. (2015). Ventromedial hypothalamic neurons control a defensive emotion state. eLife 4:e06633.
La Morgia, C., Ross-Cisneros, F. N., Koronyo, Y., Hannibal, J., Gallassi, R., Cantalupo, G., et al. (2016). Melanopsin retinal ganglion cell loss in Alzheimer disease. Ann. Neurol. 79, 90–109. doi: 10.1002/ana.24548
Lai, M. K., Tsang, S. W., Francis, P. T., Esiri, M. M., Keene, J., Hope, T., et al. (2003). Reduced serotonin 5-Ht1A receptor binding in the temporal cortex correlates with aggressive behavior in Alzheimer disease. Brain Res. 974, 82–87. doi: 10.1016/s0006-8993(03)02554-x
Leander, P., Vrang, N., and Moller, M. (1998). Neuronal projections from the mesencephalic raphe nuclear complex to the suprachiasmatic nucleus and the deep pineal gland of the golden hamster ( Mesocricetus auratus ). J. Comp. Neurol. 399, 73–93. doi: 10.1002/(sici)1096-9861(19980914)399:1<73::aid-cne6>3.0.co;2-7
Lee, I. T., Chang, A. S., Manandhar, M., Shan, Y., Fan, J., Izumo, M., et al. (2015). Neuromedin s-producing neurons act as essential pacemakers in the suprachiasmatic nucleus to couple clock neurons and dictate circadian rhythms. Neuron 85, 1086–1102. doi: 10.1016/j.neuron.2015.02.006
Leonpacher, A. K., Peters, M. E., Drye, L. T., Makino, K. M., Newell, J. A., Devanand, D. P., et al. (2016). Effects of citalopram on neuropsychiatric symptoms in Alzheimer’s dementia: evidence from the citad study. Am. J. Psychiatry 173, 473–480. doi: 10.1176/appi.ajp.2016.15020248
Li, J. D., Hu, W. P., Boehmer, L., Cheng, M. Y., Lee, A. G., Jilek, A., et al. (2006). Attenuated circadian rhythms in mice lacking the prokineticin 2 gene. J. Neurosci. 26, 11615–11623. doi: 10.1523/jneurosci.3679-06.2006
Liguori, C., Mercuri, N. B., Nuccetelli, M., Izzi, F., Bernardini, S., and Placidi, F. (2018). Cerebrospinal fluid orexin levels and nocturnal sleep disruption in Alzheimer’s disease patients showing neuropsychiatric symptoms. J. Alzheimers Dis. 66, 993–999. doi: 10.3233/jad-180769
Lin, L., Faraco, J., Li, R., Kadotani, H., Rogers, W., Lin, X., et al. (1999). The sleep disorder canine narcolepsy is caused by a mutation in the hypocretin (orexin) receptor 2 gene. Cell 98, 365–376. doi: 10.1016/s0092-8674(00)81965-0
Lin, W. H., and Yi, C. C. (2015). Unhealthy sleep practices, conduct problems, and daytime functioning during adolescence. J. Youth Adolesc. 44, 431–446. doi: 10.1007/s10964-014-0169-9
Liu, A. K., Chang, R. C., Pearce, R. K., and Gentleman, S. M. (2015). Nucleus basalis of Meynert revisited: anatomy, history and differential involvement in Alzheimer’s and Parkinson’s disease. Acta Neuropathol. 129, 527–540. doi: 10.1007/s00401-015-1392-5
Liu, C., Ding, J. M., Faiman, L. E., and Gillette, M. U. (1997). Coupling of muscarinic cholinergic receptors and cGMP in nocturnal regulation of the suprachiasmatic circadian clock. J. Neurosci. 17, 659–666. doi: 10.1523/jneurosci.17-02-00659.1997
Liu, C., and Gillette, M. U. (1996). Cholinergic regulation of the suprachiasmatic nucleus circadian rhythm via a muscarinic mechanism at night. J. Neurosci. 16, 744–751. doi: 10.1523/jneurosci.16-02-00744.1996
Liu, C., and Reppert, S. M. (2000). GABA synchronizes clock cells within the suprachiasmatic circadian clock. Neuron 25, 123–128. doi: 10.1016/s0896-6273(00)80876-4
Liu, R. Y., Zhou, J. N., Hoogendijk, W. J., Van Heerikhuize, J., Kamphorst, W., Unmehopa, U. A., et al. (2000). Decreased vasopressin gene expression in the biological clock of Alzheimer disease patients with and without depression. J. Neuropathol. Exp. Neurol. 59, 314–322. doi: 10.1093/jnen/59.4.314
Logan, R. W., and McClung, C. A. (2019). Rhythms of life: circadian disruption and brain disorders across the lifespan. Nat. Rev. Neurosci. 20, 49–65. doi: 10.1038/s41583-018-0088-y
Lovenberg, T. W., Baron, B. M., de Lecea, L., Miller, J. D., Prosser, R. A., Rea, M. A., et al. (1993). A novel adenylyl cyclase-activating serotonin receptor (5-Ht7) implicated in the regulation of mammalian circadian rhythms. Neuron 11, 449–458. doi: 10.1016/0896-6273(93)90149-l
Lu, J., Zhang, Y. H., Chou, T. C., Gaus, S. E., Elmquist, J. K., Shiromani, P., et al. (2001). Contrasting effects of ibotenate lesions of the paraventricular nucleus and subparaventricular zone on sleep-wake cycle and temperature regulation. J. Neurosci. 21, 4864–4874. doi: 10.1523/jneurosci.21-13-04864.2001
Madeira, M. D., Pereira, P. A., Silva, S. M., Cadete-Leite, A., and Paula-Barbosa, M. M. (2004). Basal forebrain neurons modulate the synthesis and expression of neuropeptides in the rat suprachiasmatic nucleus. Neuroscience 125, 889–901. doi: 10.1016/j.neuroscience.2004.03.005
Manni, R., Cremascoli, R., Perretti, C., De Icco, R., Picascia, M., Ghezzi, C., et al. (2019). Evening melatonin timing secretion in real life conditions in patients with Alzheimer disease of mild to moderate severity. Sleep Med. 63, 122–126. doi: 10.1016/j.sleep.2019.04.018
Martin, J., Marler, M., Shochat, T., and Ancoli-Israel, S. (2000). Circadian rhythms of agitation in institutionalized patients with Alzheimer’s disease. Chronobiol. Int. 17, 405–418. doi: 10.1081/cbi-100101054
Martino-Saltzman, D., Blasch, B. B., Morris, R. D., and Mcneal, L. W. (1991). Travel behavior of nursing home residents perceived as wanderers and nonwanderers. Gerontologist 31, 666–672. doi: 10.1093/geront/31.5.666
Maywood, E. S., Reddy, A. B., Wong, G. K., O’neill, J. S., O’brien, J. A., Mcmahon, D. G., et al. (2006). Synchronization and maintenance of timekeeping in suprachiasmatic circadian clock cells by neuropeptidergic signaling. Curr. Biol. 16, 599–605. doi: 10.1016/j.cub.2006.02.023
Mega, M. S., Masterman, D. M., O’connor, S. M., Barclay, T. R., and Cummings, J. L. (1999). The spectrum of behavioral responses to cholinesterase inhibitor therapy in Alzheimer disease. Arch. Neurol. 56, 1388–1393.
Menegardo, C. S., Friggi, F. A., Scardini, J. B., Rossi, T. S., Vieira, T. D. S., Tieppo, A., et al. (2019). Sundown syndrome in patients with Alzheimer’s disease dementia. Dement. Neuropsychol. 13, 469–474.
Mesulam, M. M. (2013). Cholinergic circuitry of the human nucleus basalis and its fate in Alzheimer’s disease. J. Comp. Neurol. 521, 4124–4144. doi: 10.1002/cne.23415
Meyer-Bernstein, E. L., and Morin, L. P. (1996). Differential serotonergic innervation of the suprachiasmatic nucleus and the intergeniculate leaflet and its role in circadian rhythm modulation. J. Neurosci. 16, 2097–2111. doi: 10.1523/jneurosci.16-06-02097.1996
Mintzer, J., Faison, W., Street, J. S., Sutton, V. K., and Breier, A. (2001). Olanzapine in the treatment of anxiety symptoms due to Alzheimer’s disease: a post hoc analysis. Int. J. Geriatr. Psychiatry 16(Suppl. 1), S71–S77.
Moga, M. M., and Moore, R. Y. (1997). Organization of neural inputs to the suprachiasmatic nucleus in the rat. J. Comp. Neurol. 389, 508–534. doi: 10.1002/(sici)1096-9861(19971222)389:3<508::aid-cne11>3.0.co;2-h
Mohawk, J. A., Green, C. B., and Takahashi, J. S. (2012). Central and peripheral circadian clocks in mammals. Annu. Rev. Neurosci. 35, 445–462. doi: 10.1146/annurev-neuro-060909-153128
Moore, R. Y., and Eichler, V. B. (1972). Loss of a circadian adrenal corticosterone rhythm following suprachiasmatic lesions in the rat. Brain Res. 42, 201–206. doi: 10.1016/0006-8993(72)90054-6
Moore, R. Y., Speh, J. C., and Card, J. P. (1995). The retinohypothalamic tract originates from a distinct subset of retinal ganglion cells. J. Comp. Neurol. 352, 351–366. doi: 10.1002/cne.903520304
Moore, R. Y., Weis, R., and Moga, M. M. (2000). Efferent projections of the intergeniculate leaflet and the ventral lateral geniculate nucleus in the rat. J. Comp. Neurol. 420, 398–418. doi: 10.1002/(sici)1096-9861(20000508)420:3<398::aid-cne9>3.0.co;2-9
Moraes Wdos, S., Poyares, D. R., Guilleminault, C., Ramos, L. R., Bertolucci, P. H., and Tufik, S. (2006). The effect of donepezil on sleep and REM sleep EEG in patients with Alzheimer disease: a double-blind placebo-controlled study. Sleep 29, 199–205. doi: 10.1093/sleep/29.2.199
Mrosovsky, N. (1996). Locomotor activity and non-photic influences on circadian clocks. Biol. Rev. Camb. Philos. Soc. 71, 343–372. doi: 10.1111/j.1469-185x.1996.tb01278.x
Mufson, E. J., Mash, D. C., and Hersh, L. B. (1988). Neurofibrillary tangles in cholinergic pedunculopontine neurons in Alzheimer’s disease. Ann. Neurol. 24, 623–629. doi: 10.1002/ana.410240506
Munch, M., Schmieder, M., Bieler, K., Goldbach, R., Fuhrmann, T., Zumstein, N., et al. (2017). Bright light delights: effects of daily light exposure on emotions, restactivity cycles, sleep and melatonin secretion in severely demented patients. Curr. Alzheimer Res. 14, 1063–1075.
Musiek, E. S. (2015). Circadian clock disruption in neurodegenerative diseases: cause and effect? Front. Pharmacol. 6:29. doi: 10.3389/fphar.2015.00029
Musiek, E. S., Bhimasani, M., Zangrilli, M. A., Morris, J. C., Holtzman, D. M., and Ju, Y. S. (2018). Circadian rest-activity pattern changes in aging and preclinical Alzheimer disease. JAMA Neurol. 75, 582–590.
Musiek, E. S., and Holtzman, D. M. (2016). Mechanisms linking circadian clocks, sleep, and neurodegeneration. Science 354, 1004–1008. doi: 10.1126/science.aah4968
Nagata, T., Nakajima, S., Shinagawa, S., Plitman, E., Nakayama, K., Graff-Guerrero, A., et al. (2017). Baseline predictors of antipsychotic treatment continuation and response at week 8 in patients with Alzheimer’s disease with psychosis or aggressive symptoms: an analysis of the CATIE-AD study. J. Alzheimers Dis. 60, 263–272. doi: 10.3233/jad-170412
Nakamura, T. J., Nakamura, W., Yamazaki, S., Kudo, T., Cutler, T., Colwell, C. S., et al. (2011). Age-related decline in circadian output. J. Neurosci. 31, 10201–10205. doi: 10.1523/jneurosci.0451-11.2011
Nakamura, W., Yamazaki, S., Nakamura, T. J., Shirakawa, T., Block, G. D., and Takumi, T. (2008). In vivo monitoring of circadian timing in freely moving mice. Curr. Biol. 18, 381–385. doi: 10.1016/j.cub.2008.02.024
Nautiyal, K. M., Tanaka, K. F., Barr, M. M., Tritschler, L., Le Dantec, Y., David, D. J., et al. (2015). Distinct circuits underlie the effects of 5-Ht1B receptors on aggression and impulsivity. Neuron 86, 813–826. doi: 10.1016/j.neuron.2015.03.041
Nelson, R. J., and Zucker, I. (1981). Absence of extraocular photoreception in diurnal and nocturnal rodents exposed to direct sunlight. Comp. Biochem. Physiol. A Physiol. 69, 145–148. doi: 10.1016/0300-9629(81)90651-4
Niederkofler, V., Asher, T. E., Okaty, B. W., Rood, B. D., Narayan, A., Hwa, L. S., et al. (2016). Identification of serotonergic neuronal modules that affect aggressive behavior. Cell. Rep. 17, 1934–1949. doi: 10.1016/j.celrep.2016.10.063
Nobili, A., Latagliata, E. C., Viscomi, M. T., Cavallucci, V., Cutuli, D., Giacovazzo, G., et al. (2017). Dopamine neuronal loss contributes to memory and reward dysfunction in a model of Alzheimer’s disease. Nat. Commun. 8:14727.
Oh, A. J., Amore, G., Sultan, W., Asanad, S., Park, J. C., Romagnoli, M., et al. (2019). Pupillometry evaluation of melanopsin retinal ganglion cell function and sleep-wake activity in pre-symptomatic Alzheimer’s disease. PLoS One 14:e0226197. doi: 10.1371/journal.pone.0226197
O’Leary, P. A., Haley, W. E., and Paul, P. B. (1993). Behavioral assessment in Alzheimer’s disease: use of a 24-hr log. Psychol. Aging 8, 139–143. doi: 10.1037/0882-7974.8.2.139
Paleacu, D., Mazeh, D., Mirecki, I., Even, M., and Barak, Y. (2002). Donepezil for the treatment of behavioral symptoms in patients with Alzheimer’s disease. Clin. Neuropharmacol. 25, 313–317. doi: 10.1097/00002826-200211000-00007
Pinto, T., Lanctot, K. L., and Herrmann, N. (2011). Revisiting the cholinergic hypothesis of behavioral and psychological symptoms in dementia of the Alzheimer’s type. Ageing Res. Rev. 10, 404–412.
Pollak, C. P., and Perlick, D. (1991). Sleep problems and institutionalization of the elderly. J. Geriatr. Psychiatry Neurol. 4, 204–210. doi: 10.1177/089198879100400405
Power, A., Hughes, A. T., Samuels, R. E., and Piggins, H. D. (2010). Rhythm-promoting actions of exercise in mice with deficient neuropeptide signaling. J. Biol. Rhythms 25, 235–246. doi: 10.1177/0748730410374446
Pyun, J. M., Kang, M. J., Yun, Y., Park, Y. H., and Kim, S. (2019). Apoe varepsilon4 and Rem sleep behavior disorder as risk factors for sundown syndrome in Alzheimer’s disease. J. Alzheimers Dis. 69, 521–528. doi: 10.3233/jad-190032
Randler, C., and Vollmer, C. (2013). Aggression in young adults–a matter of short sleep and social jetlag? Psychol. Rep. 113, 754–765. doi: 10.2466/16.02.pr0.113x31z7
Reebs, S. G., and Mrosovsky, N. (1989). Effects of induced wheel running on the circadian activity rhythms of Syrian hamsters: entrainment and phase response curve. J. Biol. Rhythms 4, 39–48. doi: 10.1177/074873048900400103
Ridha, B. H., Crutch, S., Cutler, D., Frost, C., Knight, W., Barker, S., et al. (2018). A double-blind placebo-controlled cross-over clinical trial of donepezil in posterior cortical atrophy due to underlying Alzheimer’s disease: donipad study. Alzheimers Res. Ther. 10:44.
Rodriguez, J. J., Noristani, H. N., and Verkhratsky, A. (2012). The serotonergic system in ageing and Alzheimer’s disease. Prog. Neurobiol. 99, 15–41. doi: 10.1016/j.pneurobio.2012.06.010
Saper, C. B. (2013). The central circadian timing system. Curr. Opin. Neurobiol. 23, 747–751. doi: 10.1016/j.conb.2013.04.004
Scheff, S. W., Price, D. A., Schmitt, F. A., and Mufson, E. J. (2006). Hippocampal synaptic loss in early Alzheimer’s disease and mild cognitive impairment. Neurobiol. Aging 27, 1372–1384. doi: 10.1016/j.neurobiolaging.2005.09.012
Schlarb, A. A., Sopp, R., Ambiel, D., and Grunwald, J. (2014). Chronotype-related differences in childhood and adolescent aggression and antisocial behavior–a review of the literature. Chronobiol. Int. 31, 1–16. doi: 10.3109/07420528.2013.829846
Schlosser Covell, G. E., Dhawan, P. S., Lee Iannotti, J. K., Hoffman-Snyder, C. R., Wellik, K. E., Caselli, R. J., et al. (2012). Disrupted daytime activity and altered sleep-wake patterns may predict transition to mild cognitive impairment or dementia: a critically appraised topic. Neurologist 18, 426–429. doi: 10.1097/nrl.0b013e318272f7ef
Schneider, L. S., Frangakis, C., Drye, L. T., Devanand, D. P., Marano, C. M., Mintzer, J., et al. (2016). Heterogeneity of treatment response to citalopram for patients with Alzheimer’s disease with aggression or agitation: the CitAD randomized clinical trial. Am. J. Psychiatry 173, 465–472. doi: 10.1176/appi.ajp.2015.15050648
Schwartz, W. J., Gross, R. A., and Morton, M. T. (1987). The suprachiasmatic nuclei contain a tetrodotoxin-resistant circadian pacemaker. Proc. Natl. Acad. Sci. U.S.A. 84, 1694–1698. doi: 10.1073/pnas.84.6.1694
Sekaran, S., Foster, R. G., Lucas, R. J., and Hankins, M. W. (2003). Calcium imaging reveals a network of intrinsically light-sensitive inner-retinal neurons. Curr. Biol. 13, 1290–1298. doi: 10.1016/s0960-9822(03)00510-4
Shih, Y. H., Pai, M. C., Huang, Y. C., and Wang, J. J. (2017). Sundown syndrome, sleep quality, and walking among community-dwelling people with Alzheimer disease. J. Am. Med. Dir. Assoc. 18, 396–401. doi: 10.1016/j.jamda.2016.10.016
Shih, Y. H., Pai, M. C., Lin, H. S., Sung, P. S., and Wang, J. J. (2019). Effects of walking on sundown syndrome in community-dwelling people with Alzheimer’s disease. Int. J. Older People Nurs. 15:e12292.
Silva, B. A., Mattucci, C., Krzywkowski, P., Murana, E., Illarionova, A., Grinevich, V., et al. (2013). Independent hypothalamic circuits for social and predator fear. Nat. Neurosci. 16, 1731–1733. doi: 10.1038/nn.3573
Silva, M. W. B., Sousa-Munoz, R. L., Frade, H. C., Fernandes, P. A., and Magalhaes, A. O. (2017). Sundown syndrome and symptoms of anxiety and depression in hospitalized elderly. Dement. Neuropsychol. 11, 154–161. doi: 10.1590/1980-57642016dn11-020008
Silver, R., Lesauter, J., Tresco, P. A., and Lehman, M. N. (1996). A diffusible coupling signal from the transplanted suprachiasmatic nucleus controlling circadian locomotor rhythms. Nature 382, 810–813. doi: 10.1038/382810a0
Skjerve, A., and Nygaard, H. A. (2000). Improvement in sundowning in dementia with Lewy bodies after treatment with donepezil. Int. J. Geriatr. Psychiatry 15, 1147–1151. doi: 10.1002/1099-1166(200012)15:12<1147::aid-gps262>3.0.co;2-l
Sloane, P. D., Mitchell, C. M., Preisser, J. S., Phillips, C., Commander, C., and Burker, E. (1998). Environmental correlates of resident agitation in Alzheimer’s disease special care units. J. Am. Geriatr. Soc. 46, 862–869. doi: 10.1111/j.1532-5415.1998.tb02720.x
Sprouse, J., Reynolds, L., Li, X., Braselton, J., and Schmidt, A. (2004). 8-OH-DPAT as a 5-HT7 agonist: phase shifts of the circadian biological clock through increases in cAMP production. Neuropharmacology 46, 52–62. doi: 10.1016/j.neuropharm.2003.08.007
Stephan, F. K., and Zucker, I. (1972). Circadian rhythms in drinking behavior and locomotor activity of rats are eliminated by hypothalamic lesions. Proc. Natl. Acad. Sci. U.S.A. 69, 1583–1586. doi: 10.1073/pnas.69.6.1583
Swaab, D. F., Fliers, E., and Partiman, T. S. (1985). The suprachiasmatic nucleus of the human brain in relation to sex, age and senile dementia. Brain Res. 342, 37–44. doi: 10.1016/0006-8993(85)91350-2
Taylor, B. J., and Hasler, B. P. (2018). Chronotype and mental health: recent advances. Curr. Psychiatry Rep. 20:59.
Todd, W. D., Fenselau, H., Wang, J. L., Zhang, R., Machado, N. L., Venner, A., et al. (2018). A hypothalamic circuit for the circadian control of aggression. Nat. Neurosci. 21, 717–724. doi: 10.1038/s41593-018-0126-0
Todd, W. D., Gall, A. J., Weiner, J. A., and Blumberg, M. S. (2012). Distinct retinohypothalamic innervation patterns predict the developmental emergence of species-typical circadian phase preference in nocturnal Norway rats and diurnal nile grass rats. J. Comp. Neurol. 520, 3277–3292. doi: 10.1002/cne.23098
Todd, W. D., and Machado, N. L. (2019). A time to fight: Circadian control of aggression and associated autonomic support. Auton Neurosci. 217, 35–40. doi: 10.1016/j.autneu.2018.12.008
Todd, W. D., Venner, A., Anaclet, C., Broadhurst, R. Y., De Luca, R., Bandaru, S. S., et al. (2020). Suprachiasmatic VIP neurons are required for normal circadian rhythmicity and comprised of molecularly distinct subpopulations. Nat. Commun. doi: 10.1038/s41467-020-17197-2
Tortosa-Martinez, J., Clow, A., Caus-Pertegaz, N., Gonzalez-Caballero, G., Abellan-Miralles, I., and Saenz, M. J. (2015). Exercise increases the dynamics of diurnal cortisol secretion and executive function in people with amnestic mild cognitive impairment. J. Aging Phys. Act. 23, 550–558. doi: 10.1123/japa.2014-0006
Vakalopoulos, C. (2017). Alzheimer’s disease: the alternative serotonergic hypothesis of cognitive decline. J. Alzheimers Dis. 60, 859–866. doi: 10.3233/jad-170364
Venner, A., Broadhurst, R. Y., Sohn, L. T., Todd, W. D., and Fuller, P. M. (2020). Selective activation of serotoninergic dorsal raphe neurons facilitates sleep through anxiolysis. Sleep 43:zsz231.
Venner, A., Todd, W. D., Fraigne, J., Bowrey, H., Eban-Rothschild, A., Kaur, S., et al. (2019). Newly identified sleep-wake and circadian circuits as potential therapeutic targets. Sleep 42:zsz023.
Venturelli, M., Sollima, A., Ce, E., Limonta, E., Bisconti, A. V., Brasioli, A., et al. (2016). Effectiveness of exercise- and cognitive-based treatments on salivary cortisol levels and sundowning syndrome symptoms in patients with Alzheimer’s disease. J. Alzheimers Dis. 53, 1631–1640. doi: 10.3233/jad-160392
Vermeiren, Y., Van Dam, D., Aerts, T., Engelborghs, S., and De Deyn, P. P. (2014). Monoaminergic neurotransmitter alterations in postmortem brain regions of depressed and aggressive patients with Alzheimer’s disease. Neurobiol. Aging 35, 2691–2700. doi: 10.1016/j.neurobiolaging.2014.05.031
Videnovic, A., and Zee, P. C. (2015). Consequences of circadian disruption on neurologic health. Sleep Med. Clin. 10, 469–480. doi: 10.1016/j.jsmc.2015.08.004
Vogels, O. J., Broere, C. A., Ter Laak, H. J., Ten Donkelaar, H. J., Nieuwenhuys, R., and Schulte, B. P. (1990). Cell loss and shrinkage in the nucleus basalis Meynert complex in Alzheimer’s disease. Neurobiol. Aging 11, 3–13. doi: 10.1016/0197-4580(90)90056-6
Vujovic, N., Gooley, J. J., Jhou, T. C., and Saper, C. B. (2015). Projections from the subparaventricular zone define four channels of output from the circadian timing system. J. Comp. Neurol. 523, 2714–2737. doi: 10.1002/cne.23812
Wahnschaffe, A., Nowozin, C., Haedel, S., Rath, A., Appelhof, S., Munch, M., et al. (2017). Implementation of dynamic lighting in a nursing home: impact on agitation but not on rest-activity patterns. Curr. Alzheimer Res. 14, 1076–1083.
Wang, J. L., Lim, A. S., Chiang, W. Y., Hsieh, W. H., Lo, M. T., Schneider, J. A., et al. (2015). Suprachiasmatic neuron numbers and rest-activity circadian rhythms in older humans. Ann. Neurol. 78, 317–322. doi: 10.1002/ana.24432
Watts, A. G., and Swanson, L. W. (1987). Efferent projections of the suprachiasmatic nucleus: II. Studies using retrograde transport of fluorescent dyes and simultaneous peptide immunohistochemistry in the rat. J. Comp. Neurol. 258, 230–252. doi: 10.1002/cne.902580205
Watts, A. G., Swanson, L. W., and Sanchez-Watts, G. (1987). Efferent projections of the suprachiasmatic nucleus: I. Studies using anterograde transport of Phaseolus vulgaris leucoagglutinin in the rat. J. Comp. Neurol. 258, 204–229. doi: 10.1002/cne.902580204
Welsh, D. K., Logothetis, D. E., Meister, M., and Reppert, S. M. (1995). Individual neurons dissociated from rat suprachiasmatic nucleus express independently phased circadian firing rhythms. Neuron 14, 697–706. doi: 10.1016/0896-6273(95)90214-7
Yamakawa, G. R., and Antle, M. C. (2010). Phenotype and function of raphe projections to the suprachiasmatic nucleus. Eur. J. Neurosci. 31, 1974–1983. doi: 10.1111/j.1460-9568.2010.07228.x
Yesavage, J. A., Friedman, L., Ancoli-Israel, S., Bliwise, D., Singer, C., Vitiello, M. V., et al. (2003). Development of diagnostic criteria for defining sleep disturbance in Alzheimer’s disease. J. Geriatr. Psychiatry Neurol. 16, 131–139. doi: 10.1177/0891988703255684
Keywords : circadian, aggression, sundowning, Alzheimer’s disease, dementia, agitation
Citation: Todd WD (2020) Potential Pathways for Circadian Dysfunction and Sundowning-Related Behavioral Aggression in Alzheimer’s Disease and Related Dementias. Front. Neurosci. 14:910. doi: 10.3389/fnins.2020.00910
Received: 15 May 2020; Accepted: 06 August 2020; Published: 03 September 2020.
Reviewed by:
Copyright © 2020 Todd. This is an open-access article distributed under the terms of the Creative Commons Attribution License (CC BY) . The use, distribution or reproduction in other forums is permitted, provided the original author(s) and the copyright owner(s) are credited and that the original publication in this journal is cited, in accordance with accepted academic practice. No use, distribution or reproduction is permitted which does not comply with these terms.
*Correspondence: William D. Todd, [email protected]
Disclaimer: All claims expressed in this article are solely those of the authors and do not necessarily represent those of their affiliated organizations, or those of the publisher, the editors and the reviewers. Any product that may be evaluated in this article or claim that may be made by its manufacturer is not guaranteed or endorsed by the publisher.
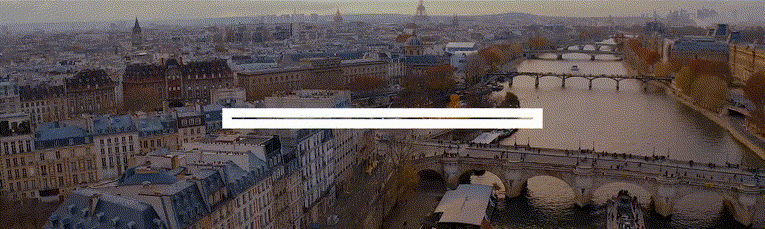
Nocturnal Frontal Lobe Epilepsy
- Sleep (M Thorpy, M Billiard, Section Editors)
- Published: 07 January 2014
- Volume 14 , article number 424 , ( 2014 )
Cite this article
- Lino Nobili 1 , 2 , 3 ,
- Paola Proserpio 1 , 2 ,
- Romina Combi 4 ,
- Federica Provini 5 , 6 ,
- Giuseppe Plazzi 5 , 6 ,
- Francesca Bisulli 5 , 6 ,
- Laura Tassi 1 &
- Paolo Tinuper 5 , 6
12k Accesses
58 Citations
12 Altmetric
Explore all metrics
Nocturnal frontal lobe epilepsy (NFLE) is a syndrome of heterogeneous etiology, characterized by the occurrence of sleep-related seizures with different complexity and duration. Genetic, lesional, and cryptogenetic NFLE forms have been described. NFLE is generally considered a benign clinical entity, although severe, drug-resistant forms do exist. A significant proportion of sleep-related complex motor seizures, hardly distinguishable from NFLE, originate outside the frontal lobe. Moreover, the distinction of NFLE from the non-rapid eye movement arousal parasomnias may be challenging. A correct diagnosis of NFLE should be based on a diagnostic approach that includes the anamnestic, video–polysomnographic, morphological, and genetic aspects. Studies on the relationships between genes, arousal regulatory mechanisms, and epileptogenesis, using both clinical and experimental models of NFLE might provide key insights in the interrelationship between sleep and epilepsy.
Similar content being viewed by others
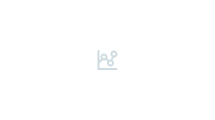
Genetic syndromes associated with frontal lobe epilepsy
Cassie Fehr & Karl Martin Klein
Sleep in Seizure Disorders
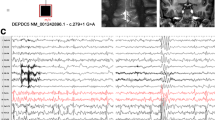
Sleep related hyper motor epilepsy (SHE): a unique syndrome with heterogeneous genetic etiologies
Francesca Bisulli, Laura Licchetta & Paolo Tinuper
Avoid common mistakes on your manuscript.
Introduction
Seizures originating from the frontal lobe mainly occur during sleep [ 1 , 2 ]. The definition of nocturnal frontal lobe epilepsy (NFLE) has been introduced in order to identify patients affected by frontal lobe epilepsy whose seizures are almost exclusively sleep related. In the last few decades, the spread and refinement of video–polysomnographic (V-PSG) technology has allowed NFLE to be fully defined and described, so that it is now considered a distinct epileptic syndrome; nevertheless, some NFLE case can be hardly distinguishable from non-rapid eye movement (NREM) parasomnias [ 3 – 7 ] and from sleep-related epileptic seizures originating outside the frontal lobe [ 8 – 10 ].
It is now recognized that NFLE is not a homogeneous disease as familial, idiopathic, sporadic, cryptogenetic, or symptomatic forms do exist [ 4 , 11 – 13 ]. In addition, a genetic heterogeneity is also evident within the familial type [ 14 ].
NFLE is usually considered a benign clinical epileptic syndrome because seizures occur almost exclusively during sleep, and in the majority of patients the pharmacological treatment is effective; however, severe and drug-resistant forms, occasionally associated with mental retardation, have been described [ 12 , 13 , 15 , 16 ••]. All the aforementioned issues will be reviewed and discussed in the following sections in order to give a comprehensive overview of the pathophysiological and clinical aspects of NFLE.
Epidemiology of NFLE
No epidemiologic data are available on NFLE prevalence. The frequency of pure sleep-related seizures, defined as “seizures occurring exclusively or predominantly (>90 %) from sleep” clusters around 12 % of people with epilepsy [ 17 , 18 ], the majority of patients being affected by focal epilepsy [ 19 , 20 ]. NFLE is probably not rare, accounting for 13 % of polysomnographic recordings for nocturnal motor disorders in a retrospective study performed at a tertiary centre in Italy [ 12 ] and for 6.3 % of a large series of focal drug-resistant epileptic patients (unpublished data). Because many cases of NFLE are misdiagnosed as parasomnias, the clinical relevance of NFLE is underestimated, especially in children [ 4 ].
Genetic Forms of NFLE
A family history for epilepsy is frequently observed in NFLE patients [ 12 ]. After the report of several families showing a clear autosomal dominant inheritance, autosomal dominant NFLE (ADNFLE) was introduced as a distinct clinical entity with marked intrafamilial variability in disease severity [ 4 , 11 , 21 ]. The genetic origin of the disease was confirmed by a linkage study in Australian kindred. More specifically, a locus was identified at chromosome 20q13.2-q13.3 [ 22 ] and two different mutations were detected in the gene coding the α4 subunit of the neuronal nicotinic acetylcholine receptor (nAChR) (CHRNA4) [ 23 , 24 ]. Both mutations were shown to have major effects on receptor function in vitro [ 23 – 26 ]. With the identification of these mutations, subsequently confirmed in families of different origins [ 27 – 29 ], ADNFLE became the first epilepsy in which genetic bases were detected, apparently showing features of a monogenic disease. Three de novo or inherited CHRNA4 mutations, occasionally associated with mild-to-moderate mental retardation were later reported [ 15 , 30 – 37 ]. ADNFLE was quickly recognized as a genetically heterogeneous disorder as most of the described families did not show mutations in the CHRNA4 gene [ 14 , 38 ], and new loci and genes were reported in the following years. In particular, a second locus was identified at chromosome 15q24 in one family [ 38 ] and a third locus spanning the pericentromeric region of chromosome 1 was identified in an Italian ADNFLE family [ 39 ], the latter containing the gene coding for the β2 subunit of the nACh receptor (CHRNB2) (Table 1 ) [ 40 ]. Further CHRNB2 mutations were disclosed in different unrelated families and one sporadic case [ 41 – 46 ] (Table 1 ); in two families a coexistence of both epilepsy and specific deficits in tasks of verbal memory was observed [ 44 , 45 ]; in two patients ictal single-photon emission computed tomography showed a significant hyperperfusion of the cingulated gyrus [ 45 ].
A fourth locus was mapped on chromosome 8p21.2-8q12 in 2006 in an Italian family and a mutation was identified in the α2 subunit of the nAChR ( CHRNA2 ) gene (Table 1 ). These patients exhibited prominent fear and organized motor behaviors, which led the authors to speculate that seizures could have a fronto-limbic involvement. In vitro studies confirmed functional alterations of the mutated protein, and postmortem brain examination disclosed a high expression of CHRNA2 in thalamic structures [ 51 ]. To date, this is the only reported ADNFLE kindred carrying CHRNA2 mutations.
The findings of a genetic alteration of the cholinergic system and the in vivo documentation [ 52 ] of an alteration of nAChR density distribution in ADNFLE patients may give some insights into understanding the relationships between genes, epileptogenesis, and arousal regulatory processes. Indeed, the nAChR are extensively distributed in the brain and contribute to the regulation of sleep and arousal oscillations, at both the cortical and subcortical level [ 53 ]. Moreover, they play a role in the release of other neurotransmitters such as gamma-aminobutyric acid (GABA) and glutamate. In animal models, it has been shown that a mutation of the nicotinic receptors may create an unbalanced excitation/inhibition circuitry within the GABAergic reticular thalamic neurons, thus favouring seizures through the synchronizing effect of spontaneous thalamocortical oscillations [ 54 ]. However, other experimental studies have reported an involvement of nAChR in the regulation of arousals, sleep stability, and the activity–rest pattern [ 53 , 55 •]. We can speculate that the observed genetic alterations in NFLE create the conditions for both arousal instability and seizure generation. Indeed, it is recognized that NFLE patients may have a high number of arousals and that nocturnal motor events tend to occur during unstable sleep [ 56 , 57 , 58 •]. With these assumptions in mind, we can also hypothesize that the high prevalence of parasomnias in the personal and family history of NFLE patients [ 59 ••] relies on a common alteration of the arousal regulatory system.
However, ADNFLE is not only related to mutations in the cholinergic system. Since 2005, the involvement of other genes, not belonging to the nACh receptor subunit family, has been recognized in the ADNFLE pathogenesis. In particular, Combi et al. [ 47 ] found two new putative ADNFLE loci on chromosomes 3p22-p24 and 8q11.2-q21.1; among the genes mapping in these regions, the one most probably involved in ADNFLE pathogenesis is corticotropin-releasing hormone (CRH) on chromosome 8. A gene sequencing procedure disclosed two nucleotide variations (Table 1 ) in the CRH promoter region in four families and in two sporadic cases [ 48 ]. An additional family was later detected, with a patient carrying both variations in compound heterozygosity [ 49 ]. The in vitro functional analysis of both variations demonstrated an altered level of protein expression [ 48 ] suggesting an interrelation between CRH concentration and neuronal excitability with a possible effect on thalamo-cortical loop dysfunctions [ 47 , 48 ]. Very recently, a further CRH mutation has been found in the protein pro-sequence region of the CRH of two affected siblings of an Italian ADNFLE family [ 50 •]. The recurrence of variants causing altered levels of the CRH secretion suggests that individuals with such an altered hormone level could be more prone to develop ADNFLE [ 50 •].
In 2012, a further gene on chromosome 9 encoding the sodium-activated potassium channel subunit 1 (KCNT1) was associated with ADNFLE. In particular, four variants in KCNT1 were identified in three families and in a sporadic ADNFLE case (Table 1 ), all showing a severe ADNFLE phenotype with early onset, high prevalence of intellectual disabilities, and psychiatric or behavioral problems, including psychosis, catatonia, and aggression. Contrary to all the previously found mutations, KCNT1 mutations showed complete penetrance [ 16 ••]. Very recently, mutations in the DEPDC5 gene were reported as responsible for different types of focal epilepsies, including ADNFLE [ 60 ••]. However, the prevalence of mutations in this gene remains to be assessed.
In summary, ADNFLE is a heterogeneous genetic syndrome that can be incidental to mutations in different genes; to date, mutations have been reported in six genes (Table 1 ). Mutations in these genes, however, account only for a minority of cases [ 61 ] and their mean penetrance ranges from 60 % to 80 %. Hence, further studies are needed to better characterize this heterogeneous syndrome. Given the high intrafamilial variability and the overlapping features of the clinical manifestations, ADNFLE patients do not show a clear distinction from sporadic NFLE cases, except for certain ADNFLE mutations frequently associated with specific additional neurological or psychiatric symptoms (Table 1 ). Hence, neuropsychological testing and psychiatric assessment are mandatory in ADNFLE patients [ 62 •].
The attempt to define a genotype–phenotype correlation is hampered by the rarity of the disease and by difference in study designs. Available data suggest that no single feature can indicate one particular form and that no correlation between functional effects of the mutations and the severity of the disease exists [ 29 , 62 •].
Symptomatic Forms of NFLE
Studies, conducted in patients with drug-resistant epilepsy, have shown that type II focal cortical dysplasia (type II FCD; Taylor-type cortical dysplasia) [ 63 ] is the most frequent etiological substrate of NFLE [ 64 •, 65 •, 66 , 67 ]. However, although type II FCD is more frequently found within the frontal lobe, it has been shown that, regardless of its anatomical localization, type II FCD increases the risk of sleep-related epilepsy, in particular the type IIb [ 64 •, 68 ]. Type IIb FCD is characterized by the presence of dysmorphic neurons, connected through an abnormal GABAergic circuit, and the so called “balloon cells” (BC). BC are not directly involved in the genesis of paroxysmal discharges, but seem to act as “bridges” between different areas of clustered neuronal hyperexcitability, enabling rapid propagation of electrical activity via their particular rearranged gap junctions [ 69 ]. During wakefulness, type IIb FCD shows a peculiar pattern of interictal activity that is characterized by the occurrence of rhythmic and subcontinuous spike- and polyspike waves with a frequency usually between 1 and 3 Hz, alternating with short bursts of fast discharges interrupted by suppression of activity. These burst of fast discharges are relatively rare and sporadic during wakefulness, but become numerous during slow wave sleep, when they tend to recur pseudo-periodically [ 65 •, 68 , 70 , 71 ] and often spread over surrounding nonlesional areas and develop into a seizure [ 65 •, 68 ]. Type IIb FCD seems to be an interesting “in vivo” model to study the relationship between sleep mechanisms and epileptogenesis, as well as a peculiar “in vitro” model for pharmacological studies.
Clinical Features of NFLE
ADNFLE and sporadic NFLE show similar clinical and electroencephalographic features [ 11 – 13 , 21 ]. In the high majority of NFLE patients, seizures begin before the age of 20 years, with a peak during childhood [ 4 , 11 – 13 , 21 ], although onset during adulthood has been also reported [ 11 , 12 , 21 ]. Seizure frequency is usually high, and patients generally experience many seizures a night [ 12 , 13 ], although the frequency may diminish during adulthood [ 11 , 21 ]. Of note, occasional seizures during wakefulness can occur [ 12 , 13 ]. Subjective seizure manifestations, uncommon during the night, have been reported by patients during these diurnal events; however, auras generally have a poor localizing and lateralizing value [ 13 , 72 ].
NFLE patients may show different sleep-related motor events of increasing complexity and duration, even during a single night. These include 1) short-lasting (2–4 s) stereotyped movements involving the limbs, the axial musculature, and/or the head [ 11 – 13 , 58 •, 73 ]; 2) paroxysmal arousals (PAs), characterized by sudden and brief arousals (5–10 s in duration) sometimes accompanied by stereotyped movements, vocalization, frightened expression, and fear [ 11 – 13 , 74 ]; and 3) major attacks, lasting 20–30 s, characterized by asymmetric tonic or dystonic posturing, or complex movements such as pelvic thrusting, pedalling, choreo-athetoid, and ballistic movements of the limbs [ 11 – 13 , 21 ]. Some patients may show ictal deambulatory behaviors often associated with frightened expression and fear [ 12 , 13 , 51 , 75 ]. Owing to the recurrence of nocturnal motor events, several NFLE patients may complain of nonrestorative sleep and of daytime sleepiness [ 12 , 13 , 71 , 74 , 76 ].
The increasing complexity of NFLE ictal motor behaviors, from minor to major events, reflects a different duration and propagation of the discharge within the frontal lobe [ 77 , 78 ]. It has been shown that the seizure onset in patients with asymmetric tonic or dystonic posturing is generally localized in the posterior portion of the frontal cingulated gyrus and in the posterior mesial frontal cortex with a primary involvement of the supplementary motor area [ 13 , 65 •, 79 , 80 ]. In patients with seizures characterized by hyperkinetic automatisms and complex motor behaviors, the region of seizure onset may involve the dorso-lateral and anterior frontal regions (fronto-polar and frontal antero-mesial regions) [ 13 , 65 •, 80 ]; in particular, the epileptic manifestations characterized by the association of fear and more organized motor behaviors (the so-called “epileptic nocturnal wandering”) seem to correspond to the activation of anterior cingulated, orbito-polar, and temporal regions (Fig. 1 ) [ 10 , 13 , 51 , 81 ]. Some of these clinical manifestations have been interpreted as a release of inhibition (provoked by the seizure) on the innate behavioral automatisms and survival behaviors, programmed in cortical and subcortical central pattern generators [ 82 , 83 ].
Video-electroencephalography recordings of an episode of epileptic nocturnal wandering. Magnetic resonance imaging shows the presence of a focal cortical dysplasia in the left orbital region (arrows). Postsurgical histopathologic examination revealed the presence of a type II focal cortical dysplasia (Taylor-type cortical dysplasia)
The interictal and ictal scalp electroencephalography (EEG) features of NFLE patients are often uninformative, especially in cases of seizures originating from the mesial frontal cortex [ 12 , 78 ]. However, prolonged video–EEG recordings can sometimes be useful in characterizing the EEG abnormalities in selected subgroups of patients undergoing presurgical investigation [ 13 ]. Unfortunately, the reduced contribution of scalp EEG to localization and lateralization of the seizure onset zone is accompanied by a low incidence of positive findings using magnetic resonance imaging [ 12 , 13 ].
In recent years it has been shown that sleep-related complex motor attacks, similar to those occurring in NFLE patients, may also originate from the temporal lobe [ 8 , 71 , 72 , 81 ], the insular-opercular region [ 9 , 65 •, 84 ••, 85 , 86 ], and the posterior cerebral regions [ 65 •]. A careful analysis of aura features may be particularly useful in the differential diagnosis of NFLE. Indeed, these patients may report subjective manifestations that could suggest an origin of seizure outside the frontal lobe, such as epigastric, acoustic sensations, and/or déjà vu in temporal lobe onset [ 72 ]; laryngeal and throat sensations, dysarthria, hypersalivation, unpleasant or electrical paresthesiae, diffuse or restricted to a small cutaneous area, in opercular, and/or insular onset [ 9 , 65 •, 84 ••, 87 ]; and visual hallucinations in occipital seizures [ 65 •].
Differential Diagnosis
Many of the differences between NFLE and non-epileptic sleep-related events have now been clarified, and a number of clinical features have been categorized to help physicians in the differential diagnosis (Table 2 ). For instance, episodes of sleep terror and sleepwalking are isolated and rare (one episode every 1–4 months), without any stereotypic complex motor patterns such as dystonic posturing, ballistic movements, tremor, OR choreoathetosis, and tend to disappear with time. REM sleep behavior disorders (RBD) can be readily differentiated from NFLE. RBD affects predominantly elderly men (around 60 years of age), and episodes prevail in the second half of the night, unlike arousal disorders and epileptic nocturnal wandering. RBD are characterized by polymorphic behavior consistent with aggressive dream content associated with the typical polygraphic finding of REM sleep without atonia. Nocturnal panic attacks are also characterized by a sudden awakening from sleep with dramatic autonomic activation and a sensation of imminent death, but these episodes often recur only once per night and are prolonged (many minutes) [ 89 ].
Nevertheless, the differential diagnosis may remain a challenge for clinicians, and some controversial points are still a matter of debate. First, the distinction between the different disorders is complicated by the similarity of behavioral patterns encountered in non-REM parasomnias, RBD, and nocturnal frontal lobe seizures (NFLS), and by the possible coexistence of these sleep disturbances in the same individual [ 12 ]. Second, there is no gold standard objective test for parasomnias or NFLE. Finally, the diagnostic potential of the conventional work flow—clinical assessment (history taking) followed by laboratory investigations, such as interictal EEG and nocturnal V-PSG—is still hampered by the lack of internationally accepted diagnostic criteria [ 7 , 90 ].
Distinguishing NFLE from non-epileptic sleep-related events, namely arousal disorders, may be challenging and sometimes impossible on the basis of history-taking alone [ 7 , 88 ]. A reliable semeiological description of motor events occurring during the night is often difficult to collect from a witness or sleep partner because observers may be lacking or, if present, not fully reliable or awake when attacks occur. Moreover, subjective semeiological elements are often absent in all types of motor events during sleep. To date, the available clinical diagnostic tools for nocturnal motor episodes, that is, the standard criteria, are unreliable, as in the case of several parasomnias [ 91 ], or are still lacking, as in the case of NFLE. Recent efforts to obtain a systematic assessment of the diagnostic reliability of clinical history have devised two instruments: the Frontal Lobe Epilepsy and Parasomnias scale [ 92 ] and the Structured Interview for NFLE [ 93 •]. Albeit clinically useful, these tools are limited by contradictory diagnostic accuracy [ 7 , 94 ].
Interictal EEGs fail to disclose epileptiform abnormalities in a substantial percentage of NFLE patients [ 12 ], whereas interictal epileptiform discharges may also occur in some patients with parasomnias, especially those with RBD (up to 26 %) [ 95 ]. Sleep V-PSG is unanimously considered the “gold-standard” diagnostic test, but it is expensive, with a limited availability, and does not always capture the event in a single-night recording. Moreover, even when the nocturnal episode has been recorded, the diagnosis remains doubtful because ictal scalp EEG fails to disclose epileptiform abnormalities or because the episode captured is a minor motor event, like the so-called PA, for which the diagnosis is not reliable, even among experts [ 6 , 7 ]. To make video analysis of nocturnal paroxysmal events more reliable a diagnostic algorithm focusing on the semeiological features of the arousal parasomnias and NFLS has recently been proposed [ 96 ••].
Despite the limits of V-PSG, the possibility of analyzing the video of the nocturnal attack remains an important diagnostic tool, making home video recording a useful adjunct when episodes are infrequent, even if the onset of the episode is missed [ 88 , 96 ••, 97 ]. Indeed, it has been shown that while the behavioral aspects characterizing the onset of a paroxysmal episode may be similar in NFLE and NREM parasomnias, the analysis of the evolution and the offset of the events are more critical for discriminating between parasomnias and NFLE seizures [ 96 ••]. However, although a home-made video can be very helpful in the diagnosis of major episodes (hypermotor seizures), the lack of reliable semeiological criteria for PAs and other minor motor events diminishes its diagnostic use for less complex brief attacks [ 6 , 73 , 96 ••, 97 ]. However, in patients with a high clinical suspicion of NFLE, the presence of a high number of sleep-related minor motor events might be interpreted as an indirect marker of epileptiform activity [ 73 , 98 ].
Response to therapy is not an ideal diagnostic tool, but may contribute to the diagnosis, especially when V-PSG is impracticable or has proven unhelpful. Unfortunately, even the response to antiepileptic drugs does not discriminate between NFLE and parasomnias. Although carbamazepine is effective at low doses in most NFLE patients, this treatment did not modify seizure frequency at all in 32 % of patients [ 12 ]. Moreover, long-term follow-up data are lacking for both parasomnias and NFLE.
In conclusion, no single, valid, and reliable diagnostic procedure (including V-PSG) or diagnostic algorithm has been defined for parasomnias and NFLE. We need to improve the sensitivity of the available diagnostic tools, studying the use of home-made videos before or instead of hospitalization and V-PSG. It is also important to devise common criteria to develop a single classification of motor events occurring during sleep and thereby make VPSG more reliable. Finally, we need to improve our knowledge on the long-term follow-up of sleep disorders, particularly when a definite diagnosis (i.e., epileptic or non-epileptic) has not been established. An international consensus on these issues is therefore advisable [ 7 ].
Many and early reports documented carbamazepine as the drug of choice in NFLE/ADNFLE patients [ 11 , 12 , 39 , 99 ], and basic studies of ADNFLE mutations suggested that mutated nAChR receptors demonstrate an increased sensitivity to carbamazepine [ 100 ].
According to the literature, about two thirds of NFLE/ADNFLE patients benefit from carbamazepine administration. This drug is usually given at low doses (200–1,000 mg at bedtime), abolishing seizures in ~20 % of cases, and giving significant relief (at least 50 % seizure reduction) in another 48 %. One third of patients are drug-resistant and most of these patients have many seizures per month [ 12 , 101 ].
Oxcarbamazepine (at a mean dose of 30.4 ±11.7 mg/kg/day) fully controlled nocturnal seizures in a single study on eight children between the age of 4 and 16 years with NFLE (some of whom were refractory to previous antiepileptic medications) [ 102 ].
Topiramate administered as single or add-on therapy from 50 to 300 mg daily at bedtime was found to be effective in about 90 % of cases in a small series of 24 NFLE patients. In the follow-up, ranging from 6 months to 6 years, the authors found 25 % of patients to be seizure-free and 62.5 % with a reduction in seizures of at least 50 % [ 103 ].
Varadkar et al. [ 104 ] reported a reduction or complete control of nocturnal seizures in three members of an ADNFLE family with acetazolamide, 500 mg at night, as add-on therapy to carbamazepine.
Treatment with nicotine transdermal patches had a beneficial effect on seizure frequency in a single patient with ADNFLE, whose seizures were refractory to standard antiepileptic drugs [ 105 ]. The beneficial effect of nicotine on seizure frequency was later reported in 9 out of 22 patients from two Norwegian ADNFLE pedigrees with CHRNA4 mutations [ 106 ], supporting the role of a nicotine defect in the arousal pathway in NFLE/ADNFLE patients. Recently, in a case–control family study, Naldi et al. [ 107 ] failed to find any significant difference in the distribution of tobacco use among NFLE patients and their relatives compared to that in the control group.
Surgical treatment may provide excellent results for selected drug-resistant NFLE patients both for seizures and for epilepsy-related sleep disturbances, such as fragmented sleep and excessive daytime somnolence [ 13 , 71 ]. An in-depth pre-surgical evaluation, often including invasive recording by stereo EEG, is mandatory for resective surgery [ 13 ]. In a population of 21 drug-resistant NFLE patients, after at least 12 months following surgery, 76 % of patients were completely seizure-free and the remainder reported significant improvements in seizure frequency and/or intensity [ 13 ]. Surgical treatment can also be effective in patients with sleep-related complex motor seizures with an extrafrontal origin [ 8 , 10 , 65 •, 72 , 84 ••, 85 , 86 ].
In summary, the interpretation of NFLE as relatively benign clinical entity, because seizures occur only or predominantly during sleep, is not completely true. Some patients suffering from rare and brief attacks tolerate them well and choose not to undergo therapy because they do not feel incapacitated by the seizures. However, a third of NFLE/ADNFLE patients are drug-resistant, and also in patients responding to treatment, withdrawal of the antiepileptic therapy is often followed by the reappearance of the seizures [ 101 ]. Finally, discrepancies between seizure outcome reported by patient and bed partner and V-PSG recordings can be found. The subjective effectiveness of antiepileptic treatment could be mostly related to the partial reduction of the longer and more complex seizures, opening the debate to the identification of clinical and sleep measures, which can better define a more objective response to treatment [ 108 •].
Conclusions
NFLE is a syndrome of heterogeneous etiology characterized by the occurrence of sleep-related seizures with various motor and affective clinical manifestations, depending on the involved neuronal networks within the frontal lobe. Moreover, a significant proportion of sleep-related complex motor seizures have an extra-frontal origin, predominantly temporal and insular. NFLE is generally considered a benign clinical entity, although severe, drug-resistant forms do exist. Comprehensive clinical and instrumental investigations have enabled us to shed light on the wide spectrum of paroxysmal events occurring during sleep; however, in a small percentage of cases, the differential diagnosis between NFLE and NREM parasomnias can still be challenging. It is therefore imperative to adopt a comprehensive diagnostic approach that includes the anamnestic, V-PSG, morphologic, and genetic aspects. Finally, we believe that further studies on the relationships between genes, arousal regulatory mechanisms, and epileptogenesis using both clinical and experimental models of NFLE could provide key insight in the interrelationship between sleep and epilepsy.
Papers of particular interest, published recently, have been highlighted as: • Of importance •• Of major importance
Crespel A, Baldy-Moulinier M, Coubes P. The relationship between sleep and epilepsy in frontal and temporal lobe epilepsies: practical and physiopathologic considerations. Epilepsia. 1998;39:150–7.
CAS PubMed Google Scholar
Herman ST, Walczak TS, Bazil CW. Distribution of partial seizures during the sleep–wake cycle: differences by seizure onset site. Neurology. 2001;56:1453–9.
Zucconi M, Ferini-Strambi L. NREM parasomnias: arousal disorders and differentiation from nocturnal frontal lobe epilepsy. Clin Neurophysiol. 2000;111 Suppl 2:S129–35.
PubMed Google Scholar
Scheffer IE, Bhatia KP, Lopes-Cendes I, Fish DR, Marsden CD, Andermann F, et al. Autosomal dominant frontal epilepsy misdiagnosed as sleep disorder. Lancet. 1994;343:515–7.
Zucconi M, Oldani A, Ferini-Strambi L, Bizzozero D, Smirne S. Nocturnal paroxysmal arousals with motor behaviors during sleep: frontal lobe epilepsy or parasomnia? Clin Neurophysiol. 1997;14:513–22.
CAS Google Scholar
Vignatelli L, Bisulli F, Provini F, Naldi I, Pittau F, Zaniboni A. Interobserver reliability of video recording in the diagnosis of nocturnal frontal lobe seizures. Epilepsia. 2007;48:1506–11.
Bisulli F, Vignatelli L, Provini F, Lugaresi E, Tinuper P. Parasomnias and nocturnal frontal lobe epilepsy (NFLE): lights and shadows – controversial points in the differential diagnosis. Sleep Med. 2011;12 Suppl 2:S27–32.
Nobili L, Cossu M, Mai R, Tassi L, Cardinale F, Castana L, et al. Sleep-related hyperkinetic seizures of temporal lobe origin. Neurology. 2004;62:482–5.
Ryvlin P, Minotti L, Demarquay G, Hirsch E, Arzimanoglou A, Hoffman D, et al. Nocturnal hypermotor seizures, suggesting frontal lobe epilepsy, can originate in the insula. Epilepsia. 2006;47:755–65.
Nobili L, Francione S, Cardinale F, Lo RG. Epileptic nocturnal wanderings with a temporal lobe origin: a stereoelectroencephalographic study. Sleep. 2002;25:669–71.
Oldani A, Zucconi M, Asselta R, Modugno M, Bonati MT, Dalpra L, et al. Autosomal dominant nocturnal frontal lobe epilepsy. A video-polysomnographic and genetic appraisal of 40 patients and delineation of the epileptic syndrome. Brain. 1998;121:205–23.
Provini F, Plazzi G, Tinuper P, Vandi S, Lugaresi E, Montagna P. Nocturnal frontal lobe epilepsy: a clinical and polygraphic overview of 100 consecutive cases. Brain. 1999;122:1017–31.
Nobili L, Francione S, Mai R, Cardinale F, Castana L, Tassi L, et al. Surgical treatment of drug-resistant nocturnal frontal lobe epilepsy. Brain. 2007;130:561–73.
De Marco EV, Gambardella A, Annesi F, Labate A, Carrideo S, Forabosco P, et al. Further evidence of genetic heterogeneity in families with autosomal dominant nocturnal frontal lobe epilepsy. Epilepsy Res. 2007;74:70–3.
Cho YW, Motamedi GK, Laufenberg I, Sohn SI, Lim JG, Lee H, et al. A Korean kindred with autosomal dominant nocturnal frontal lobe epilepsy and mental retardation. Arch Neurol. 2003;60:1625–32.
Heron SE, Smith KR, Bahlo M, Nobili L, Kahana E, Licchetta L, et al. Missense mutations in the sodium-gated potassium channel gene KCNT1 cause severe autosomal dominant nocturnal frontal lobe epilepsy. Nat Genet. 2012;44:1188–90. This study identified a new gene, encoding a sodium-gated potassium channel subunit, causing severe autosomal dominant nocturnal frontal lobe epilepsy. The mutations were identified in families and in a sporadic case. Patients with this mutation were not only affected by severe nocturnal focal seizures, but also showed intellectual and or psychiatric disability. The identification of this new gene has important implications for genetic counselling and for the understanding of the spectrum of epilepsy disorders. .
Thomas RH, King WH, Johnston JA, Smith PE. Awake seizures after pure sleep-related epilepsy: a systematic review and implications for driving law. J Neurol Neurosurg Psychiatry. 2010;81:130–5.
Derry CP, Duncan S. Sleep and epilepsy. Epilepsy Behav. 2013;26:394–404.
Yaqub BA, Waheed G, Kabiraj MM. Nocturnal epilepsies in adults. Seizure. 1997;6:145–9.
Fernandez LB, Salas-Puig J. Pure sleep seizures: risk of seizures while awake. Epileptic Disord. 2007;9:65–70.
Scheffer IE, Bhatia KP, Lopes-Cendes I, Fish DR, Marsden CD, Andermann E, et al. Autosomal dominant nocturnal frontal lobe epilepsy. A distinctive clinical disorder. Brain. 1995;118:61–73.
Phillips HA, Scheffer IE, Berkovic SF, Hollway GE, Sutherland GR, Mulley JC. Localization of a gene for autosomal dominant nocturnal frontal lobe epilepsy to chromosome 20q 13.2. Nat Genet. 1995;10:117–8.
Steinlein OK, Mulley JC, Propping P, Wallace RH, Phillips HA, Sutherland GR, et al. A missense mutation in the neuronal nicotinic acetylcholine receptor alpha 4 subunit is associated with autosomal dominant nocturnal frontal lobe epilepsy. Nat Genet. 1995;11:201–3.
Steinlein OK, Magnusson A, Stoodt J, Bertrand S, Weiland S, Berkovic SF, et al. An insertion mutation of the CHRNA4 gene in a family with autosomal dominant nocturnal frontal lobe epilepsy. Hum Mol Genet. 1997;6:943–7.
Kuryatov A, Gerzanich V, Nelson M, Olale F, Lindstrom J. Mutation causing autosomal dominant nocturnal frontal lobe epilepsy alters Ca2+ permeability, conductance, and gating of human alpha4beta2 nicotinic acetylcholine receptors. J Neurosci. 1997;17:9035–47.
Weiland S, Witzemann V, Villarroel A, Propping P, Steinlein O. An amino acid exchange in the second transmembrane segment of a neuronal nicotinic receptor causes partial epilepsy by altering its desensitization kinetics. FEBS Lett. 1996;398:91–6.
Saenz A, Galan J, Caloustian C, Lorenzo F, Marquez C, Rodriguez N, et al. Autosomal dominant nocturnal frontal lobe epilepsy in a Spanish family with a Ser252Phe mutation in the CHRNA4 gene. Arch Neurol. 1999;56:1004–9.
Steinlein OK, Stoodt J, Mulley J, Berkovic S, Scheffer IE, Brodtkorb E. Independent occurrence of the CHRNA4 Ser248Phe mutation in a Norwegian family with nocturnal frontal lobe epilepsy. Epilepsia. 2000;41:529–35.
McLellan A, Phillips HA, Rittey C, Kirkpatrick M, Mulley JC, Goudie D, et al. Phenotypic comparison of two Scottish families with mutations in different genes causing autosomal dominant nocturnal frontal lobe epilepsy. Epilepsia. 2003;44:613–7.
Hirose S, Iwata H, Akiyoshi H, Kobayashi K, Ito M, Wada K, et al. A novel mutation of CHRNA4 responsible for autosomal dominant nocturnal frontal lobe epilepsy. Neurology. 1999;53:1749–53.
Phillips HA, Marini C, Scheffer IE, Sutherland GR, Mulley JC, Berkovic SF. A de novo mutation in sporadic nocturnal frontal lobe epilepsy. Ann Neurol. 2000;48:264–7.
Sansoni V, Nobili L, Proserpio P, Ferini-Strambi L, Combi R. A de novo mutation in an Italian sporadic patient affected by nocturnal frontal lobe epilepsy. J Sleep Res. 2012;21:352–3.
Rozycka A, Skorupska E, Kostyrko A, Trzeciak WH. Evidence for S284L mutation of the CHRNA4 in a white family with autosomal dominant nocturnal frontal lobe epilepsy. Epilepsia. 2003;44:1113–7.
Ito M, Kobayashi K, Fujii T, Okuno T, Hirose S, Iwata H, et al. Electroclinical picture of autosomal dominant nocturnal frontal lobe epilepsy in a Japanese family. Epilepsia. 2000;41:52–8.
Leniger T, Kananura C, Hufnagel A, Bertrand S, Bertrand D, Steinlein OK. A new Chrna4 mutation with low penetrance in nocturnal frontal lobe epilepsy. Epilepsia. 2003;44:981–5.
Chen Y, Wu L, Fang Y, He Z, Peng B, Shen Y, et al. A novel mutation of the nicotinic acetylcholine receptor gene CHRNA4 in sporadic nocturnal frontal lobe epilepsy. Epilepsy Res. 2009;83:152–6.
Magnusson A, Stordal E, Brodtkorb E, Steinlein O. Schizophrenia, psychotic illness and other psychiatric symptoms in families with autosomal dominant nocturnal frontal lobe epilepsy caused by different mutations. Psychiatr Genet. 2003;13:91–5.
Phillips HA, Scheffer IE, Crossland KM, Bhatia KP, Fish DR, Marsden CD, et al. Autosomal dominant nocturnal frontal-lobe epilepsy: genetic heterogeneity and evidence for a second locus at 15q24. Am J Hum Genet. 1998;63:1108–16.
CAS PubMed Central PubMed Google Scholar
Gambardella A, Annesi G, De Fusco M, Patrignani A, Aguglia U, Annesi F, et al. A new locus for autosomal dominant nocturnal frontal lobe epilepsy maps to chromosome 1. Neurology. 2000;55:1467–71.
De Fusco M, Becchetti A, Patrignani A, Annesi G, Gambardella A, Quattrone A, et al. The nicotinic receptor beta 2 subunit is mutant in nocturnal frontal lobe epilepsy. Nat Genet. 2000;26:275–6.
Hoda JC, Gu W, Friedli M, Phillips HA, Bertrand S, Antonarakis SE, et al. Human nocturnal frontal lobe epilepsy: pharmocogenomic profiles of pathogenic nicotinic acetylcholine receptor beta-subunit mutations outside the ion channel pore. Mol Pharmacol. 2008;74:379–91.
Phillips HA, Favre I, Kirkpatrick M, Zuberi SM, Goudie D, Heron SE, et al. CHRNB2 is the second acetylcholine receptor subunit associated with autosomal dominant nocturnal frontal lobe epilepsy. Am J Hum Genet. 2001;68:225–31.
Diaz-Otero F, Quesada M, Morales-Corraliza J, Martinez-Parra C, Gomez-Garre P, Serratosa JM. Autosomal dominant nocturnal frontal lobe epilepsy with a mutation in the CHRNB2 gene. Epilepsia. 2008;49:516–20.
Bertrand D, Elmslie F, Hughes E, Trounce J, Sander T, Bertrand S, et al. The CHRNB2 mutation I312M is associated with epilepsy and distinct memory deficits. Neurobiol Dis. 2005;20:799–804.
Cho YW, Yi SD, Lim JG, Kim DK, Motamedi GK. Autosomal dominant nocturnal frontal lobe epilepsy and mild memory impairment associated with CHRNB2 mutation I312M in the neuronal nicotinic acetylcholine receptor. Epilepsy Behav. 2008;13:361–5.
Liu H, Lu C, Li Z, Zhou S, Li X, Ji L, et al. The identification of a novel mutation of nicotinic acetylcholine receptor gene CHRNB2 in a Chinese patient: Its possible implication in non-familial nocturnal frontal lobe epilepsy. Epilepsy Res. 2011;95:94–9.
Combi R, Ferini-Strambi L, Montruccoli A, Bianchi V, Malcovati M, Zucconi M, et al. Two new putative susceptibility loci for ADNFLE. Brain Res Bull. 2005;67:257–63.
Combi R, Dalpra L, Ferini-Strambi L, Tenchini ML. Frontal lobe epilepsy and mutations of the corticotropin-releasing hormone gene. Ann Neurol. 2005;58:899–904.
Combi R, Ferini-Strambi L, Tenchini ML. Compound heterozygosity with dominance in the Corticotropin Releasing Hormone (CRH) promoter in a case of nocturnal frontal lobe epilepsy. J Sleep Res. 2008;17:361–2.
Sansoni V, Forcella M, Mozzi A, Fusi P, Ambrosini R, Ferini-Strambi L, et al. Functional characterization of a CRH missense mutation identified in an ADNFLE family. PLoS One. 2013;8:e61306. This is the first mutation described in the coding region of the CRH gene associated with ADNFLE. Previous studies have shown that CRH promotes wakefulness and impairs sleep in a dose-dependent way, and may increase seizures susceptibility. Individuals with an altered CRH level could be more prone to developing the disease. .
Aridon P, Marini C, Di Resta C, Brilli E, De Fusco M, Politi F, et al. Increased sensitivity of the neuronal nicotinic receptor alpha 2 subunit causes familial epilepsy with nocturnal wandering and ictal fear. Am J Hum Genet. 2006;79:342–50.
Picard F, Bruel D, Servent D, Saba W, Fruchart-Gaillard C, Schollhorn-Peyronneau MA, et al. Alteration of the in vivo nicotinic receptor density in ADNFLE patients: a PET study. Brain. 2006;129:2047–60.
Lena C, Popa D, Grailhe R, Escourrou P, Changeux JP, Adrien J. Beta2-containing nicotinic receptors contribute to the organization of sleep and regulate putative micro-arousals in mice. J Neurosci. 2004;24:5711–8.
Klaassen A, Glykys J, Maguire J, Labarca C, Mody I, Boulter J. Seizures and enhanced cortical GABAergic inhibition in two mouse models of human autosomal dominant nocturnal frontal lobe epilepsy. Proc Natl Acad Sci U S A. 2006;103:19152–7.
Xu J, Cohen BN, Zhu Y, Dziewczapolski G, Panda S, Lester HA, et al. Altered activity-rest patterns in mice with a human autosomal-dominant nocturnal frontal lobe epilepsy mutation in the beta2 nicotinic receptor. Mol Psychiatry. 2011;16:1048–61. This experimental study shows that mice with a mutation in the beta2 nicotinic receptor may have an alteration of their sleep pattern, independent of the presence of epileptic activity. The study underlines the role of nicotinic receptors in the sleep regulation and has important implications for the interpretation of the pathophysiological mechanisms of ADNFLE. .
Parrino L, De Paolis F, Milioli G, Gioi G, Grassi A, Riccardi S, et al. Distinctive polysomnographic traits in nocturnal frontal lobe epilepsy. Epilepsia. 2012;53:1178–84.
Terzaghi M, Sartori I, Mai R, Tassi L, Francione S, Cardinale F, et al. Coupling of minor motor events and epileptiform discharges with arousal fluctuations in NFLE. Epilepsia. 2008;49:670–6.
Halasz P, Kelemen A, Szucs A. The role of NREM sleep micro-arousals in absence epilepsy and in nocturnal frontal lobe epilepsy. Epilepsy Res. 2013;107:9–19. This is a systematic review on the role of NREM sleep-related phasic events, micro-arousals, in two major idiopathic epilepsies. Taking into account both experimental and clinical findings, the authors discuss the role of arousal fluctuations in NFLE and the relationships between cholinergic alterations, thalamocortical oscillations, and seizures. .
Bisulli F, Vignatelli L, Naldi I, Licchetta L, Provini F, Plazzi G, et al. Increased frequency of arousal parasomnias in families with nocturnal frontal lobe epilepsy: a common mechanism? Epilepsia. 2010;51:1852–60. This details a case-control family study that recruited 33 NFLE probands, 200 relatives of probands, 31 controls, and 194 control relatives. The authors found an increased prevalence of arousal disorders (odds ratio 4.7) and nightmares (odds ratio 2.6) among NFLE proband relatives. The study suggests a possible common pathophysiological mechanism (based on an alteration of the cholinergic system) in parasomnias and NFLE. .
Ishida S, Picard F, Rudolf G, Noe E, Achaz G, Thomas P, et al. Mutations of DEPDC5 cause autosomal dominant focal epilepsies. Nat Genet. 2013;45:552–5. This study shows that loss-of-function mutations in DEPDC5 are a major cause of a broad spectrum of autosomal dominant focal epilepsies with different brain localization and electroclinical expression, including ADNFLE, familial temporal lobe epilepsy, and familial focal epilepsy with variable foci. The study indicates that the seizure initiation sites may be dissociated from underlying genetic mechanisms. .
Heron SE, Scheffer IE, Berkovic SF, Dibbens LM, Mulley JC. Channelopathies in idiopathic epilepsy. Neurotherapeutics. 2007;4:295–304.
Steinlein OK, Hoda JC, Bertrand S, Bertrand D. Mutations in familial nocturnal frontal lobe epilepsy might be associated with distinct neurological phenotypes. Seizure. 2012;21:118–23. This study shows that certain ADNFLE mutations might be associated with an increased risk of cognitive deficits, mental retardation, and psychiatric symptoms. These results suggest that a neuropsychological evaluation should be included in the clinical assessment of patients with ADNFLE. .
Blumcke I, Thom M, Aronica E, Armstrong DD, Vinters HV, Palmini A, et al. The clinicopathologic spectrum of focal cortical dysplasias: a consensus classification proposed by an ad hoc Task Force of the ILAE Diagnostic Methods Commission. Epilepsia. 2011;52:158–74.
PubMed Central PubMed Google Scholar
Nobili L, Cardinale F, Magliola U, Cicolin A, Didato G, Bramerio M, et al. Taylor's focal cortical dysplasia increases the risk of sleep-related epilepsy. Epilepsia. 2009;50:2599–604. This study evaluated the relative roles of the topography of the epileptogenic zone and of the etiologic substrate as risk factors for sleep-related focal epilepsy. The analysis of the clinical and histopathologic features of 303 drug-resistant patients seizure-free since surgery revealed that one of every two patients with a Taylor-type cortical dysplasia, independent of its location, had a sleep-related focal epilepsy. The presence of Taylor-type cortical dysplasia increases the risk of sleep-related focal epilepsy 14-fold. .
Proserpio P, Cossu M, Francione S, Gozzo F, Lo Russo G, Mai R, et al. Epileptic motor behaviors during sleep: anatomo-electro-clinical features. Sleep Med. 2011;12 Suppl 2:S33–8. This study included 40 patients with drug-resistant sleep-related focal epilepsy submitted to Stereo-Electroencephalography investigation and seizure-free after surgical treatment. The study points out that a significant proportion (30%) of sleep-related complex motor seizures may originate outside the frontal lobe. These results are clinically relevant when considering drug-resistant epileptic patients in whom a surgical approach could be an effective treatment. .
Chassoux F, Landre E, Mellerio C, Turak B, Mann MW, Daumas-Duport C, et al. Type II focal cortical dysplasia: electroclinical phenotype and surgical outcome related to imaging. Epilepsia. 2012;53:349–58.
Noli D, Bartuluchi M, Gonzalez FS, Kaltenmeier MC, Cersosimo R, Rugilo C, et al. Type II focal cortical dysplasia: electroclinical study and surgical outcome in 31 pediatric patients. Childs Nerv Syst. 2013;29:2079–87.
Tassi L, Garbelli R, Colombo N, Bramerio M, Russo GL, Mai R, et al. Electroclinical, MRI and surgical outcomes in 100 epileptic patients with type II FCD. Epileptic Disord. 2012;14:257–66.
Garbelli R, Frassoni C, Condorelli DF, Trovato Salinaro A, Musso N, Medici V, et al. Expression of connexin 43 in the human epileptic and drug-resistant cerebral cortex. Neurology. 2011;76:895–902.
Chassoux F, Devaux B, Landre E, Turak B, Nataf F, Varlet P, et al. Stereoelectroencephalography in focal cortical dysplasia: a 3D approach to delineating the dysplastic cortex. Brain. 2000;123:1733–51.
Nobili L, Sartori I, Terzaghi M, Stefano F, Mai R, Tassi L, et al. Relationship of epileptic discharges to arousal instability and periodic leg movements in a case of nocturnal frontal lobe epilepsy: a stereo-EEG study. Sleep. 2006;29:701–4.
Mai R, Sartori I, Francione S, Tassi L, Castana L, Cardinale F, et al. Sleep-related hyperkinetic seizures: always a frontal onset? Neurol Sci. 2005;26 Suppl 3:s220–4.
Terzaghi M, Sartori I, Mai R, Tassi L, Francione S, Cardinale F, et al. Sleep-related minor motor events in nocturnal frontal lobe epilepsy. Epilepsia. 2007;48:335–41.
Peled R, Lavie P. Paroxysmal awakenings from sleep associated with excessive daytime somnolence: a form of nocturnal epilepsy. Neurology. 1986;36:95–8.
Plazzi G, Tinuper P, Montagna P, Provini F, Lugaresi E. Epileptic nocturnal wanderings. Sleep. 1995;18:749–56.
Zucconi M, Oldani A, Smirne S, Ferini-Strambi L. The macrostructure and microstructure of sleep in patients with autosomal dominant nocturnal frontal lobe epilepsy. J Clin Neurophysiol. 2000;17:77–86.
Montagna P. Nocturnal paroxysmal dystonia and nocturnal wandering. Neurology. 1992;42(7 Suppl 6):61–7.
Nobili L, Francione S, Mai R, Tassi L, Cardinale F, Castana L, et al. Nocturnal frontal lobe epilepsy: intracerebral recordings of paroxysmal motor attacks with increasing complexity. Sleep. 2003;26:883–6.
Morris 3rd HH, Dinner DS, Luders H, Wyllie E, Kramer R. Supplementary motor seizures: clinical and electroencephalographic findings. Neurology. 1988;38:1075–82.
Rheims S, Ryvlin P, Scherer C, Minotti L, Hoffmann D, Guenot M, et al. Analysis of clinical patterns and underlying epileptogenic zones of hypermotor seizures. Epilepsia. 2008;49:2030–40.
Vaugier L, Aubert S, McGonigal A, Trebuchon A, Guye M, Gavaret M, et al. Neural networks underlying hyperkinetic seizures of "temporal lobe" origin. Epilepsy Res. 2009;86:200–8.
Tassinari CA, Rubboli G, Gardella E, Cantalupo G, Calandra-Buonaura G, Vedovello M, et al. Central pattern generators for a common semiology in fronto-limbic seizures and in parasomnias. A neuroethologic approach. Neurol Sci. 2005;26 Suppl 3:s225–32.
Tassinari CA, Cantalupo G, Hogl B, Cortelli P, Tassi L, Francione S, et al. Neuroethological approach to frontolimbic epileptic seizures and parasomnias: the same central pattern generators for the same behaviours. Rev Neurol (Paris). 2009;165:762–8.
Proserpio P, Cossu M, Francione S, Tassi L, Mai R, Didato G, et al. Insular-opercular seizures manifesting with sleep-related paroxysmal motor behaviors: a stereo-EEG study. Epilepsia. 2011;52:1781–91. These data reinforce the concept that sleep-related complex motor seizures can originate outside the frontal lobe and give helpful electroclinical information to distinguish patients with sleep-related insular–opercular seizures. Moreover, this study indicates that intracranial EEG in the insula can be performed safely, and surgical treatment may represent a highly effective treatment option in these drug-resistant patients. .
Kaido T, Otsuki T, Nakama H, Kaneko Y, Kubota Y, Sugai K, et al. Complex behavioral automatism arising from insular cortex. Epilepsy Behav. 2006;8:315–9.
Dobesberger J, Ortler M, Unterberger I, Walser G, Falkenstetter T, Bodner T, et al. Successful surgical treatment of insular epilepsy with nocturnal hypermotor seizures. Epilepsia. 2008;49:159–62.
Isnard J, Guenot M, Sindou M, Mauguiere F. Clinical manifestations of insular lobe seizures: a stereo-electroencephalographic study. Epilepsia. 2004;45:1079–90.
Tinuper P, Provini F, Bisulli F, Vignatelli L, Plazzi G, Vetrugno R, et al. Movement disorders in sleep: guidelines for differentiating epileptic from non-epileptic motor phenomena arising from sleep. Sleep Med Rev. 2007;11:255–67.
Plazzi G, Montagna P, Provini F, Tinuper P, Lugaresi E. Sudden arousals from slow-wave sleep and panic disorder. Sleep. 1998;21:548.
ASDA. American Academy of Sleep Medicine: The international classification of sleep disorders: diagnostic and coding manual. 2nd ed. Westchester: American Academy of Sleep Medicine; 2005.
Google Scholar
Vignatelli L, Bisulli F, Zaniboni A, Naldi I, Fares JE, Provini F, et al. Interobserver reliability of ICSD-R minimal diagnostic criteria for the parasomnias. J Neurol. 2005;252:712–7.
Derry CP, Davey M, Johns M, Kron K, Glencross D, Marini C, et al. Distinguishing sleep disorders from seizures: diagnosing bumps in the night. Arch Neurol. 2006;63:705–9.
Bisulli F, Vignatelli L, Naldi I, Pittau F, Provini F, Plazzi G, et al. Diagnostic accuracy of a structured interview for nocturnal frontal lobe epilepsy (SINFLE): a proposal for developing diagnostic criteria. Sleep Med. 2012;13:81–7. The objective of this investigation was to measure the accuracy of anamnestic features collected during clinical history for the diagnosis of NFLE. The authors conducted a a case-control diagnostic study including a case group of NFLE and a control group of people with sleep disorders potentially confounding for NFLE. The study disclosed two major anamnestic patterns (dystonic posturing and hyperkinetic behaviors) and four minor features (duration < 2 mins, unstructured vocalization during the episode, experience of an aura preceding the motor attack, and a history of tonic–clonic seizures during sleep) with unsatisfactory sensitivity, but high specificity. .
Manni R, Terzaghi M, Repetto A. The FLEP scale in diagnosing nocturnal frontal lobe epilepsy, NREM and REM parasomnias: data from a tertiary sleep and epilepsy unit. Epilepsia. 2008;49:1581–5.
Manni R, Terzaghi M, Zambrelli E. REM sleep behavior disorder and epileptic phenomena: clinical aspects of the comorbidity. Epilepsia. 2006;47 Suppl 5:78–81.
Derry CP, Harvey AS, Walker MC, Duncan JS, Berkovic SF. NREM arousal parasomnias and their distinction from nocturnal frontal lobe epilepsy: a video EEG analysis. Sleep. 2009;32:1637–44. This study analyzed a video–EEG monitoring series focusing on the semiological features of the arousal parasomnias and NFLS with the aim of making video analysis of nocturnal paroxysmal events more reliable. Dystonic posturing and hyperkinetic automatisms (e.g., kicking, rocking, or cycling movements) were found to be specific for NFLS. In the absence of these major features the presence of verbal interaction and failure to achieve full arousal after the event strongly suggest a diagnosis of parasomnia. However, other motor patterns, mainly characterized by brief motor manifestations or complex fearful behaviors, like sitting, standing, or walking, did not discriminate between the two conditions. .
Nobili L. Can homemade video recording become more than a screening tool? Sleep. 2009;32:1544–5.
Nobili L. Nocturnal frontal lobe epilepsy and non-rapid eye movement sleep parasomnias: differences and similarities. Sleep Med Rev. 2007;11:251–4.
Lugaresi E, Cirignotta F. Hypnogenic paroxysmal dystonia: epileptic seizure or a new syndrome? Sleep. 1981;4:129–38.
Picard F, Bertrand S, Steinlein OK, Bertrand D. Mutated nicotinic receptors responsible for autosomal dominant nocturnal frontal lobe epilepsy are more sensitive to carbamazepine. Epilepsia. 1999;40:1198–209.
Provini F, Plazzi G, Montagna P, Lugaresi E. The wide clinical spectrum of nocturnal frontal lobe epilepsy. Sleep Med Rev. 2000;4:375–86.
Raju GP, Sarco DP, Poduri A, Riviello JJ, Bergin AM, Takeoka M. Oxcarbazepine in children with nocturnal frontal-lobe epilepsy. Pediatr Neurol. 2007;37:345–9.
Oldani A, Manconi M, Zucconi M, Martinelli C, Ferini-Strambi L. Topiramate treatment for nocturnal frontal lobe epilepsy. Seizure. 2006;15:649–52.
Varadkar S, Duncan JS, Cross JH. Acetazolamide and autosomal dominant nocturnal frontal lobe epilepsy. Epilepsia. 2003;44:986–7.
Willoughby JO, Pope KJ, Eaton V. Nicotine as an antiepileptic agent in ADNFLE: an N-of-one study. Epilepsia. 2003;44:1238–40.
Brodtkorb E, Picard F. Tobacco habits modulate autosomal dominant nocturnal frontal lobe epilepsy. Epilepsy Behav. 2006;9:515–20.
Naldi I, Bisulli F, Vignatelli L, Licchetta L, Pittau F, Di Vito L, et al. Tobacco habits in nocturnal frontal lobe epilepsy. Epilepsy Behav. 2013;26:114–7.
De Paolis F, Colizzi E, Milioli G, Grassi A, Riccardi S, Puligheddu M, et al. Effects of antiepileptic treatment on sleep and seizures in nocturnal frontal lobe epilepsy. Sleep Med. 2013;14:597–604. In this study the authors report the effects of antiepileptic drug treatment on both sleep macro- and microstructure, and on video-PSG-recorded seizures in a group of NFLE patients. A significant reduction of major seizures was observed; however, the authors also report the persistence of a certain number of minor seizures and a high sleep instability. The authors suggest a peculiar sleep microstructure instability as a possible trait marker of NFLE. .
Download references
Acknowledgments
We thank Dr. Steve Gibbs for insightful discussions.
Compliance with Ethics Guidelines
Conflict of interest.
Lino Nobili, Paola Proserpio, Romina Combi, Federica Provini, Laura Tassi, and Paolo Tinuper declare that they have no conflict of interest.
Giuseppe Plazzi has received financial compensation for board membership for UCB Pharma and Jazz Pharmaceuticals.
Francesca Bisulli has received grants from the Italian Ministry of Health.
Human and Animal Rights and Informed Consent
This article does not contain any studies with human or animal subjects performed by any of the authors.
Author information
Authors and affiliations.
Centre for Epilepsy Surgery, Niguarda Hospital, Piazza Ospedale Maggiore 3, 20154, Milan, Italy
Lino Nobili, Paola Proserpio & Laura Tassi
Centre of Sleep Medicine, Niguarda Hospital, Milan, Italy
Lino Nobili & Paola Proserpio
Institute of Bioimaging and Molecular Physiology, National Research Council, Milan, Italy
Lino Nobili
Department of Biotechnology and Biosciences, University of Milano-Bicocca, Milan, Italy
Romina Combi
IRCCS Institute of Neurological Sciences of Bologna, Bologna, Italy
Federica Provini, Giuseppe Plazzi, Francesca Bisulli & Paolo Tinuper
Department of Biomedical and Neuromotor Sciences, University of Bologna, Bologna, Italy
You can also search for this author in PubMed Google Scholar
Corresponding author
Correspondence to Lino Nobili .
Additional information
This article is part of the Topical Collection on Sleep
Rights and permissions
Reprints and permissions
About this article
Nobili, L., Proserpio, P., Combi, R. et al. Nocturnal Frontal Lobe Epilepsy. Curr Neurol Neurosci Rep 14 , 424 (2014). https://doi.org/10.1007/s11910-013-0424-6
Download citation
Published : 07 January 2014
DOI : https://doi.org/10.1007/s11910-013-0424-6
Share this article
Anyone you share the following link with will be able to read this content:
Sorry, a shareable link is not currently available for this article.
Provided by the Springer Nature SharedIt content-sharing initiative
- Nocturnal frontal lobe epilepsy
- Autosomal nocturnal frontal lobe epilepsy
- Parasomnias
- Sleep-related epilepsy
- Focal cortical dysplasia
Advertisement
- Find a journal
- Publish with us
- Track your research
- Open access
- Published: 17 July 2019
Sleep related hyper motor epilepsy (SHE): a unique syndrome with heterogeneous genetic etiologies
- Francesca Bisulli ORCID: orcid.org/0000-0002-1109-7296 1 , 2 ,
- Laura Licchetta 1 , 2 &
- Paolo Tinuper 1 , 2
Sleep Science and Practice volume 3 , Article number: 3 ( 2019 ) Cite this article
19k Accesses
5 Citations
4 Altmetric
Metrics details
Sleep-related hypermotor epilepsy (SHE), formerly known as Nocturnal Frontal Lobe Epilepsy is a focal epilepsy characterized by seizures with complex hyperkinetic automatisms and/or asymmetric tonic/dystonic posturing occurring mostly during sleep. SHE is a rare disease with an estimated minimum prevalence of 1.8/100,000 individuals and represent about 10% of drug-resistant surgical cases. This disorder, though uncommon, is of considerable interest to a broad spectrum of specialists, from child neurologists to neurosurgeons. Distinguishing this condition from non-epileptic paroxysmal behaviour occurring physiologically or pathologically during sleep is often difficult and sometimes impossible on clinical grounds alone, even for experienced epileptologists and sleep physicians. Recognized aetiologies of SHE are heterogeneous and include acquired injuries, genetic causes and structural anomalies such as focal cortical dysplasia. Multiple aetiologies (structural-genetic) are also possible. Non-specific clinical features distinguished different aetiologies even if SHE due to structural lesions usually manifests with early-onset drug-resistant seizures and showed a worse long-term prognosis.
The causative genes for SHE are multiple and encode for proteins involved in different molecular pathways. The cholinergic system and the mTOR pathway are the most relevant. This review will provide an exhaustive overview of the genetic background of SHE.
Sleep-related Hypermotor epilepsy (SHE)
SHE, formerly Nocturnal Frontal Lobe Epilepsy (NFLE), is a focal epilepsy characterized by hyperkinetic seizures occurring predominantly in clusters during non-REM sleep.
This disorder affects individuals of both sexes and any age, with a peak of seizure onset during childhood and adolescence (Scheffer et al. 1994 ; Tinuper et al. 2016 ). A familial form of SHE with autosomal dominant inheritance (ADSHE) has been described. So far, more than 100 families have been identified worldwide (Marini and Guerrini 2007 ; Steinlein 2014 ), but no accurate data concerning the prevalence of ADSHE exist.
The estimated prevalence of non-familial SHE in the adult population is 1.8–1.9 per 100,000 (Vignatelli et al. 2015 ; Vignatelli et al. 2017 ). However, the disorder is likely to be under diagnosed, or in some cases misdiagnosed. Distinguishing this condition from non-epileptic paroxysmal behaviour occurring physiologically or pathologically during sleep is often difficult and sometimes impossible on clinical grounds alone, even for experienced epileptologists and sleep physicians. As a result, misdiagnosis is common and patients may be denied effective treatments or treated inappropriately, leading to long-term side effects and the social consequences of erroneous epilepsy diagnosis (e.g., impacts on driver’s licence).
Most patients show a good response to the pharmacological treatment, low doses of carbamazepine at bedtime being the first choice of therapy. However, about one-third of patients are drug-resistant and only 22% achieved terminal remission after a median 16-year follow-up, most with a remitting pattern from disease onset (Licchetta et al., 2017 ). These data, showing the poor outcome after a long follow-up, possibly explain the reason why SHE has been reported in up to 10% of surgical series (Menghi et al. 2018 ). The surgical outcome seems to be relatively good in this population, especially in patients with positive brain MRI.
From NPD to SHE
First described in 1981 (Lugaresi and Cirignotta 1981 ), the condition was initially considered a new motor disorder of sleep, namely parasomnia, and the misleading term Nocturnal Paroxysmal Dystonia (NPD) was introduced. Subsequently, similarity of the attacks to those in patients with frontal lobe epilepsy undergoing neurosurgical evaluation (Williamson et al. 1985 ; Waterman et al. 1987 ) and documentation of epileptiform discharges in some patients (Tinuper et al. 1990 ) proved the epileptic origin of the syndrome. The disorder was therefore re-named Nocturnal Frontal Lobe Epilepsy (NFLE). In the following two decades, the clinical boundaries of the disorder were defined, however many controversial issues highlighted the need to change the nomenclature (Tinuper and Bisulli 2017 ). In 2014, a Consensus Conference was held in Bologna, Italy (Tinuper et al. 2016 ). Experts in the field discussed the spectrum of NFLE and produced a final Consensus Statement based on a rigorous protocol addressing nomenclature, electro-clinical definition, diagnostic criteria with levels of certainty supported by available evidence, aetiology, and research needs. The Consensus Conference highlighted three critical issues justifying the change of nomenclature. First, the term nocturnal was considered misleading because it implies a chronobiological pattern of seizure occurrence, whereas evidence indicates that seizure occurrence in sleep is the most important characteristic rather than the time of day. Second, the term frontal lobe is not always appropriate because the characteristic seizures may also arise from extra-frontal areas (Proserpio et al. 2011 ). Third, the term NFLE did not specify the typical clinical semiology involved, which consists primarily of hyperkinetic seizures (Tinuper et al. 2016 ). For all these reasons the term Sleep related Hypermotor Epilepsy (SHE) has been proposed to replace NFLE.
Clinical features
Seizures in SHE are usually brief (< 2 min in duration), with an abrupt onset and offset and with stereotyped motor patterns. The hypermotor semiology is the primary clinical pattern of the seizures, characterized by hyperkinetic features possibly associated with asymmetric tonic/dystonic posturing with or without head/eye deviation. In a minority of cases the asymmetric dystonic posturing is the unique feature of the seizures (Vignatelli et al. 2015 ). Seizures typically show variable complexity and duration varying from brief stereotyped sudden arousals from sleep (paroxysmal arousals or minor motor events) to more complex dystonic-dyskinetic seizures and, more rarely, prolonged ambulatory behavior known as “epileptic nocturnal wandering” (Montagna 1992 ; Provini et al. 1999 ; Nobili et al. 2003 ; Terzaghi et al. 2008 ). Retained awareness during seizures is common and affected individuals may report a distinct aura. Seizures occur typically in cluster during (non-REM) sleep even if episodes during active wakefulness may rarely occur during the patient’s lifetime.
Seizure frequency in SHE patients can be very high, ranging from one to more than 50 attacks per night (Scheffer et al. 1994 ; Provini et al. 1999 ) and paroxysmal arousals or minor motor events may be even more frequent. SHE patients can complain of nocturnal sleep disruption with spontaneous midsleep awakening, sleep inertia in the morning, tiredness at awakening and excessive sleepiness (Peled and Lavie 1986 ; Maccario and Lustman 1990 ; Schwalen and Jorg 1998 ; Zucconi et al. 2000 ; Alanis-Guevara et al. 2005 ) impacting negatively on their quality of life. Although daytime sleepiness-related symptoms could be common in SHE patients, the frequency of excessive daytime sleepiness did not statistically differ compared to controls (Vignatelli et al. 2006 ).
Neurophysiological features
Background activity is usually normal. Interictal EEG is normal in about half cases (Licchetta et al. 2017 ) or may demonstrate rare epileptiform abnormalities, enhanced by sleep deprivation and occurring mainly during sleep (Menghi et al. 2018 ). The ictal scalp EEG may be normal or may only demonstrate movement artifacts. Epileptiform abnormalities, rhythmic slow activity or diffuse background flattening over frontal areas are seen in 50–60% of cases (Licchetta et al. 2017 ).
Scalp EEG and invasive intracranial stereo-electroencephalography recordings (SEEG) documented a frontal lobe origin of seizures in most cases (Nobili et al. 2007 ; Rheims et al. 2008 ). However, in up to 20% of drug resistant cases the ictal discharges may arise from various extra-frontal areas including temporal (Nobili et al. 2004 ; Vaugier et al. 2009 ), insulo-opercular (Ryvlin et al. 2006 ; Dobesberger et al. 2008 ; Nguyen et al. 2009 ; Proserpio et al. 2011 ) and parietal (Montavont et al. 2013 ; Gibbs et al. 2016 ) cortices, then propagating to the frontal cortex and resulting in hypermotor seizures.
Diagnostic criteria
The clinical history and clinicffttal semiology of the attacks are the main criteria to establish the diagnosis, as both interictal and ictal EEG may be uninformative (Scheffer et al. 1994 ; Oldani et al. 1998 ; Nobili et al. 2007 ; Licchetta et al. 2017 ). Three categories for the diagnosis with different levels of certainty have been proposed: 1) Witnessed (possible), based on the description of the core clinical features, as provided by an eye-witness; 2) Video Documented (clinical), based on the evaluation of at least 1 entire (preferably 2) video recorded hyperkinetic episode, confirmed to be typical by witness; 3) Video-EEG documented (confirmed), requiring the video-polygraphic recording of stereotyped events (one or two) and ictal or interictal epileptiform abnormalities (Tinuper et al. 2016 ).
Etiology is unknown in the majority of patients. Recognized etiologies of SHE are heterogeneous and include acquired injuries, genetic causes and structural anomalies such as focal cortical dysplasia (FCD). Multiple etiologies (structural-genetic) are also possible. Non-specific clinical features distinguished different etiologies (Tinuper et al. 2016 ) even if SHE due to structural lesions (FCD) usually manifests with early-onset drug-resistant seizures (Nobili et al. 2009 ) and showed a worse long-term prognosis (Licchetta et al. 2017 ). In these cases, epilepsy surgery and removal of the epileptogenic zone could represent a highly effective treatment option (Nobili et al. 2007 ).
Most patients (86%) are sporadic cases, while 14% reported a family history for epilepsy with only 5% of cases showing a clear-cut autosomal dominant pattern of inheritance, i.e. ADSHE (Licchetta et al. 2017 ). So far ADSHE has been associated with mutations in several genes, encoding proteins involved in different biological pathways. Although the diverse underlying aetiologies and networks involved in its pathogenesis, according to the current state of knowledge, it is considered a single syndrome, defined by clinical manifestations (i.e. hypermotor seizures) resulting from presumed shared downstream mechanisms occurring during sleep/wake oscillation changes. In clinical practice it is most helpful to consider SHE as a single syndrome because it requires a specific diagnostic work-up and therapeutic approach e (Tinuper et al. 2016 ).
Genetics SHE
SHE is the first epilepsy syndrome in which a genetic aetiology was documented. It is also the first the first epilepsy channelopathy described, as it was initially related to mutations in genes coding for subunits of the neuronal nicotinic acetylcholine receptor (nAChR), CHRNA4 , CHRNB2 and CHRNA2 (Steinlein et al. 1995 ).
Subsequently, SHE has been associated with mutations in several other genes, encoding proteins involved in different biological pathways, such as, CRH , KCNT1 , DEPDC5, NPRL2, NPRL3 and PRIMA1 .
Inherited SHE usually shows an autosomal dominant pattern of transmission, except for a single reported family mutated in PRIMA1 , showing an autosomal recessive inheritance (Hildebrand et al. 2015 ).
Ach receptor genes
In 1994, Scheffer et al. (Scheffer et al. 1994 ) described the first large Australian family with SHE inherited in an autosomal dominant manner and named this condition Autosomal Dominant Nocturnal Frontal Lobe Epilepsy (ADNFLE). In this family, SHE had been misdiagnosed as a sleep disorder in many affected members.
Marked variations in severity have been observed amongst different members. Further molecular genetic studies established linkage to chromosome 20q13.2– q13.13 in some families (Phillips et al. 1995 ), leading to the subsequent identification of the causative role of the gene coding for the α 4-subunit of the nAChR, CHRNA4 (Cholinergic Receptor Nicotinic Alpha 4 Subunit, MIM *118504) (Steinlein et al. 1995 ). Following the initial recognition, more than a hundred families have been described worldwide (Marini and Guerrini 2007 ) and mutations in two homologous genes, CHRNB2 (Cholinergic Receptor Nicotinic Beta 2 Subunit, MIM *118507) and CHRNA2 (Cholinergic Receptor Nicotinic Alpha 2 Subunit, MIM *118502), encoding the β2 and α2 subunit of the nAChR respectively, have been identified (De Fusco et al. 2000 ; Aridon et al. 2006 ). The phenotype produced by mutations of the three nAChR subunit genes is generally indistinguishable (McLellan et al. 2003 ).
By now, 14 different mutations in CHRNA4 , CHRNB2 and CHRNA2 have been reported in 20 ADSHE pedigrees and three sporadic cases, as reported in Table 1 . Overall, they account for less than 20% of SHE/ADSHE cases, reflecting the genetic heterogeneity of the syndrome and the possible role of systems other than the cholinergic one, involved in its pathogenesis (Steinlein et al. 2012 ).
In-vitro analyses of the functional properties of nAChR disclosed a functional gain (i.e. an increase in acetylcholine sensitivity) (Bertrand et al. 2002 ) of mutant receptors associated with ADSHE that may underlie the neuronal network dysfunction responsible for the epileptic seizures. Positron-emission tomography (PET) studies in ADSHE patients suggest hyperactivation of the cholinergic pathway ascending from the brainstem (Picard et al. 2006 ). Cholinergic neurons modulate sleep and arousal at both thalamic and cortical levels and their involvement in sleep-related disorders is plausible, although the pathophysiological mechanism remains elusive.
Interestingly, a high prevalence of NREM arousal parasomnias has been reported not only by SHE probands but also by their healthy relatives (Bisulli et al. 2010 ; Licchetta et al. 2017 ) suggesting a possible common background. The relationship between these disorders is also supported by the neurophysiological and neuro-imaging evidence that the pathway controlling physiologic arousal is impaired in both conditions (Picard et al. 2006 ; Montagna et al. 2008 ; Fedi et al. 2008 ).
Seizure frequency improved in a single patient with refractory ADSHE after nicotine transdermal patches treatment (Willoughby et al. 2003 ).
The favourable effect of nicotine on seizure frequency was also described in 9 out of 22 patients from two European ADSHE families carrying CHRNA4 mutations (Brodtkorb and Picard 2006 ). Considering the role of the cholinergic system in arousal regulatory processes, these observations suggested a possible link between nicotine defect, alteration of arousal regulation and seizures in SHE/ADSHE patients. However, despite the reported positive effect of nicotine in reducing seizure frequency, a case–control family study, did not find a higher tendency to smoke tobacco in SHE patients and their relatives compared to the control cases (Naldi et al. 2013 ).
Recently, Puligheddu and colleagues have shown that fenofibrate, an agonist at peroxisome proliferator-activated receptor alpha (PPARa) acting as a negative modulator of nAChRs, may have beneficial effects both in mutated mouse models of SHE and pharmacoresistant SHE patients (Puligheddu et al. 2017 ). Interestingly, good results with fenofibrate administration have been obtained both in the mutated ( CHRNA2 and CHRNA4 ) and non-mutated SHE patients.
Other mutations besides the ones coding for the nAChR subunits have been reported in both sporadic and ADSHE cases. In 2005, Combi et al. found two new nucleotide variations in the CRH (corticotropin-releasing hormone, MIM *122560) promoter in ADSHE pedigrees and sporadic cases (Combi et al. 2005 ) but these findings were not replicated in larger series. The first one (g.1470G > A) recurred in three ADSHE pedigrees and two patients without family history and it was shown to increase CRH levels. The same change was later identified in two affected siblings of another family, but it was also present in a homozygous status in the healthy father (Combi et al. 2008 ), thus reducing the likelihood that the change is pathogenic. The second one (g.1166G > C) was found only in the index-case of a family (Combi et al. 2005 ) and later recognized as non-causative (Combi et al. 2008 ).
Finally, a novel heterozygous exonic missense change was detected in an additional ADSHE family. In vitro assay in this case showed decreased CRH concentrations (Sansoni et al. 2013 ).
CRH encodes for a neurotransmitter/neuromodulator widely distributed throughout the central nervous system that acts in extrahypothalamic circuits to integrate a multisystem response to stress that controls numerous behaviours such as sleep and arousal (Combi et al. 2005 ). The authors suggested that altered (decreased/increased) CHR levels cause increased susceptibility of seizures through excessive sleep fragmentation and brain hyperexcitability (Combi et al. 2005 ).
Further insight into the genetic background of SHE occurred only from 2012 when, combining genome-wide linkage analysis with novel Next Generation Sequencing (NGS) techniques, Heron and coauthors identified a novel gene for SHE, KCNT1 (Potassium Sodium-Activated Channel Subfamily T Member 1, MIM *608167), encoding a subunit of the sodium-activated potassium channel (Heron et al. 2012 ). Mutations in KCNT1 were detected in three ADSHE families with complete penetrance and a sporadic case, all with early-onset refractory seizures, possible intellectual disability and psychiatric or behavioral problems including psychosis, catatonia and aggression (Heron et al. 2012 ). Co-occurrence of mild malformation of cortical development (MCD), namely histologically-confirmed FCD type I and periventricular nodular heterotopia, have been reported very recently in some individuals (Rubboli et al. 2018 ).
Simultaneously, de novo gain-of-function mutations in KCNT1 were identified in six out of 12 unrelated individuals with Malignant Migrating Focal Seizures of Infancy (MMFSI) (Barcia et al. 2012 ), a rare early onset epileptic encephalopathy characterized by refractory, polymorphous focal seizures and arrest of psychomotor development within the first 6 months of life (Coppola et al. 1995 ).
KCNT1 is expressed in the neurons of the frontal cortex (Bhattacharjee et al. 2002 ) and assemble with KCNT2 to form heterotetrameric channel complexes composed of a small amino-terminal domain, a transmembrane domain containing six segments and a large intracellular carboxy-terminal domain containing tandem regulators of potassium conductance domains and an NAD + binding domain. Its activity contributes to the slow hyperpolarization that follows repetitive firing, regulates the rate of bursting and enhances the accuracy with which action potentials lock incoming stimuli (Bhattacharjee and Kaczmarek 2005 ; Brown et al. 2008 ). All the mutations initially described in both ADSHE and MMFSI were clustered around the regulator of potassium conductance and Nicotinamide Adenine Dinucleotide (NAD+) binding domains, which also interacts with a protein network, including fragile X mental retardation protein. Functional study documented that KCNT1 mutations cause a constitutive hyperactivation of the channel that impairs its gating and suppress its subconductance states whit effect on ion currents and increased amplitude. Moreover, they may also alter the conformation of the C-terminal region and its ability to interact with developmentally relevant proteins (Barcia et al. 2012 ). ADSHE mutations were associated with currents approximately 3-fold larger than wild type, while those associated with MMFSI were about 5-fold greater. These differences in the increased ion current amplitude seemed to explain the diversity on phenotypes associated with KCNT1 mutations (Milligan et al. 2014 ). Some of the variants recurred in several patients, suggesting the presence of mutational “hot spots” in KCNT1 (Møller et al. 2015 ). Specific mutations (p.G288S and p.R398Q) can lead to either ADSHE or MMFSI, even within the same family, indicating that genotype–phenotype correlations are not straightforward (Kim et al. 2014 ; Møller et al. 2015 ).
In the last few years, KCNT1 has been implicated in a wide spectrum of focal/multifocal epilepsies and early onset epileptic encephalopathies, in addition to ADSHE and MMFSI phenotypes (Shimada et al. 2014 ; Møller et al. 2015 ; Ohba et al. 2015 ; Rizzo et al. 2016 ).
Very recently, a new therapeutic approach with quinidine was tested in few drug-resistant epileptic patients carrying KCNT1 genetic mutations, no one fitting SHE phenotype, with conflicting results (Mikati et al. 2015 ; Abdelnoura et al. 2018 ).
Surgical treatment of the three unrelated patients with FCD type I reported was ineffective in two individuals (Engel Class IV) and only ameliorate seizure frequency in one (Engel Class II). The authors postulated that the poor surgical outcome may be due to the diffuse nature of FCD type I or to a wider epileptogenic network caused by germline KCNT1 mutations that sustains seizure propensity after epilepsy surgery (Rubboli et al. 2018 ).
GATOR1-complex genes
In 2013, mutations in DEPDC5 (DEP Domain Containing 5, MIM *614191) were implicated in familial focal epilepsy with variable foci (FFEVF) (Dibbens et al. 2013 ), as well as and in a variable percentage (12.5–37%) of heterogenous familial FEs, including ADSHE (Ishida et al. 2013 ; Picard et al. 2014 ).
In particular, DEPDC5 loss-of-function mutations were found in the 13% of a series of 30 families with ADSHE presentation (Picard et al. 2014 ). Electro-Clinical assessments revealed a higher rate of drug resistance and of daytime seizures compared to classical phenotype (Picard et al. 2014 ). DEPDC5 mutations are associated with both lesional and non-lesional epilepsies, even within the same family. In some individuals, brain MRI disclosed MCD, ranging from FCD to subtle band heterotopia, with the predominant pattern being bottom-of-the-sulcus dysplasia, a variety of FCD type IIb (Scheffer et al. 2014 ) (Fig. 1 a-c; Fig. 2 a-c).
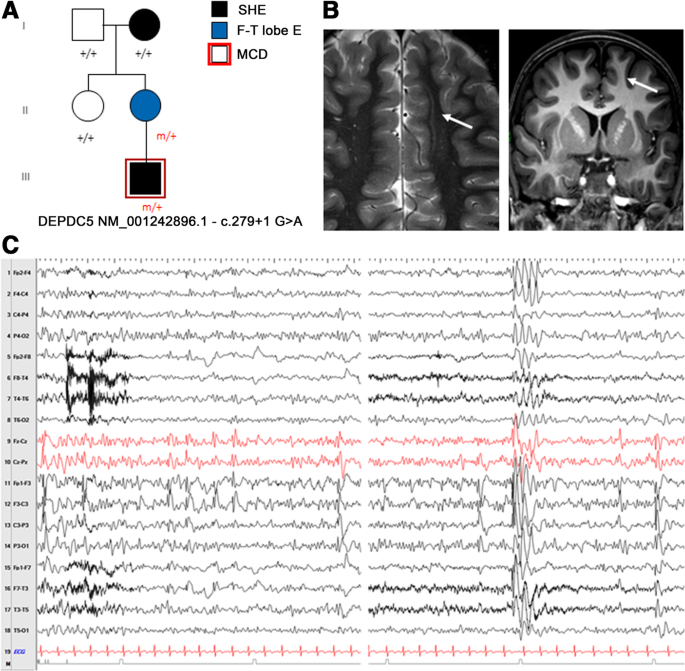
a Pedigree of the family carring the splicing mutation in DEPDC5 c.279 + 1 G > A. Individual III.1 showed Malformation of cortical development at 3 T brain MRI. Previously published in Dibbens et al. 2013 (fam I); Scheffer et al. 2014 (fam C). b Brain MRI of individual III.1. The white arrows point to the unilateral subtle band heterotopia within the white matter adjacent to dysplastic cortex in the left frontal lobe. Blurring of the gray–white matter junction involving part of the cingulate cortex and left frontal cortex was seen. c Interictal EEG of the same individual (III.1) showing frequent spike-wave discharges over the left frontal region (sometimes with phase opposition on F3) enhanced by drowsiness and light sleep, spreading to the ipsilateral and contralateral hemispheres
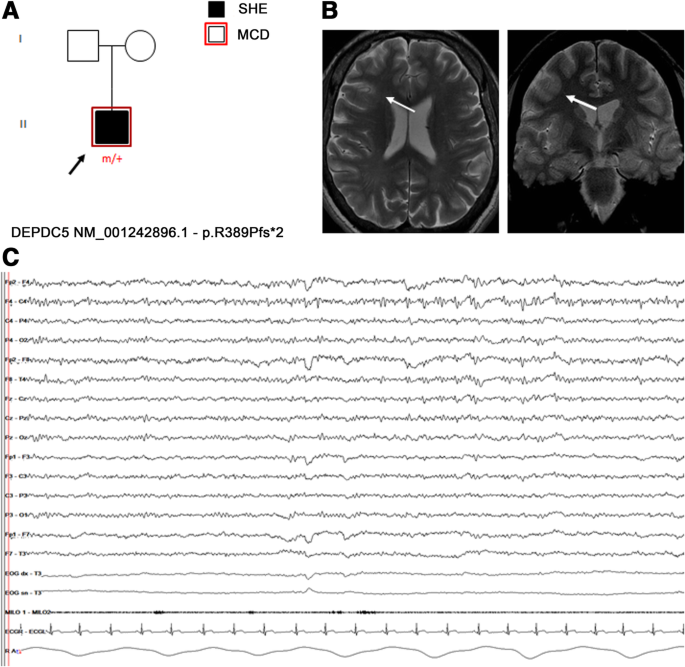
a Pedigree of an isolated SHE patient carring the frameshift DEPDC5 mutation p.R389Pfs*2. Previously published in Baldassari et al., 2019 (Proband 18). b Targeted brain MRI showed a focal increase of cortical thickness with blurring of gray-white matter junction, over the right medium frontal gyrus (white arrows), suggestive of focal cortical dysplasia. c Interictal EEG showing repetitive low-amplitude sharp-waves over the right fronto-central region, enhanced by drowsiness
Subsequently, mutations in NPRL2 (NPR2-like Protein, MIM *607072) (Ricos et al. 2016 ), NPRL3 (Nitrogen Permease Regulator-like 3, MIM *600928) (Korenke et al. 2016 ) have been reported in ADSHE and sporadic cases.
DEPDC5 , NPRL2 and NPRL3 are components of the GATOR1 complex (Gap Activity TOward Rags 1), a negative regulator of the mammalian target of rapamycin (mTOR) complex1 (mTORC1) (Bar-Peled et al. 2013 ). Most of the variants described in these genes are loss of function mutations, with impact on the protein product and consequent hyperactivation of mTORC1 pathway (van Kranenburg et al. 2015 ). In line with this evidence, so far a wide number of germline and somatic (brain-only) mutations of the mTOR-pathway genes have been associated with a range of lesional and non-lesional FEs. With regards to lesional cases, mutations in these genes have been identified in several MCDs and in particular in up to 46% of FCD type IIb (Nakashima et al. 2015 ; Scheffer et al. 2014 ; Lal et al. 2014 ; Martin et al. 2014 ; Scerri et al. 2015 ; D'Gama et al. 2015 ; Baulac et al. 2015 ).
Additional insights into the role of DEDCD5 in FCD-related focal epilepsies derive from the rat model (Marsan et al. 2016 ). The heterozygous rats exhibited an altered cortical neuron excitability and firing patterns and cortical cytomegalic dysmorphic neurons and balloon-like cells strongly expressing phosphorylated rpS6, supporting mTORC1 upregulation. These neuropathological abnormalities are reminiscent of the hallmark brain pathology of human FCD.
These data showed that genetic and structural cause are not mutually exclusive either and, in particular, SHE related to GATOR1-complex genes may have a genetic-structural etiology.
FCDs represent the most common, potentially treatable architectural disorder underlying FE, responsible for up to 42% of drug-resistant cases (Harvey et al. 2008 ). In general, epilepsy surgery is a highly effective curative option in these patients, affording the opportunity to achieve seizure freedom and potential medication withdrawal also with improvements in quality of life, employment rates and school attendance (Wiebe et al. 2001 ). Although mutated patients who have undergone epilepsy surgery are anecdotal, surgery has proved to be curative in cases with MCD clearly detectable with conventional neuroimaging (Baulac et al. 2015 ), suggesting that epileptogenesis is underpinned by a genetically determined, focal cerebral structural lesion, even in the presence of germline mutations. On the other hand the role of surgery in non lesion cases with germline mutation is still controversial/needs to be clarify. In the cited series non lesional cases who underwent surgery had a worse outcome compared with the lesional ones (Baulac et al. 2015 ). Moreover, a SEEG study in a patient carrying DEPDC5 truncating mutation failed to identify a definitive epileptogenic zone (Fig. 3 a-c). These evidences suggest that DEPDC5 germline mutations could play a role in non-lesional, refractory focal epilepsies with multiple independent epileptogenic foci or widespread epileptogenic networks (Ferri et al. 2017 ). According to this hypothesis the presence of germinal mutations in mTOR genes could represent a contraindication not only for surgery but possibly for presurgical invasive procedures (i.e. stereoEEG, corticography). However, given the association of DEPDC5 mutations also with FCD type I lesions (Baulac et al. 2015 ), in these non lesional cases it cannot be excluded the presence of multiple, diffuse subtle dysplastic areas missed by conventional brain MRI.
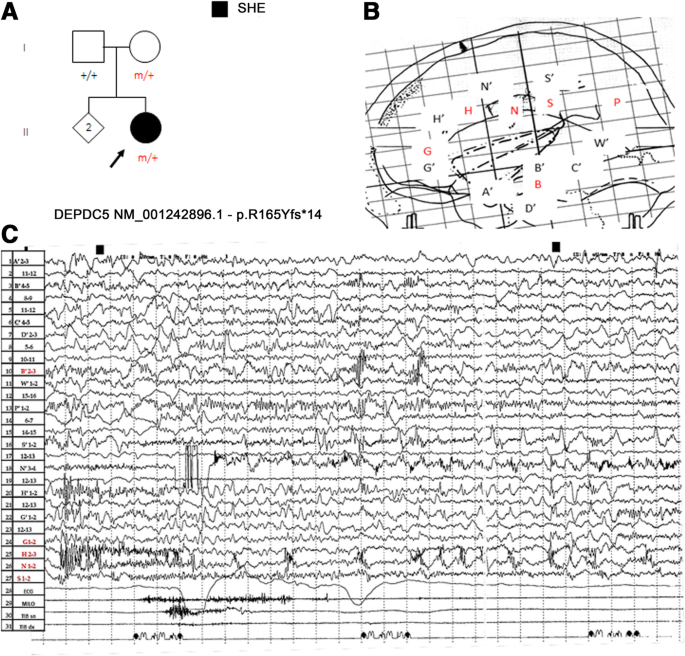
a Pedigree of a 57-year-old female with a frameshift mutation of DEPDC5, p.Arg165Tyrfs*14 inherited by her healthy mother. Previously published in Ferri et al. 2017 . b Stereotactic scheme of the patient who at age 43 years underwent stereoelectroencephalography (SEEG) study with bilateral limbic exploration extended to the inferior parietal lobe. The SEEG exploration shown (lateral view) included 15 intra-cerebral electrodes implanted mainly on the left. The EEG focus area was mainly explored by electrode H. Black letters with the accent (A’, B′, C′, D’, G’, H′, N′, S′, W′) indicates left side; red letters (B, G, H, N, S, P) indicated the right. c SEEG ictal recording showing fast polyspike activity over both the anterior-mid cingulate gyrus preceding a typical nocturnal hypermotor seizure, prevailing on right central-anterior cingulate cortex (H electrode). Note that interictal activity is recorded also in electrodes remote from ictal onset zone. Although the electrical pattern was suggestive for focal cortical dysplasia, tailored brain MRI was unrevealing
A novel missense mutation in the CABP4 gene encoding the neuronal Ca2 + −binding protein 4 (CaBP4) has been found in a Chinese family including 11 individuals diagnosed with ADSHE (Chen et al. 2017 ).
In a two-generation Australian family of Italian origin affected with SHE and ID, Hildebrand and coauthors identified by Whole Exome Sequencing (WES) analysis a homozygous mutation in PRIMA1 (Hildebrand et al. 2015 ). This gene encodes a transmembrane protein that anchors acetylcholinesterase (AChE), the enzyme hydrolyzing Ach to membrane rafts of neurons. The c.93 + 2 T > C mutation identified leads to knockout of PRIMA1, with reduction of AChE and accumulation of acetylcholine at the synapse, as shown in PRIMA1 knockout mice. The authors concluded that, similarly to the gain of function mutations of the genes coding for nAChR subunits, the enhanced cholinergic responses are the likely cause of severe SHE and intellectual disability in this family. However, apart from this single pedigree, this finding has not been replicated yet, as no other mutations were identified in a confirmation cohort of hundreds of SHE probands (Hildebrand et al. 2015 ).
Conclusions
SHE is a heterogeneous genetic syndrome, caused by genes involved in different molecular pathways. Despite a great effort to study the genetic background of SHE, a genetic cause may be recognizable in a very low percentage of sporadic cases and in less than 30% of ADSHE families, with incomplete penetrance (Kurahashi H et al., 2002 ). This is a cumulative estimate coming from studies of different case-series (principally families), each focused on the screening for mutations in a singular SHE gene. A systematic genetic characterization of a population of familial and sporadic patients diagnosed with SHE based on reliable diagnostic criteria is lacking.
Moreover, to date, there are no clear-cut correlations between disease severity, genetic findings and functional effects of the known genetic mutations (Tinuper et al. 2016 ) and further studies focused on genotype-phenotype correlations in SHE are needed. By now, KCNT1 gene mutated SHE patients seem to present a more severe form (Heron et al. 2012 ), whereas mutations in GATOR1-complex genes have been implicated in genetic-structural etiology of SHE.
Although at present genetic testing has limited usefulness in clinical practice, as genetics explain a minority of SHE patients, from a clinical perspective, analysis of SHE-related genes is worth even in isolated cases for whom a genetic aetiology is not primarily considered, because of possible implications for the diagnostic work-up and clinical management. In particular, detection of mutations in GATOR1-complex genes represent a red flag for FCDs, the most common potentially treatable architectural disorder underlying refractory FE. In non-lesional cases carrying pathogenic variants of GATOR1-complex genes, repeated and careful review of targeted, high-resolution imaging is needed to highlight subtle structural abnormalities susceptible of surgery. Moreover, the identification of pathogenic or possibly pathogenic mutations in GATOR1-complex genes, as well as in genes coding proteins acting upstream in the mTOR pathway could have important future therapeutic implications even in mutated patients considered not eligible for surgery. In fact, the development of a novel class of therapies based on mTOR inhibitors, whose prototype is rapamycin, will improve the treatment and prognosis of these patients. Both preclinical and clinical trials using mTOR inhibitors to treat epilepsy, and possibly prevent it, are currently underway (Citraro et al. 2016 ).
Availability of data and materials
Not applicable.
Abbreviations
Acetylcholinesterase
Autosomal dominant nocturnal frontal lobe epilepsy
Autosomal dominant sleep-related hypermotor epilepsy
Focal cortical dysplasia
Focal epilepsy
familial focal epilepsy with variable foci
mammalian Target Of Rapamycin
mammalian Target Of Rapamycin Complex1
neuronal nicotinic ACetylcholine Receptor
Nicotinamide adenine dinucleotide
- Nocturnal frontal lobe epilepsy
Nocturnal paroxysmal dystonia
Positron-emission tomography
Peroxisome proliferator-activated receptor alpha
Stereoelectroencephalography
- Sleep-related hypermotor epilepsy
Whole exome sequencing
Abdelnoura E, et al. Does age affect response to quinidine in patients with KCNT1 mutations? Report of three new cases and review of the literature. Seizure. 2018;55:1–3.
Article Google Scholar
Alanis-Guevara I, et al. Sleep disturbances, socioeconomic status, and seizure control as main predictors of quality of life in epilepsy. Epilepsy Behav. 2005;7:481–5.
Article CAS PubMed Google Scholar
Aridon P, et al. Increased sensitivity of the neuronal nicotinic receptor alpha 2 subunit causes familial epilepsy with nocturnal wandering and ictal fear. Am J Hum Genet. 2006;79:342–50.
Article CAS PubMed PubMed Central Google Scholar
Baldassari S, et al. The landscape of epilepsy-related GATOR1 variants. Genet Med. 2019;21(2):398–408.
Barcia G, et al. De novo gain-of-function KCNT1 channel mutations cause malignant migrating partial seizures of infancy. Nat Genet. 2012;44(11):1255–9.
Bar-Peled L, et al. A tumor suppressor complex with GAP activity for the rag GTPases that signal amino acid sufficiency to mTORC1. Science. 2013;340:1100–6.
Baulac S, et al. Familial focal epilepsy with focal cortical dysplasia due to DEPDC5 mutations. Ann Neurol. 2015;77:675–83.
Bertrand D, et al. How mutations in the nAChRs can cause ADNFLE epilepsy. Epilepsia. 2002;43(Suppl 5):112–22.
Bertrand D, et al. The CHRNB2 mutation I312M is associated with epilepsy and distinct memory deficits. Neurobiol Dis. 2005;20(3):799–804.
Bhattacharjee A, Kaczmarek LKJ. For K+ channels, Na+ is the new Ca2+. Trends Neurosci. 2005;28:422–8.
Bhattacharjee A, et al. Localization of the Slack potassium channel in the rat central nervous system. J Comp Neurol. 2002;454:241–54.
Bisulli F, et al. Increased frequency of arousal parasomnias in families with nocturnal frontal lobe epilepsy: a common mechanism? Epilepsia. 2010;51:1852–60.
Article PubMed Google Scholar
Brodtkorb E, Picard F. Tobacco habits modulate autosomal dominant nocturnal frontal lobe epilepsy. Epilepsy Behav. 2006;9:515–20.
Brown MR, et al. Amino-termini isoforms of the slack K+ channel, regulated by alternative promoters, differentially modulate rhythmic firing and adaptation. J Physiol Lond. 2008;586:5161–79.
Chen Y, et al. A novel mutation of the nicotinic acetylcholine receptor gene CHRNA4 in sporadic nocturnal frontal lobe epilepsy. Epilepsy Res. 2009;83(2–3):152–6.
Chen ZH, et al. Exome sequencing identified a novel missense mutation c.464G>a (p.G155D) in Ca2+−binding protein 4 (CABP4) in a Chinese pedigree with autosomal dominant nocturnal frontal lobe epilepsy. Oncotarget. 2017;8:78940–7.
PubMed PubMed Central Google Scholar
Cho YW, et al. A Korean kindred with autosomal dominant nocturnal frontal lobe epilepsy and mental retardation. Arch Neurol. 2003;60:1625–32.
Cho YW, et al. Autosomal dominant nocturnal frontal lobe epilepsy and mild memory impairment associated with CHRNB2 mutation I312M in the neuronal nicotinic acetylcholine receptor. Epilepsy Behav. 2008;13:361–5.
Citraro R, et al. mTOR pathway inhibition as a new therapeutic strategy in epilepsy and epileptogenesis. Pharmacol Res. 2016;107:333–43.
Combi R, et al. Two new putative susceptibility loci for ADNFLE. Brain Res. 2005;67:257–63.
Article CAS Google Scholar
Combi R, et al. Compound heterozygosity with dominance in the Corticotropin releasing hormone (CRH) promoter in a case of nocturnal frontal lobe epilepsy. J Sleep Res. 2008;17(3):361–2.
Conti V, et al. Nocturnal frontal lobe epilepsy with paroxysmal arousals due to CHRNA2 loss of function. Neurology. 2015;84(15):1520–8.
Coppola G, et al. Migrating partial seizures in infancy: a malignant disorder with developmental arrest. Epilepsia. 1995;36:1017–24.
De Fusco M, et al. The nicotinic receptor beta 2 subunit is mutant in nocturnal frontal lobe epilepsy. Nat Genet. 2000;26:275–6.
D'Gama AM, et al. Mammalian target of rapamycin pathway mutations cause hemimegalencephaly and focal cortical dysplasia. Ann Neurol. 2015;77(4):720–5.
Díaz-Otero F, et al. Autosomal dominant nocturnal frontal lobe epilepsy with a mutation in the CHRNB2 gene. Epilepsia. 2008;49(3):516–20.
Article PubMed CAS Google Scholar
Dibbens LM, et al. Mutations in DEPDC5 cause familial focal epilepsy with variable foci. Nat Genet. 2013;45:546–51.
Dobesberger J, et al. Successful surgical treatment of insular epilepsy with nocturnal hypermotor seizures. Epilepsia. 2008;49:159–62.
Fedi M, et al. Reduced striatal D1 receptor binding in autosomal dominant nocturnal frontal lobe epilepsy. Neurology. 2008;71:795–8.
Ferri L, et al. A stereo EEG study in a patient with sleep-related hypermotor epilepsy due to DEPDC5 mutation. Seizure. 2017;53:51–4.
Gibbs SA, et al. Sleep- related epileptic behaviors and non-REM-related parasomnias: insights from stereo-EEG. Sleep Med Rev. 2016;25:4–20.
Harvey AS, et al. Defining the spectrum of international practice in pediatric epilepsy surgery patients. Epilepsia. 2008;49:146–55.
Heron SE, et al. Missense mutations in the sodium-gated potassium channel gene KCNT1 cause severe autosomal dominant nocturnal frontal lobe epilepsy. Nat Genet. 2012;44:1188–90.
Hildebrand MS, et al. PRIMA1 mutation: a new cause of nocturnal frontal lobe epilepsy. Ann Clin Transl Neurol. 2015;2(8):821–30.
Hirose S, et al. A novel mutation of CHRNA4 responsible for autosomal dominant nocturnal frontal lobe epilepsy. Neurology. 1999;53(8):1749–53.
Hoda JC, et al. Human nocturnal frontal lobe epilepsy: pharmocogenomic profiles of pathogenic nicotinic acetylcholine receptor beta-subunit mutations outside the ion channel pore. Mol Pharmacol. 2008;74(2):379–91.
Ishida S, et al. Mutations of DEPDC5 cause autosomal dominant focal epilepsies. Nat Genet. 2013;45:552–5.
Kim GE, et al. Human slack potassium channel mutations increase positive cooperativity between individual channels. Cell Rep. 2014;9:1661–72.
Korenke GC, et al. Nocturnal frontal lobe epilepsy caused by a mutation in the GATOR1 complex gene NPRL3. Epilepsia. 2016;57(3):e60–3.
Kurahashi H, Hirose S. Autosomal Dominant Nocturnal Frontal Lobe Epilepsy. In: Pagon RA, Adam MP, Ardinger HH, Wallace SE, Amemiya A, Bean LJH, Bird TD, Ledbetter N, Mefford HC, Smith RJH, Stephens K, editors. GeneReviews®. Seattle: University of Washington Seattle; 2002. p. 1993–2017. Available from http://www.ncbi.nlm.nih.gov/books/NBK1169/ [Initial Posting: May 16, 2002; Last Update: March 15, 2018].
Lal D, et al. DEPDC5 mutations in genetic focal epilepsies of childhood. Ann Neurol. 2014;75:788–92.
Leniger T, et al. A new Chrna4 mutation with low penetrance in nocturnal frontal lobe epilepsy. Epilepsia. 2003;44(7):981–5.
Licchetta L, et al. Sleep-related hypermotor epilepsy: long-term outcome in a large cohort. Neurology. 2017;88(1):70–7.
Article PubMed PubMed Central Google Scholar
Liu H, et al. The identification of a novel mutation of nicotinic acetylcholine receptor gene CHRNB2 in a Chinese patient: its possible implication in non-familial nocturnal frontal lobe epilepsy. Epilepsy Res. 2011;95(1–2):94–9.
Lugaresi E, Cirignotta F. Hypnogenic paroxysmal dystonia: epileptic seizure or a new syndrome? Sleep. 1981;4(2):129–38.
Maccario M, Lustman LI. Paroxysmal nocturnal dystonia presenting as excessive daytime somnolence. Arch Neurol. 1990;47:291–4.
Marini C, Guerrini R. The role of the nicotinic acetylcholine receptors in sleep-related epilepsy. Biochem Pharmacol. 2007;74:1308–14.
Marsan E, et al. Depdc5 knockout rat: a novel model of mTORopathy. Neurobiol Dis. 2016;89:180–9.
Martin C, et al. A recurrent mutation in DEPDC5 predisposes to focal epilepsies in the French-Canadian population. Clin Genet. 2014;86:570–4.
McLellan A, et al. Phenotypic comparison of two Scottish families with mutations in different genes causing autosomal dominant nocturnal frontal lobe epilepsy. Epilepsia. 2003;44:613–7.
Menghi V, et al. Sleep-related hypermotor epilepsy: prevalence, impact and management strategies. Nat Sci Sleep. 2018;10:317–26.
Mikati MA, et al. Quinidine in the treatment of KCNT1-positive epilepsies. Ann Neurol. 2015;78(6):995–9.
Milligan CJ, et al. KCNT1 gain of function in 2 epilepsy phenotypes is reversed by quinidine. Ann Neurol. 2014;75:581–90.
Møller RS, et al. Mutations in KCNT1 cause a spectrum of focal epilepsies. Epilepsia. 2015;56(9):e114–20.
Article PubMed PubMed Central CAS Google Scholar
Montagna P. Nocturnal paroxysmal dystonia and nocturnal wandering. Neurology. 1992;42:61–7.
CAS PubMed Google Scholar
Montagna P, et al. Nocturnal epileptic seizures versus the arousal parasomnias. Somnologie. 2008;12:25–37.
Montavont A, et al. Hypermotor seizures in lateral and mesial parietal epilepsy. Epilepsy Behav. 2013;28:408–12.
Nakashima M, et al. Somatic mutations in the MTOR gene cause focal cortical dysplasia type IIb. Ann Neurol. 2015;78:375–86.
Naldi I, et al. Tobacco habits in nocturnal frontal lobe epilepsy. Epilepsy Behav. 2013;26:114–7.
Nguyen DK, et al. Revisiting the role of the insula in refractory partial epilepsy. Epilepsia. 2009;50:2599–604.
Nobili L, et al. Nocturnal frontal lobe epilepsy: intracerebral recordings of paroxysmal motor attacks with increasing complexity. Sleep. 2003;26:883–6.
PubMed Google Scholar
Nobili L, et al. Sleep-related hyperkinetic seizures of temporal lobe origin. Neurology. 2004;62:482–5.
Nobili L, et al. Surgical treatment of drug-resistant nocturnal frontal lobe epilepsy. Brain. 2007;130:561–73.
Nobili L, et al. Taylor’s focal cortical dysplasia increases the risk of sleep-related epilepsy. Epilepsia. 2009;50:2599–604.
Ohba C, et al. De novo KCNT1 mutations in early-onset epileptic encephalopathy. Epilepsia. 2015;56(9):e121–8.
Oldani A, et al. Autosomal dominant nocturnal frontal lobe epilepsy: a video-polysomnographic and genetic appraisal of 40 patients and delineation of the epileptic syndrome. Brain. 1998;121:205–23.
Peled R, Lavie P. Paroxysmal awakenings from sleep associated with excessive daytime somnolence: a form of nocturnal epilepsy. Neurology. 1986;36:95–8.
Phillips HA, et al. Localization of a gene for autosomal dominant nocturnal frontal lobe epilepsy to chromosome 20q13.2. Nat Genet. 1995;10:117–8.
Phillips HA, et al. A de novo mutation in sporadic nocturnal frontal lobe epilepsy. Ann Neurol. 2000;48(2):264–7.
Phillips HA, et al. CHRNB2 is the second acetylcholine receptor subunit associated with autosomal dominant nocturnal frontal lobe epilepsy. Am J Hum Genet. 2001;68(1):225–31.
Picard F, et al. Alteration of the in vivo nicotinic receptor density in ADNFLE patients: a PET study. Brain. 2006;129:2047–60.
Picard F, et al. DEPDC5 mutations in families presenting as autosomal dominant nocturnal frontal lobe epilepsy. Neurology. 2014;82:2101–6.
Proserpio P, et al. Insular-opercular seizures manifesting with sleep-related paroxysmal motor behaviors: a stereo-EEG study. Epilepsia. 2011;52:1781–91.
Provini F, et al. Nocturnal frontal lobe epilepsy. A clinical and polygraphic overview of 100 consecutive cases. Brain. 1999;122:1017–31.
Puligheddu M, et al. Rationale for an adjunctive therapy with fenofibrate in pharmacoresistant nocturnal frontal lobe epilepsy. Epilepsia. 2017;58:1762–70.
Rheims S, et al. Analysis of clinical patterns and underlying epileptogenic zones of hypermotor seizures. Epilepsia. 2008;49:2030–40.
Ricos MG, et al. Mutations in the mammalian target of rapamycin pathway regulators NPRL2 and NPRL3 cause focal epilepsy. Ann Neurol. 2016;79:120–31.
Rizzo F, et al. Characterization of two de novo KCNT1 mutations in children with malignant migrating partial seizures in infancy. Mol Cell Neurosci. 2016;72:54–63.
Rozycka A, et al. Evidence for S284L mutation of the CHRNA4 in a white family with autosomal dominant nocturnal frontal lobe epilepsy. Epilepsia. 2003;44(8):1113–7.
Rubboli G, et al. Mild malformations of cortical development insleep-related hypermotor epilepsy due to KCNT1 mutations. Ann Clin Transl Neurol. 2018;6(2):386–91.
Ryvlin P, Minotti L, Demarquay G, et al. Nocturnal hypermotor seizures, suggesting frontal lobe epilepsy, can originate in the insula. Epilepsia. 2006;47:755–65.
Sáenz A, et al. Autosomal dominant nocturnal frontal lobe epilepsy in a Spanish family with a Ser252Phe mutation in the CHRNA4 gene. Arch Neurol. 1999;56(8):1004–9.
Sansoni V, et al. A de novo mutation in an Italian sporadic patient affected by nocturnal frontal lobe epilepsy. J Sleep Res. 2012;21:352–3.
Sansoni V, et al. Functional characterization of a CRH missense mutation identified in an ADNFLE family. PLoS One. 2013;8(4):e61306.
Scerri T, et al. Familial cortical dysplasia type IIA caused by a germline mutation in DEPDC5. Ann Clin Transl Neurol. 2015;2(5):575–80.
Scheffer IE, et al. Autosomal dominant frontal epilepsy misdiagnosed as sleep disorder. Lancet. 1994;343:515–7.
Scheffer IE, et al. Mutations in mammalian target of rapamycin regulator DEPDC5 cause focal epilepsy with brain malformations. Ann Neurol. 2014;75:782–7.
Schwalen S, Jorg J. Day-time fatigue in frontal lobe epilepsy with primarily sleep-related seizures. A case report. Nervenarzt. 1998;69:166–70.
Shimada S, et al. A novel KCNT1 mutation in a Japanese patient with epilepsy of infancy with migrating focal seizures. Hum Genome Var. 2014;1:14027.
Steinlein OK. Genetic heterogeneity in familial nocturnal frontal lobe epilepsy. Prog Brain Res. 2014;213:1–15.
Steinlein OK, et al. A missense mutation in the neuronal nicotinic acetylcholine receptor α4 subunit is associated with autosomal dominant nocturnal frontal lobe epilepsy. Nat Genet. 1995;11:201–3.
Steinlein OK, et al. An insertion mutation of the CHRNA4 gene in a family with autosomal dominant nocturnal frontal lobe epilepsy. Hum Mol Genet. 1997;6(6):943–7.
Steinlein OK, et al. Independent occurrence of the CHRNA4 Ser248Phe mutation in a Norwegian family with nocturnal frontal lobe epilepsy. Epilepsia. 2000;41(5):529–35.
Steinlein OK, et al. Mutations in familial nocturnal frontal lobe epilepsy might be associated with distinct neurological phenotypes. Seizure. 2012;21:118–23.
Terzaghi M, et al. Coupling of minor motor events and epileptiform discharges with arousal fluctuations in NFLE. Epilepsia. 2008;49:670–6.
Tinuper P, Bisulli F. From nocturnal frontal lobe epilepsy to sleep-related Hypermotor epilepsy: a 35-year diagnostic challenge. Seizure. 2017;44:87–92.
Tinuper P, et al. Nocturnal paroxysmal dystonia with short-lasting attacks: three cases with evidence for an epileptic frontal lobe origin of seizures. Epilepsia. 1990;31(5):549–56.
Tinuper P, et al. Definition and diagnostic criteria of sleep-related hypermotor epilepsy. Neurology. 2016;86:1834–42.
van Kranenburg M, et al. Preliminary functional assessment and classification of DEPDC5 variants associated with focal epilepsy. Hum Mutat. 2015;36(2):200–9.
Vaugier L, et al. Neural networks underlying hyperkinetic seizures of “temporal lobe” origin. Epilepsy Res. 2009;86:200–8.
Vignatelli L, et al. Excessive daytime sleepiness and subjective sleep quality in patients with nocturnal frontal lobe epilepsy: a case-control study. Epilepsia. 2006;47(suppl 5):73–7.
Vignatelli L, et al. Prevalence of nocturnal frontal lobe epilepsy in the adult population of Bologna and Modena, Emilia-Romagna region, Italy. Sleep. 2015;38:479–85.
Vignatelli L, et al. Prevalence of nocturnal frontal lobe epilepsy in the adult population of Bologna and Modena, Emilia-Romagna region. Italy Sleep. 2017;1(2):40.
Google Scholar
Wang MY, et al. A novel mutation of the nicotinic acetylcholine receptor gene CHRNA4 in a Chinese patient with non-familial nocturnal frontal lobe epilepsy. Epilepsy Res. 2014;108(10):1927–31.
Waterman K, et al. An epileptic syndrome caused by mesial frontal lobe seizure foci. Neurology. 1987;37(4):577–82.
Wiebe S, et al. A randomized, controlled trial of surgery for temporal-lobe epilepsy. N Engl J Med. 2001;345:311–8.
Williamson PD, et al. Complex partial seizures of frontal lobe origin. Ann Neurol. 1985;18(4):497–504.
Willoughby JO, et al. Nicotine as an antiepileptic agent in ADNFLE: an N-of-one study. Epilepsia. 2003;44(9):1238–40.
Zucconi M, et al. The macrostructure and microstructure of sleep in patients with autosomal dominant nocturnal frontal lobe epilepsy. J Clin Neurophysiol. 2000;17:77–86.
Download references
Acknowledgements
We would like to acknowledge all our collaborators of Bellaria Hospital, Bologna, especially Dr. Federica Pondrelli and Dr. Toni Francesco. We are also grateful to all the EEG technicians of our staff, in particular Francesco Mignani.
Author information
Authors and affiliations.
IRCCS Istituto delle Scienze Neurologiche di Bologna, Bellaria Hospital, Via Altura 3, Bologna, Italy
Francesca Bisulli, Laura Licchetta & Paolo Tinuper
Department of Biomedical and Neuromotor Sciences (DIBINEM), University of Bologna, Bologna, Italy
You can also search for this author in PubMed Google Scholar
Contributions
BF drafted the work and substantively revised it; LL drafted the work; TP revised the work. All authors read and approved the final manuscript.
Corresponding author
Correspondence to Francesca Bisulli .
Ethics declarations
Ethics approval and consent to participate, consent for publication, competing interests.
The authors declare that they have no competing interests.
Additional information
Publisher’s note.
Springer Nature remains neutral with regard to jurisdictional claims in published maps and institutional affiliations.
Rights and permissions
Open Access This article is distributed under the terms of the Creative Commons Attribution 4.0 International License ( http://creativecommons.org/licenses/by/4.0/ ), which permits unrestricted use, distribution, and reproduction in any medium, provided you give appropriate credit to the original author(s) and the source, provide a link to the Creative Commons license, and indicate if changes were made. The Creative Commons Public Domain Dedication waiver ( http://creativecommons.org/publicdomain/zero/1.0/ ) applies to the data made available in this article, unless otherwise stated.
Reprints and permissions
About this article
Cite this article.
Bisulli, F., Licchetta, L. & Tinuper, P. Sleep related hyper motor epilepsy (SHE): a unique syndrome with heterogeneous genetic etiologies. Sleep Science Practice 3 , 3 (2019). https://doi.org/10.1186/s41606-019-0035-5
Download citation
Received : 25 March 2019
Accepted : 24 June 2019
Published : 17 July 2019
DOI : https://doi.org/10.1186/s41606-019-0035-5
Share this article
Anyone you share the following link with will be able to read this content:
Sorry, a shareable link is not currently available for this article.
Provided by the Springer Nature SharedIt content-sharing initiative
- Genetics; structural-genetic
Sleep Science and Practice
ISSN: 2398-2683
- General enquiries: [email protected]
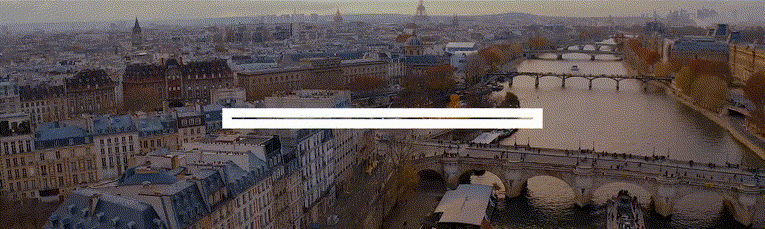
IMAGES
VIDEO
COMMENTS
Wandering in diseases classified elsewhere. Z91.83 is a billable/specific ICD-10-CM code that can be used to indicate a diagnosis for reimbursement purposes. The 2024 edition of ICD-10-CM Z91.83 became effective on October 1, 2023. This is the American ICD-10-CM version of Z91.83 - other international versions of ICD-10 Z91.83 may differ.
G47.8 is a billable/specific ICD-10-CM code that can be used to indicate a diagnosis for reimbursement purposes. The 2024 edition of ICD-10-CM G47.8 became effective on October 1, 2023. This is the American ICD-10-CM version of G47.8 - other international versions of ICD-10 G47.8 may differ. Convert G47.8 to ICD-9-CM.
Abstract. The syndrome known as nocturnal frontal lobe epilepsy is recognized worldwide and has been studied in a wide range of clinical and scientific settings (epilepsy, sleep medicine, neurosurgery, pediatric neurology, epidemiology, genetics). Though uncommon, it is of considerable interest to practicing neurologists because of complexity ...
Sleep disorder, unspecified. G47.9 is a billable/specific ICD-10-CM code that can be used to indicate a diagnosis for reimbursement purposes. The 2024 edition of ICD-10-CM G47.9 became effective on October 1, 2023. This is the American ICD-10-CM version of G47.9 - other international versions of ICD-10 G47.9 may differ.
Z91.83 is a billable diagnosis code used to specify a medical diagnosis of wandering in diseases classified elsewhere. The code is valid during the current fiscal year for the submission of HIPAA-covered transactions from October 01, 2023 through September 30, 2024. The code is exempt from present on admission (POA) reporting for inpatient ...
As noted by the Ohayon et al. [1], nocturnal wandering (NW) is not synonymous with sleepwalking. NW may also refer to wandering during the night due to epilepsy. Alcohol intoxication is well known to result in drunken behavior while awake but this type of cognitive impairment may be undistinguishable from other forms of NW.
Episodic Nocturnal Wandering (ENW): Preceded by both PA and NPD, episodic nocturnal wandering results in agitated wandering outside the bed. These episodes are the rarest nocturnal seizure subtype, only occurring in ~2% of nocturnal seizures. There may be some symptoms resembling dysautonomia with screaming, yelling, or talking and there still ...
The lifetime prevalence of nocturnal wandering with abnormal state of consciousness among US adults may be as high as 29.2%. ; however, the prevalence of sleepwalking disorder, marked by repeated episodes and impairment or distress, is much lower (1-5%). In Sweden, the incidence of quiet sleepwalking is reported as 40%, with a yearly prevalence ...
Z91.83. Z91.83 is a valid billable ICD-10 diagnosis code for Wandering in diseases classified elsewhere . It is found in the 2024 version of the ICD-10 Clinical Modification (CM) and can be used in all HIPAA-covered transactions from Oct 01, 2023 - Sep 30, 2024 . Z91.83 is exempt from POA reporting ( Present On Admission).
ICD-10-CM Codes. Factors influencing health status and contact with health services. Persons with potential health hazards related to family and personal history and certain conditions influencing health status. Personal risk factors, not elsewhere classified (Z91) Wandering in diseases classified elsewhere (Z91.83) Z91.82.
ICD-10-CM Diagnosis Codes. Z91.83 - Wandering in diseases classified elsewhere. The above description is abbreviated. This code description may also have Includes, Excludes, Notes, Guidelines, Examples and other information. Access to this feature is available in the following products: Find-A-Code Essentials. HCC Plus.
The American Psychiatric Association classifies sleepwalking as a mental illness if, in addition to the ICD-10 CM characteristics, the events cause clinically significant distress or impairment in social, ... as a proxy measure of nocturnal wandering. Immediate parent-report relies on the child being observable to parents. Self-report relies on ...
Ohayon M, Mahowald M, Dauvilliers Y, Krystal A, Leger D. Prevalence and comorbidity of nocturnal wandering in the US adult general population. ... ICD-9-CM Code: 327.41 ICD-10-CM Code: G47.51 Diagnostic Criteria Criteria A-C must be met A. The disorder meets general criteria for a NREM disorder of arousal.
Patient with nocturnal events; referral diagnosis is "atypical night terrors." ... suggests "episodic nocturnal wandering" of epileptic origin more than bona fide somnambulism as a parasomnia. 6. ... and poor field coverage with the international 10-20 system. In addition, the EEG can be frequently obscured by muscle artifact. Moreover ...
The ICD-10-CM code Z91.83 (wandering in diseases classified elsewhere) helps document wandering and to prompt important discussions about safety among caregivers, individuals, and providers. This code is not linked to a specific diagnosis, nor is it part of the diagnostic codes used for ASD or intellectual disabilities. Wandering should be ...
For instance, some groups have focused more on the emotional components of the syndrome, some more on the increased nocturnal locomotor activity such as wandering, whereas fewer have described sundowning as primarily a sleep-related disturbance (Boronat et al., 2019). It is also important to note that sundowning is not an official diagnosis (it ...
Nocturnal frontal lobe epilepsy (NFLE) is a syndrome of heterogeneous etiology, characterized by the occurrence of sleep-related seizures with different complexity and duration. Genetic, lesional, and cryptogenetic NFLE forms have been described. NFLE is generally considered a benign clinical entity, although severe, drug-resistant forms do exist. A significant proportion of sleep-related ...
Sleep-related hypermotor epilepsy (SHE), formerly known as Nocturnal Frontal Lobe Epilepsy is a focal epilepsy characterized by seizures with complex hyperkinetic automatisms and/or asymmetric tonic/dystonic posturing occurring mostly during sleep. SHE is a rare disease with an estimated minimum prevalence of 1.8/100,000 individuals and represent about 10% of drug-resistant surgical cases ...
14 result found: ICD-10-CM Diagnosis Code Z91.83 [convert to ICD-9-CM] Wandering in diseases classified elsewhere. Wandering associated w a mental disorder; Wandering due to mental disorder; underlying disorder such as:; Alzheimer's disease (G30.-); autism or pervasive developmental disorder (F84.-); intellectual disabilities (F70-F79 ...
The 2024 edition of ICD-10-CM F03.91 became effective on October 1, 2023. This is the American ICD-10-CM version of F03.91 - other international versions of ICD-10 F03.91 may differ. The following code(s) above F03.91 contain annotation back-references
Search All ICD-10 Toggle Dropdown. Search All ICD-10; ICD-10-CM Diagnosis Codes; ICD-10-PCS Procedure Codes; ICD-10-CM Diagnosis Index; ICD-10-CM External Causes Index; ICD-10-CM Table of Drugs; ICD-10-CM Table of Neoplasms; HCPCS Codes; ICD-9-CM Diagnosis Codes; ICD-9-Vol-3 Procedure Code; Search All Data