- Share full article
Advertisement
Supported by
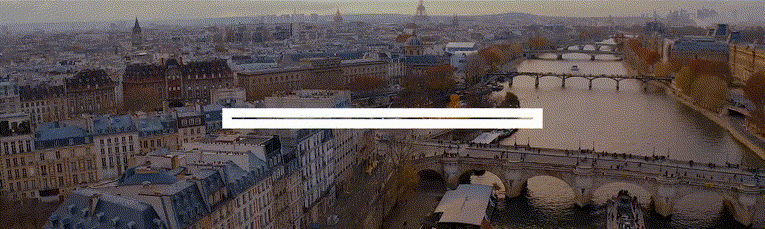
The Future of Space Tourism Is Now. Well, Not Quite.
From zero-pressure balloon trips to astronaut boot camps, reservations for getting off the planet — or pretending to — are skyrocketing. The prices, however, are still out of this world.

By Debra Kamin
Ilida Alvarez has dreamed of traveling to space since she was a child. But Ms. Alvarez, a legal-mediation firm owner, is afraid of flying, and she isn’t a billionaire — two facts that she was sure, until just a few weeks ago, would keep her fantasy as out of reach as the stars. She was wrong.
Ms. Alvarez, 46, and her husband, Rafael Landestoy, recently booked a flight on a 10-person pressurized capsule that — attached to a massive helium-filled balloon — will gently float to 100,000 feet while passengers sip champagne and recline in ergonomic chairs. The reservation required a $500 deposit; the flight itself will cost $50,000 and last six to 12 hours.
“I feel like it was tailor-made for the chickens like me who don’t want to get on a rocket,” said Ms. Alvarez, whose flight, organized by a company called World View , is scheduled to depart from the Grand Canyon in 2024.
Less than a year after Jeff Bezos and Richard Branson kicked off a commercial space race by blasting into the upper atmosphere within weeks of each other last summer, the global space tourism market is skyrocketing, with dozens of companies now offering reservations for everything from zero-pressure balloon trips to astronaut boot camps and simulated zero-gravity flights. But don’t don your spacesuit just yet. While the financial services company UBS estimates the space travel market will be worth $3 billion by 2030, the Federal Aviation Administration has yet to approve most out-of-this-world trips, and construction has not started on the first space hotel. And while access and options — not to mention launchpads — are burgeoning, space tourism remains astronomically expensive for most.
First, what counts as space travel?
Sixty miles (about 100 kilometers) above our heads lies the Kármán line, the widely accepted aeronautical boundary of the earth’s atmosphere. It’s the boundary used by the Féderátion Aéronautique Internationale, which certifies and controls global astronautical records. But many organizations in the United States, including the F.A.A. and NASA, define everything above 50 miles to be space.
Much of the attention has been focused on a trio of billionaire-led rocket companies: Mr. Bezos’ Blue Origin , whose passengers have included William Shatner; Mr. Branson’s Virgin Galactic , where tickets for a suborbital spaceflight start at $450,000; and Elon Musk’s SpaceX , which in September launched an all-civilian spaceflight, with no trained astronauts on board. Mr. Branson’s inaugural Virgin Galactic flight in 2021 reached about 53 miles, while Blue Origin flies above the 62-mile mark. Both are eclipsed by SpaceX, whose rockets charge far deeper in to the cosmos, reaching more than 120 miles above Earth.
Balloons, like those operated by World View, don’t go nearly as high. But even at their maximum altitude of 18 or 19 miles, operators say they float high enough to show travelers the curvature of the planet, and give them a chance to experience the overview effect — an intense perspective shift that many astronauts say kicks in when you view Earth from above.
Now, how to get there …
Blue Origin and Virgin Galactic, which are both licensed for passenger space travel by the F.A.A., are open for ticket sales. (Blue Origin remains mum on pricing.) Both companies currently have hundreds or even thousands of earthlings on their wait lists for a whirl to the edge of space. SpaceX charges tens of millions of dollars for its further-reaching flights and is building a new facility in Texas that is currently under F.A.A. review.
Craig Curran is a major space enthusiast — he’s held a reserved seat on a Virgin Galactic flight since 2011 — and the owner of Deprez Travel in Rochester, N.Y. The travel agency has a special space travel arm, Galactic Experiences by Deprez , through which Mr. Curran sells everything from rocket launch tickets to astronaut training.
Sales in the space tourism space, Mr. Curran acknowledges, “are reasonably difficult to make,” and mostly come from peer-to-peer networking. “You can imagine that people who spend $450,000 to go to space probably operate in circles that are not the same as yours and mine,” he said.
Some of Mr. Curran’s most popular offerings include flights where you can experience the same stomach-dropping feeling of zero gravity that astronauts feel in space, which he arranges for clients via chartered, specialized Boeing 727s that are flown in parabolic arcs to mimic being in space. Operators including Zero G also offer the service; the cost is around $8,200.
You can almost count the number of completed space tourist launches on one hand — Blue Origin has had four; SpaceX, two. Virgin Galactic, meanwhile, on Thursday announced the launch of its commercial passenger service, previously scheduled for late 2022, was delayed until early 2023. Many of those on waiting lists are biding their time before blastoff by signing up for training. Axiom Space, which contracts with SpaceX, currently offers NASA-partnered training at Houston’s Johnson Space Center. Virgin Galactic, which already offers a “customized Future Astronaut Readiness program” at its Spaceport America facility in New Mexico, is also partnering with NASA to build a training program for private astronauts.
Would-be space tourists should not expect the rigor that NASA astronauts face. Training for Virgin Galactic’s three-hour trips is included in the cost of a ticket and lasts a handful of days; it includes pilot briefings and being “fitted for your bespoke Under Armour spacesuit and boots,” according to its website.
Not ready for a rocket? Balloon rides offer a less hair-raising celestial experience.
“We go to space at 12 miles an hour, which means that it’s very smooth and very gentle. You’re not rocketing away from earth,” said Jane Poynter, a co-founder and co-chief executive of Space Perspective , which is readying its own touristic balloon spaceship, Spaceship Neptune. If all goes according to plan, voyages are scheduled to begin departing from Florida in 2024, at a cost of $125,000 per person. That’s a fraction of the price tag for Blue Origin and Virgin Galactic, but still more than double the average annual salary of an American worker.
Neither Space Perspective nor World View has the required approval yet from the F.A.A. to operate flights.
Unique implications
Whether a capsule or a rocket is your transport, the travel insurance company battleface launched a civilian space insurance plan in late 2021, a direct response, said chief executive Sasha Gainullin, to an increase in space tourism interest and infrastructure. Benefits include accidental death and permanent disablement in space and are valid for spaceflights on operators like SpaceX, Blue Origin and Virgin Galactic, as well as on stratospheric balloon rides. They’ve had many inquiries, Mr. Gainullin said, but no purchases just yet.
“Right now it’s such high-net-worth individuals who are traveling to space, so they probably don’t need insurance,” he said. “But for quote-unquote regular travelers, I think we’ll see some takeups soon.”
And as the industry grows, so perhaps will space travel’s impact on the environment. Not only do rocket launches have immense carbon footprints, even some stratospheric balloon flights have potentially significant implications: World View’s balloons are powered by thousands of cubic meters of helium, which is a limited resource . But Ted Parson, a professor of environmental law at the University of California, Los Angeles, said that space travel’s environmental impact is still dwarfed by civil aviation. And because space travel is ultra-niche, he believes it’s likely to stay that way.
“Despite extensive projections, space tourism is likely to remain a tiny fraction of commercial space exploration,” he said. “It reminds me of tourism on Mt. Everest. It’s the indulgence of very rich people seeking a transcendent, once-in-a-lifetime experience, and the local environmental burden is intense.”
Stay a while?
In the future, space enthusiasts insist, travelers won’t be traveling to space just for the ride. They’ll want to stay a while. Orbital Assembly Corporation, a manufacturing company whose goal is to colonize space, is currently building the world’s first space hotels — two ring-shaped properties that will orbit Earth, called Pioneer Station and Voyager Station. The company, quite optimistically, projects an opening date of 2025 for Pioneer Station, with a capacity of 28 guests. The design for the larger Voyager Station , which they say will open in 2027, promises villas and suites, as well as a gym, restaurant and bar. Both provide the ultimate luxury: simulated gravity. Axiom Space , a space infrastructure company, is currently building the world’s first private space station; plans include Philippe Starck-designed accommodations for travelers to spend the night.
Joshua Bush, chief executive of travel agency Avenue Two Travel , has sold a handful of seats on upcoming Virgin Galactic flights to customers. The market for space travel (and the sky-high prices that come with it), he believes, will evolve much like civilian air travel did.
“In the beginning of the 20th century, only very affluent people could afford to fly,” he said. “Just as we have Spirit and Southwest Airlines today, there will be some sort of equivalent of that in space travel, too. Hopefully within my lifetime.”

52 Places for a Changed World
The 2022 list highlights places around the globe where travelers can be part of the solution.
Follow New York Times Travel on Instagram , Twitter and Facebook . And sign up for our weekly Travel Dispatch newsletter to receive expert tips on traveling smarter and inspiration for your next vacation. Dreaming up a future getaway or just armchair traveling? Check out our 52 Places for a Changed World for 2022.
What’s Up in Space and Astronomy
Keep track of things going on in our solar system and all around the universe..
Never miss an eclipse, a meteor shower, a rocket launch or any other 2024 event that’s out of this world with our space and astronomy calendar .
Scientists may have discovered a major flaw in their understanding of dark energy, a mysterious cosmic force . That could be good news for the fate of the universe.
A new set of computer simulations, which take into account the effects of stars moving past our solar system, has effectively made it harder to predict Earth’s future and reconstruct its past.
Dante Lauretta, the planetary scientist who led the OSIRIS-REx mission to retrieve a handful of space dust , discusses his next final frontier.
A nova named T Coronae Borealis lit up the night about 80 years ago. Astronomers say it’s expected to put on another show in the coming months.
Is Pluto a planet? And what is a planet, anyway? Test your knowledge here .
Five reasons to explore Mars
Subscribe to the center for technology innovation newsletter, darrell m. west darrell m. west senior fellow - center for technology innovation , douglas dillon chair in governmental studies.
August 18, 2020
The recent launch of the Mars rover Perseverance is the latest U.S. space mission seeking to understand our solar system. Its expected arrival at the Red Planet in mid-February 2021 has a number of objectives linked to science and innovation. The rover is equipped with sophisticated instruments designed to search for the remains of ancient microbial life, take pictures and videos of rocks, drill for soil and rock samples, and use a small helicopter to fly around the Jezero Crater landing spot .
Mars is a valuable place for exploration because it can be reached in 6 ½ months, is a major opportunity for scientific exploration, and has been mapped and studied for several decades. The mission represents the first step in a long-term effort to bring Martian samples back to Earth, where they can be analyzed for residues of microbial life. Beyond the study of life itself, there are a number of different benefits of Mars exploration.
Understand the Origins and Ubiquity of Life
The site where Perseverance is expected to land is the place where experts believe 3.5 billion years ago held a lake filled with water and flowing rivers. It is an ideal place to search for the residues of microbial life, test new technologies, and lay the groundwork for human exploration down the road.
The mission plans to investigate whether microbial life existed on Mars billions of years ago and therefore that life is not unique to Planet Earth. As noted by Chris McKay, a research scientist at NASA’s Ames Research Science Center, that would be an extraordinary discovery. “Right here in our solar system, if life started twice , that tells us some amazing things about our universe,” he pointed out. “It means the universe is full of life. Life becomes a natural feature of the universe, not just a quirk of this odd little planet around this star.”
The question of the origins of life and its ubiquity around the universe is central to science, religion, and philosophy. For much of our existence, humans have assumed that even primitive life was unique to Planet Earth and not present in the rest of the solar system, let alone the universe. We have constructed elaborate religious and philosophical narratives around this assumption and built our identity along the notion that life is unique to Earth.
If, as many scientists expect, future space missions cast doubt on that assumption or outright disprove it by finding remnants of microbial life on other planets, it will be both invigorating and illusion-shattering. It will force humans to confront their own myths and consider alternative narratives about the universe and the place of Earth in the overall scheme of things.
Related Books
Darrell M. West
October 18, 2016
Darrell M. West, John R. Allen
July 28, 2020
As noted in my Brookings book, Megachange , given the centrality of these issues for fundamental questions about human existence and the meaning of life, it would represent a far-reaching shift in existing human paradigms. As argued by scientist McKay, discovering evidence of ancient microbial life on Mars would lead experts to conclude that life likely is ubiquitous around the universe and not limited to Planet Earth. Humans would have to construct new theories about ourselves and our place in the universe.
Develop New Technologies
The U.S. space program has been an extraordinary catalyst for technology innovation . Everything from Global Positioning Systems and medical diagnostic tools to wireless technology and camera phones owe at least part of their creation to the space program. Space exploration required the National Aeronautics and Space Administration to learn how to communicate across wide distances, develop precise navigational tools, store, transmit, and process large amounts of data, deal with health issues through digital imaging and telemedicine, and develop collaborative tools that link scientists around the world. The space program has pioneered the miniaturization of scientific equipment and helped engineers figure out how to land and maneuver a rover from millions of miles away.
Going to Mars requires similar inventiveness. Scientists have had to figure out how to search for life in ancient rocks, drill for rock samples, take high resolution videos, develop flying machines in a place with gravity that is 40 percent lower than on Earth, send detailed information back to Earth in a timely manner, and take off from another planet. In the future, we should expect large payoffs in commercial developments from Mars exploration and advances that bring new conveniences and inventions to people.
Encourage Space Tourism
In the not too distant future, wealthy tourists likely will take trips around the Earth, visit space stations, orbit the Moon, and perhaps even take trips around Mars. For a substantial fee, they can experience weightlessness, take in the views of the entire planet, see the stars from outside the Earth’s atmosphere, and witness the wonders of other celestial bodies.
The Mars program will help with space tourism by improving engineering expertise with space docking, launches, and reentry and providing additional experience about the impact of space travel on the human body. Figuring out how weightlessness and low gravity situations alter human performance and how space radiation affects people represent just a couple areas where there are likely to be positive by-products for future travel.
The advent of space tourism will broaden human horizons in the same way international travel has exposed people to other lands and perspectives. It will show them that the Earth has a delicate ecosystem that deserves protecting and why it is important for people of differing countries to work together to solve global problems. Astronauts who have had this experience say it has altered their viewpoints and had a profound impact on their way of thinking.
Facilitate Space Mining
Many objects around the solar system are made of similar minerals and chemical compounds that exist on Earth. That means that some asteroids, moons, and planets could be rich in minerals and rare elements. Figuring out how to harvest those materials in a safe and responsible manner and bring them back to Earth represents a possible benefit of space exploration. Elements that are rare on Earth may exist elsewhere, and that could open new avenues for manufacturing, product design, and resource distribution. This mission could help resource utilization through advances gained with its Mars Oxygen Experiment (MOXIE) equipment that converts Martian carbon dioxide into oxygen. If MOXIE works as intended, it would help humans live and work on the Red Planet.
Advance Science
One of the most crucial features of humanity is our curiosity about the life, the universe, and how things operate. Exploring space provides a means to satisfy our thirst for knowledge and improve our understanding of ourselves and our place in the universe.
Space travel already has exploded centuries-old myths and promises to continue to confront our long-held assumptions about who we are and where we come from. The next decade promises to be an exciting period as scientists mine new data from space telescopes, space travel, and robotic exploration. Ten or twenty years from now, we may have answers to basic questions that have eluded humans for centuries, such as how ubiquitous life is outside of Earth, whether it is possible for humans to survive on other planets, and how planets evolve over time.
The author would like to thank Victoria E. Hamilton, staff scientist at the Southwest Research Institute, for her helpful feedback on this blog post.
Related Content
May 13, 2014
Hillary Schaub
May 16, 2014
February 16, 2021
Space Exploration
Governance Studies
Center for Technology Innovation
Online Only
2:00 pm - 3:00 pm EDT
Darrell M. West, Marina Koren
March 25, 2024
Darrell M. West, Tom Colvin, Regina Ta
August 28, 2023
Mars Attracts: Space Tourism Becomes The Final Fun-tier
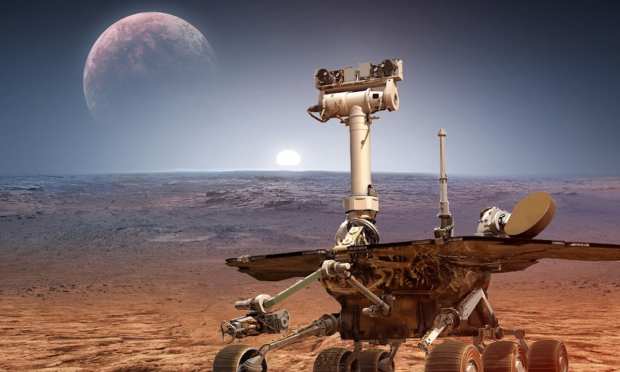
Restrictions continue to put a chill on world travel, cutting off popular places on maps we’re all familiar with. But what about places and travel experiences of the third kind ?
We’re talking about boldly going where no tourist has gone before. Space, the final frolicking frontier. Encouraged that not one but three spacecraft put aloft by as many nations are at this very moment casing the Red Planet for its future potential as an extra Earth (one never knows), it’s shining a laser light on off-world experiences being quickly commercialized.
We’re getting there, step by step. Headlines blared congrats on Feb. 18 as NASA’s Perseverance robotic rover landed safely on Mars — America’s sixth Mars landing — even as China’s Tianwen-1 probe and the United Arab Emirates spacecraft Hope took up orbit above.
In other words, Mars is getting crowded already, like The Hamptons. But at least when “Mars Hotel” finally opens (as foretold in 1974 by The Grateful Dead) there’s already a way to pay.
Marscoin is a new cryptocurrency fielded by The Mars Society to not only help fund missions, but also to act as the new world’s reserve currency. Sounds far out, but it’s just the payments business looking for new opportunities. And, as the Marscoin folks say , for “a new planetary settlement, a payment and value transfer/storage system such as bitcoin would be useful, first and foremost. It is very unlikely that the first Martian settlers will start printing paper.”
Don’t be so sure. Paper checks can survive in a vacuum.
Since there are no “Fifth Element”-style space pleasure cruises to Mars (yet), let’s look at some of the near-Earth activities that are now available (or soon will be) to zero-gravity thrill-seekers.
Near-Space Tourism Is Taking Off
Planetary forces like international commerce and the obsessive need to post the best vacation photos on Instagram are combining to break the surly bonds of earth.
In 2021, there are at least two companies testing high-altitude balloon rides to the edge of space. Based out of Florida’s Kennedy Space Center, Space Perspective just notched $7 million in seed financing to get its Spaceship Neptune project ( ahem ) off the ground.
Founder and Co-CEO Taber MacCallum said in a December statement that “our ability to make space accessible in unprecedented ways carries immense importance. We are grateful that this premier group of investors recognizes that space tourism has arrived and is a significant driver in the future growth of commercial spaceflight.”
And there it is: space tourism. Everybody wants a piece of the high-flying action.
In Spain, startup Zero 2 Infinity (Z2I) bills itself as a “zero-emissions space transportation company” with its Bloon space balloon gondola concept. Not only does Z2I want to take people to the edge of space, it also wants to take paid advertisements up there. Introducing what it calls HAPS for “High-Altitude Platform,s” Z2I intends to put advertising high in the sky, perhaps ushering in a new era in Superbowl ads (and neck strain from looking up).
What about Mr. Tesla himself, Elon Musk? On Feb. 1, the SpaceX founder announced that the first all-civilian crew will be lifted aboard a SpaceX Dragon spacecraft. And who’s leading that civilian crew? It’s Jared Isaacman, founder and CEO of Shift4 Payments , a Pennsylvania-based payment processor. Talk about moving money around the planet — at 17,500 miles per hour.
Well played, sir.
Space Is Officially Open for Business
As for the further commercialization of near-Earth, it’ll be profits before fun. But still fun.
Last October, NASA announced that it will completely commercialize near-Earth communications for orbital and moon missions by 2030. NASA’s reasons for turning to the private sector sound an awful lot like the reasons that banks partner with FinTechs .
“By shifting to commercial communications services, NASA will free up personnel and resources to focus on technology development while bolstering the commercial space economy. This shift may also reduce the overall cost of communications services while enhancing network responsiveness and availability,” per the announcement . Might as well be talking about RTP.
“When NASA becomes just one of many customers in a commercial marketplace, missions can benefit from competitive pricing from an abundance of service providers,” it said.
No discussion about the commercialization of space would be complete without checking in on two other high-flying high-rollers: Virgin’s Sir Richard Branson and Amazon’s Mr. Jeff Bezos.
For his part, Branson’s Virgin Galactic is waitlisting private citizens for a future visit to the International Space Station. The company says that “under an agreement with NASA’s Johnson Space Center, Virgin Galactic is developing a new orbital astronaut readiness program for private individuals interested in purchasing missions to the International Space Station. This program builds on Virgin Galactic’s commercial spaceflight training experience and position as the world’s preeminent space experience brand. It will offer a unique turnkey approach to orbital flight, from the procurement of transportation to the provision of training, all delivered with an unparalleled and proven commitment to personalized customer experience.”
If you’re wondering what Bezos is going to do with his spare time as he’s no longer day-to-day at Amazon, we’re betting he’ll spend more time with Blue Origin , his own spaceflight venture.
People are waitlisting for rides in Blue Origin’s suborbital, reusable New Shepard rocket system, of which the website boasts “every seat’s a window seat.” A trip will include a stay at the secluded high-plains desert launch area in West Texas. It’s an 11-minute flight, or will be, but we’re guessing it would be the 11 most memorable minutes of one’s earthy life.
Recommended
Trending news, the big story.
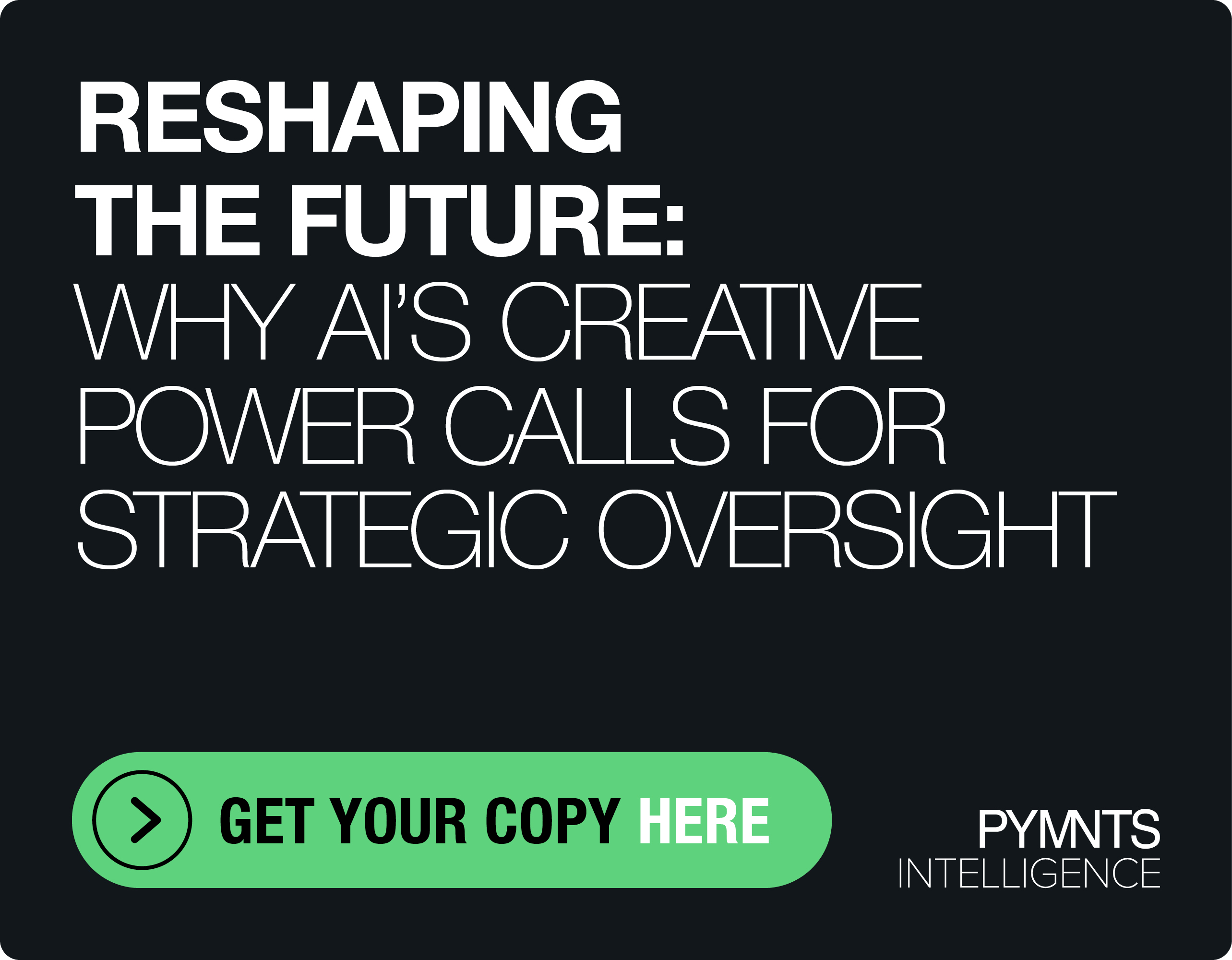
Featured News
Partner with pymnts.
We’re always on the lookout for opportunities to partner with innovators and disruptors.
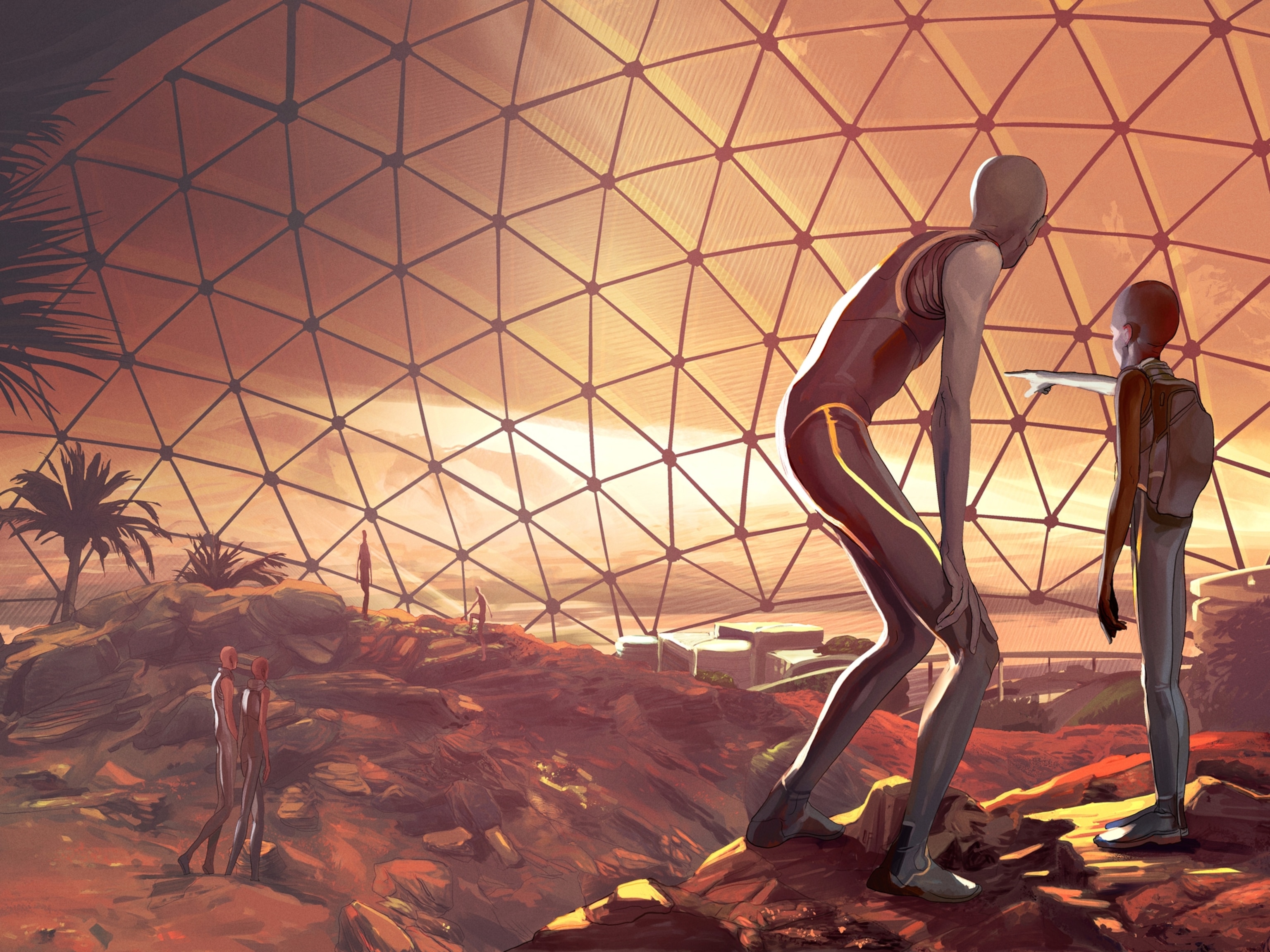
The future of spaceflight—from orbital vacations to humans on Mars
NASA aims to travel to the moon again—and beyond. Here’s a look at the 21st-century race to send humans into space.
Welcome to the 21st-century space race, one that could potentially lead to 10-minute space vacations, orbiting space hotels , and humans on Mars. Now, instead of warring superpowers battling for dominance in orbit, private companies are competing to make space travel easier and more affordable. This year, SpaceX achieved a major milestone— launching humans to the International Space Station (ISS) from the United States —but additional goalposts are on the star-studded horizon.
Private spaceflight
Private spaceflight is not a new concept . In the United States, commercial companies played a role in the aerospace industry right from the start: Since the 1960s, NASA has relied on private contractors to build spacecraft for every major human spaceflight program, starting with Project Mercury and continuing until the present.
Today, NASA’s Commercial Crew Program is expanding on the agency’s relationship with private companies. Through it, NASA is relying on SpaceX and Boeing to build spacecraft capable of carrying humans into orbit. Once those vehicles are built, both companies retain ownership and control of the craft, and NASA can send astronauts into space for a fraction of the cost of a seat on Russia’s Soyuz spacecraft.
SpaceX, which established a new paradigm by developing reusable rockets , has been running regular cargo resupply missions to the International Space Station since 2012. And in May 2020, the company’s Crew Dragon spacecraft carried NASA astronauts Doug Hurley and Bob Behnken to the ISS , becoming the first crewed mission to launch from the United States in nearly a decade. The mission, called Demo-2, is scheduled to return to Earth in August. Boeing is currently developing its Starliner spacecraft and hopes to begin carrying astronauts to the ISS in 2021.
Other companies, such as Blue Origin and Virgin Galactic , are specializing in sub-orbital space tourism. Test launch video from inside the cabin of Blue Origin’s New Shepard shows off breathtaking views of our planet and a relatively calm journey for its first passenger, a test dummy cleverly dubbed “Mannequin Skywalker.” Virgin Galactic is running test flights on its sub-orbital spaceplane , which will offer paying customers roughly six minutes of weightlessness during its journey through Earth’s atmosphere.
With these and other spacecraft in the pipeline, countless dreams of zero-gravity somersaults could soon become a reality—at least for passengers able to pay the hefty sums for the experience.
Early U.S. Spaceflight
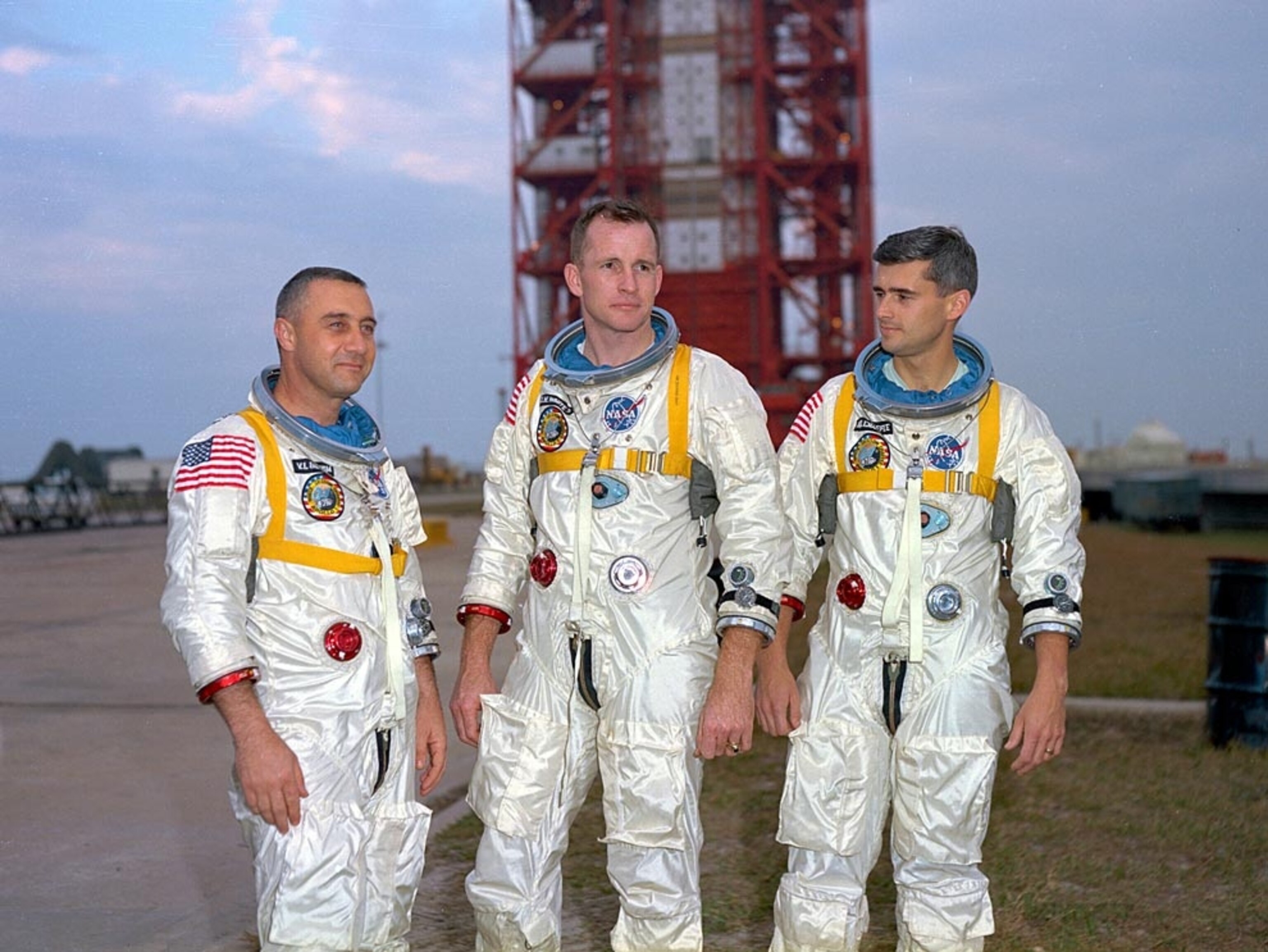
Looking to the moon
Moon missions are essential to the exploration of more distant worlds. After a long hiatus from the lunar neighborhood, NASA is again setting its sights on Earth’s nearest celestial neighbor with an ambitious plan to place a space station in lunar orbit sometime in the next decade. Sooner, though, the agency’s Artemis program , a sister to the Apollo missions of the 1960s and 1970s, is aiming to put the first woman (and the next man) on the lunar surface by 2024.
FREE BONUS ISSUE
Extended lunar stays build the experience and expertise needed for the long-term space missions required to visit other planets. As well, the moon may also be used as a forward base of operations from which humans learn how to replenish essential supplies, such as rocket fuel and oxygen, by creating them from local material.
You May Also Like
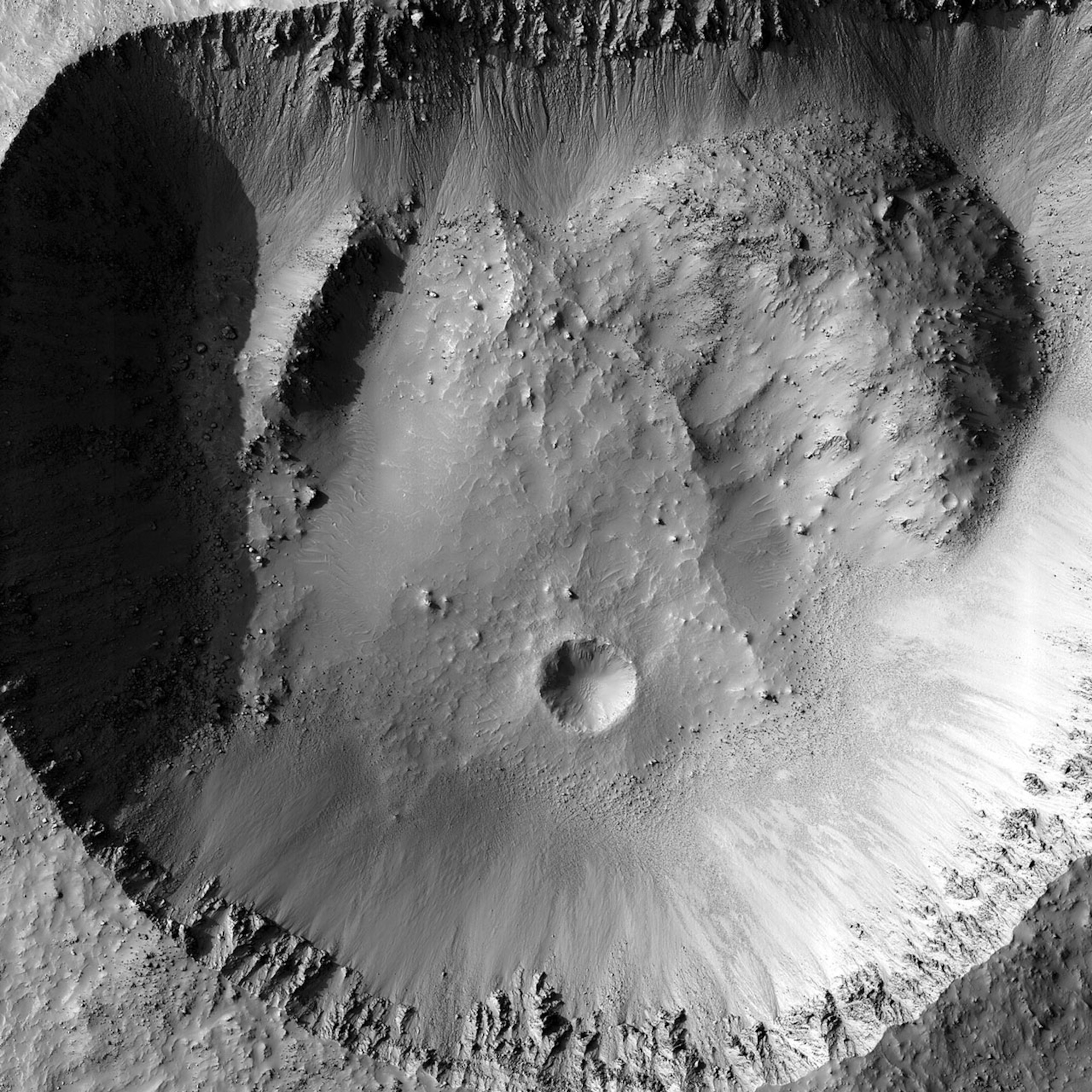
In a first, NASA Mars lander feels shockwaves from meteor impacts
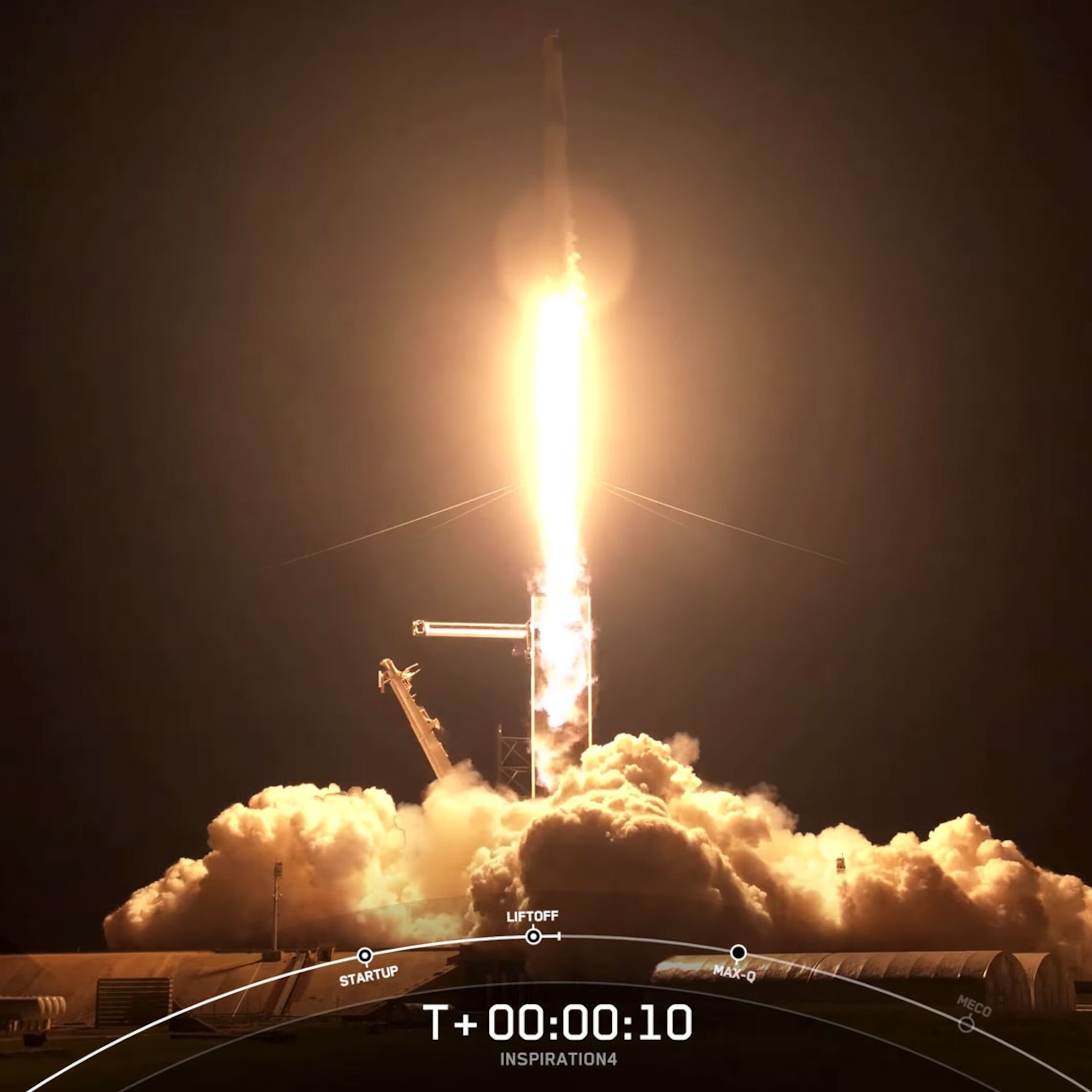
SpaceX takes 4 passengers to orbit—a glimpse at private spaceflight’s future
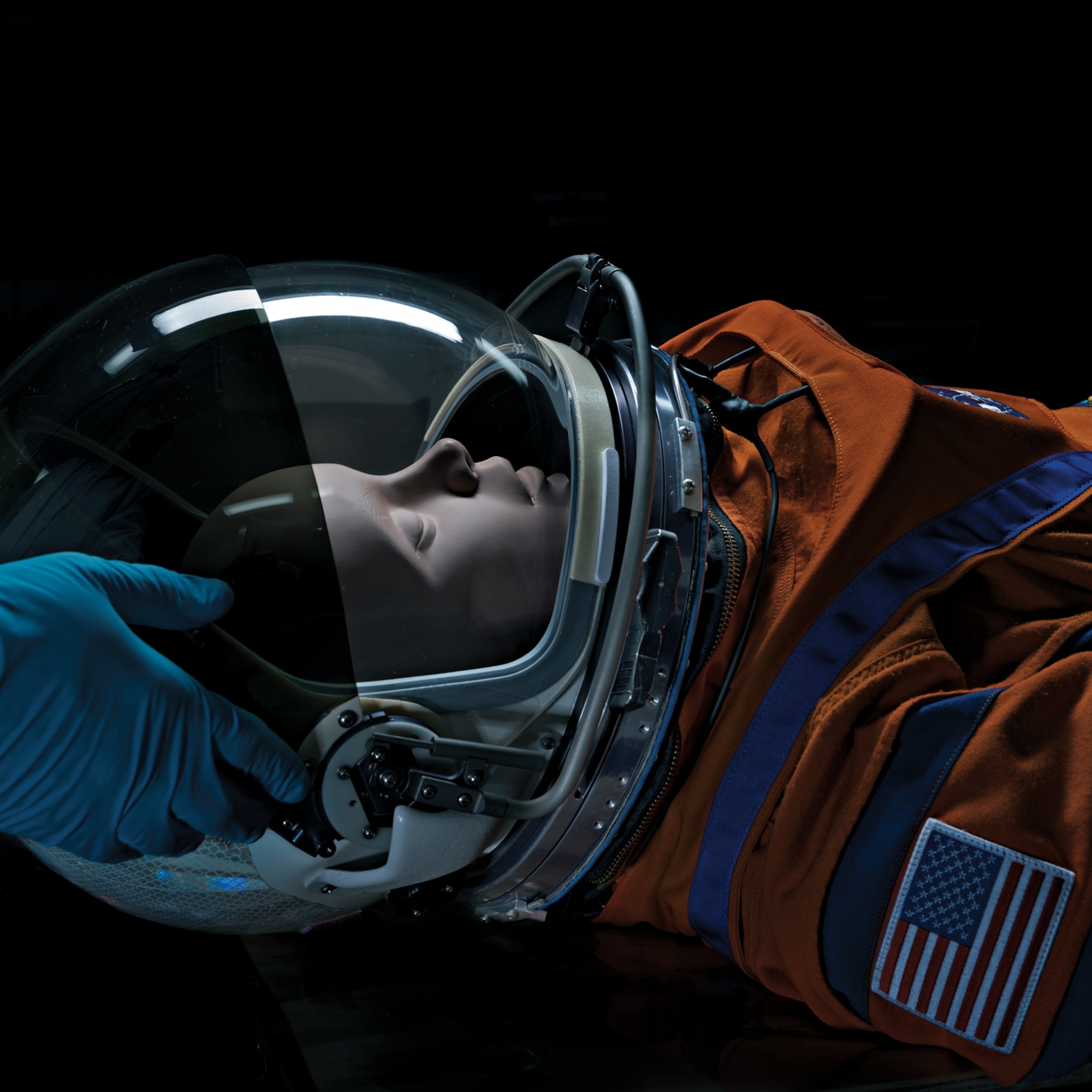
Why go back to the moon? NASA’s Artemis program has even bigger ambitions
Such skills are crucial for the future expansion of human presence into deeper space, which demands more independence from Earth-based resources. And although humans have visited the moon before, the cratered sphere still harbors its own scientific mysteries to be explored—including the presence and extent of water ice near the moon's south pole, which is one of the top target destinations for space exploration .
NASA is also enlisting the private sector to help it reach the moon. It has awarded three contracts to private companies working on developing human-rated lunar landers—including both Blue Origin and SpaceX. But the backbone of the Artemis program relies on a brand new, state-of-the-art spacecraft called Orion .
Archival Photos of Spaceflight
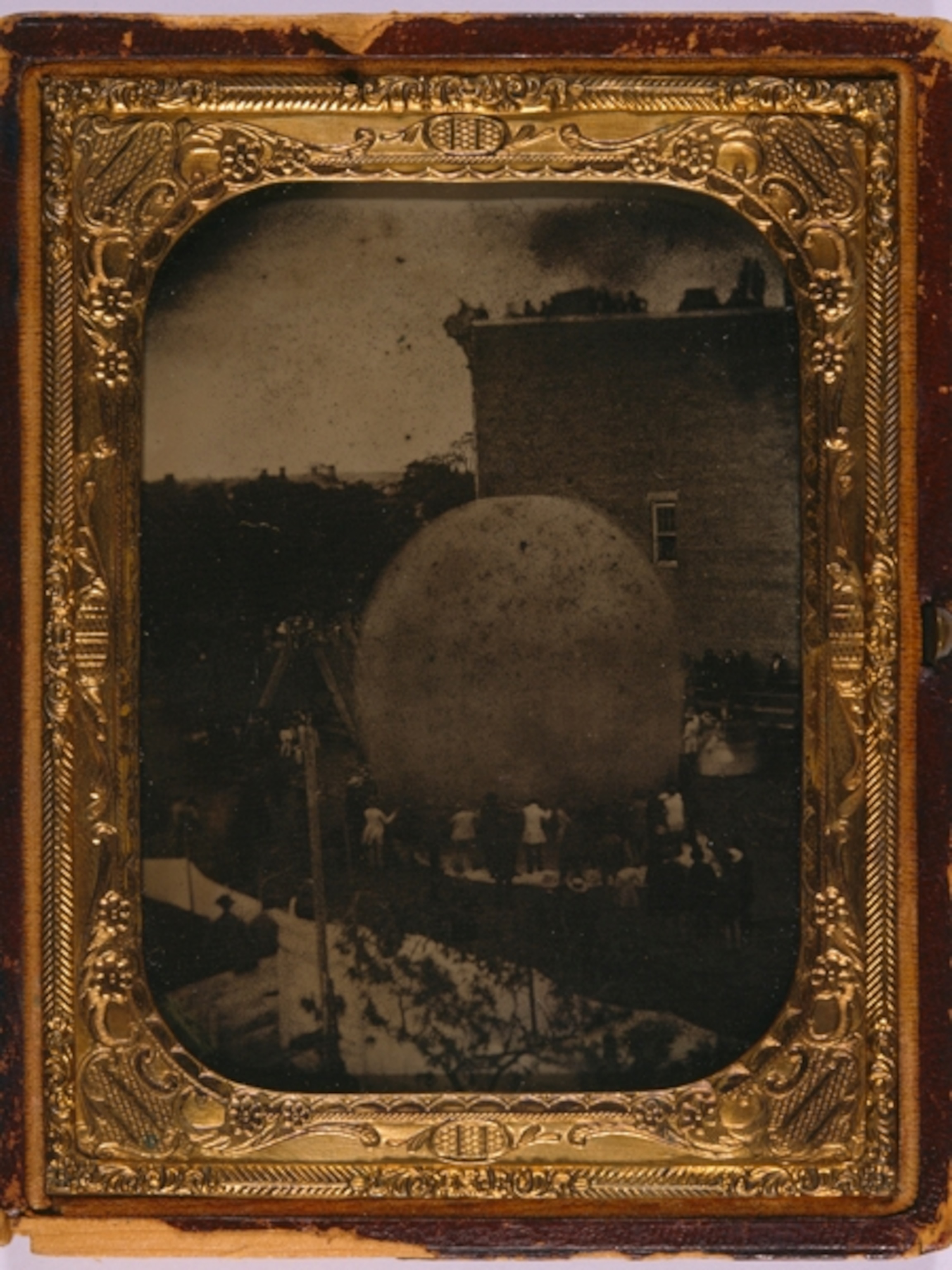
Currently being built and tested, Orion—like Crew Dragon and Starliner—is a space capsule similar to the spacecraft of the Mercury, Gemini, and Apollo programs, as well as Russia’s Soyuz spacecraft. But the Orion capsule is larger and can accommodate a four-person crew. And even though it has a somewhat retro design, the capsule concept is considered to be safer and more reliable than NASA’s space shuttle—a revolutionary vehicle for its time, but one that couldn’t fly beyond Earth’s orbit and suffered catastrophic failures.
Capsules, on the other hand, offer launch-abort capabilities that can protect astronauts in case of a rocket malfunction. And, their weight and design mean they can also travel beyond Earth’s immediate neighborhood, potentially ferrying humans to the moon, Mars, and beyond.
A new era in spaceflight
By moving into orbit with its Commercial Crew Program and partnering with private companies to reach the lunar surface, NASA hopes to change the economics of spaceflight by increasing competition and driving down costs. If space travel truly does become cheaper and more accessible, it’s possible that private citizens will routinely visit space and gaze upon our blue, watery home world—either from space capsules, space stations, or even space hotels like the inflatable habitats Bigelow Aerospace intends to build .
The United States isn’t the only country with its eyes on the sky. Russia regularly launches humans to the International Space Station aboard its Soyuz spacecraft. China is planning a large, multi-module space station capable of housing three taikonauts, and has already launched two orbiting test vehicles—Tiangong-1 and Tiangong-2, both of which safely burned up in the Earth’s atmosphere after several years in space.
Now, more than a dozen countries have the ability to launch rockets into Earth orbit. A half-dozen space agencies have designed spacecraft that shed the shackles of Earth’s gravity and traveled to the moon or Mars. And if all goes well, the United Arab Emirates will join that list in the summer of 2020 when its Hope spacecraft heads to the red planet . While there are no plans yet to send humans to Mars, these missions—and the discoveries that will come out of them—may help pave the way.
Related Topics
- SPACE EXPLORATION
- SCIENCE AND TECHNOLOGY
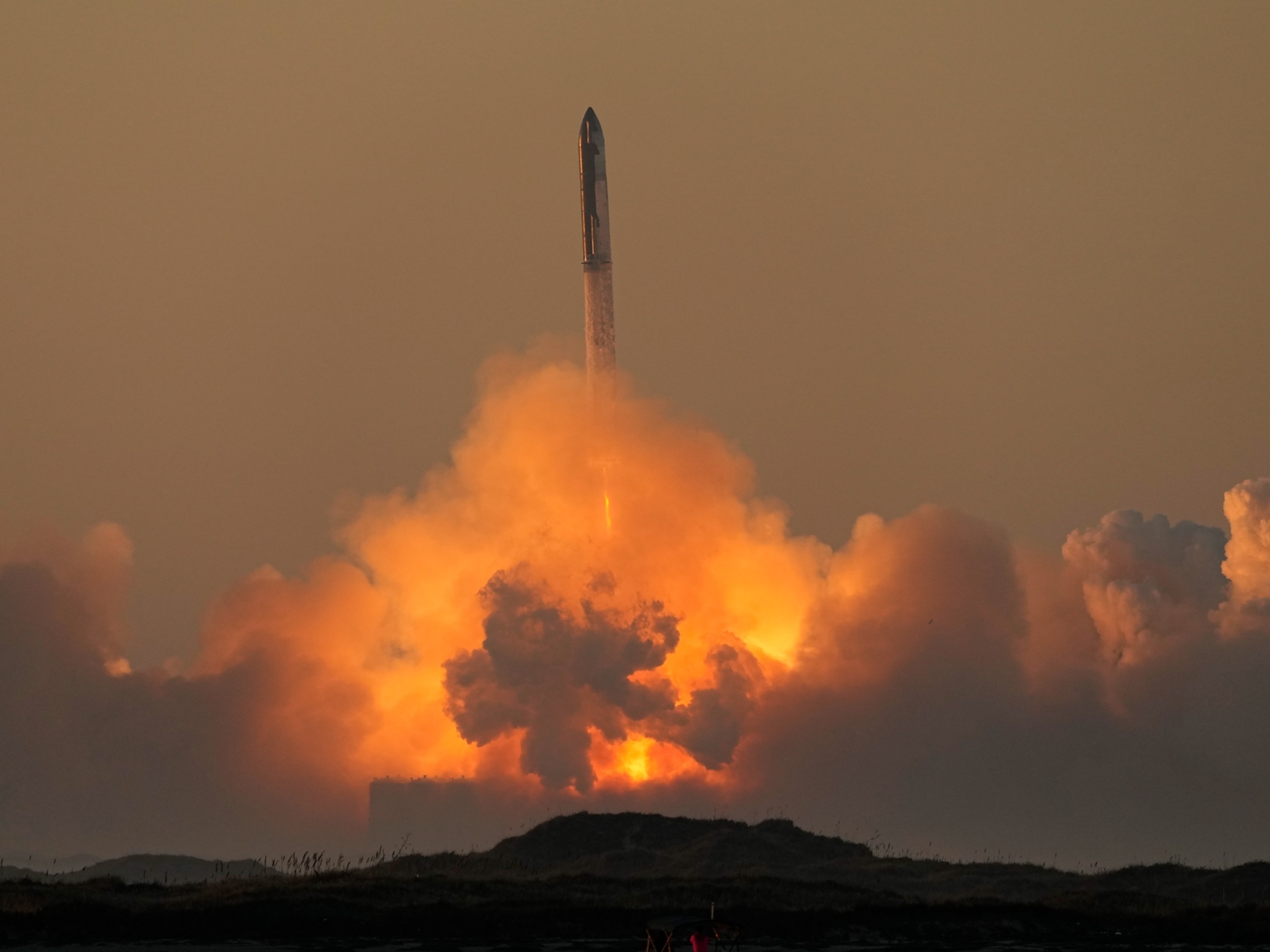
Second SpaceX megarocket launch ends with another explosion. What happens next?
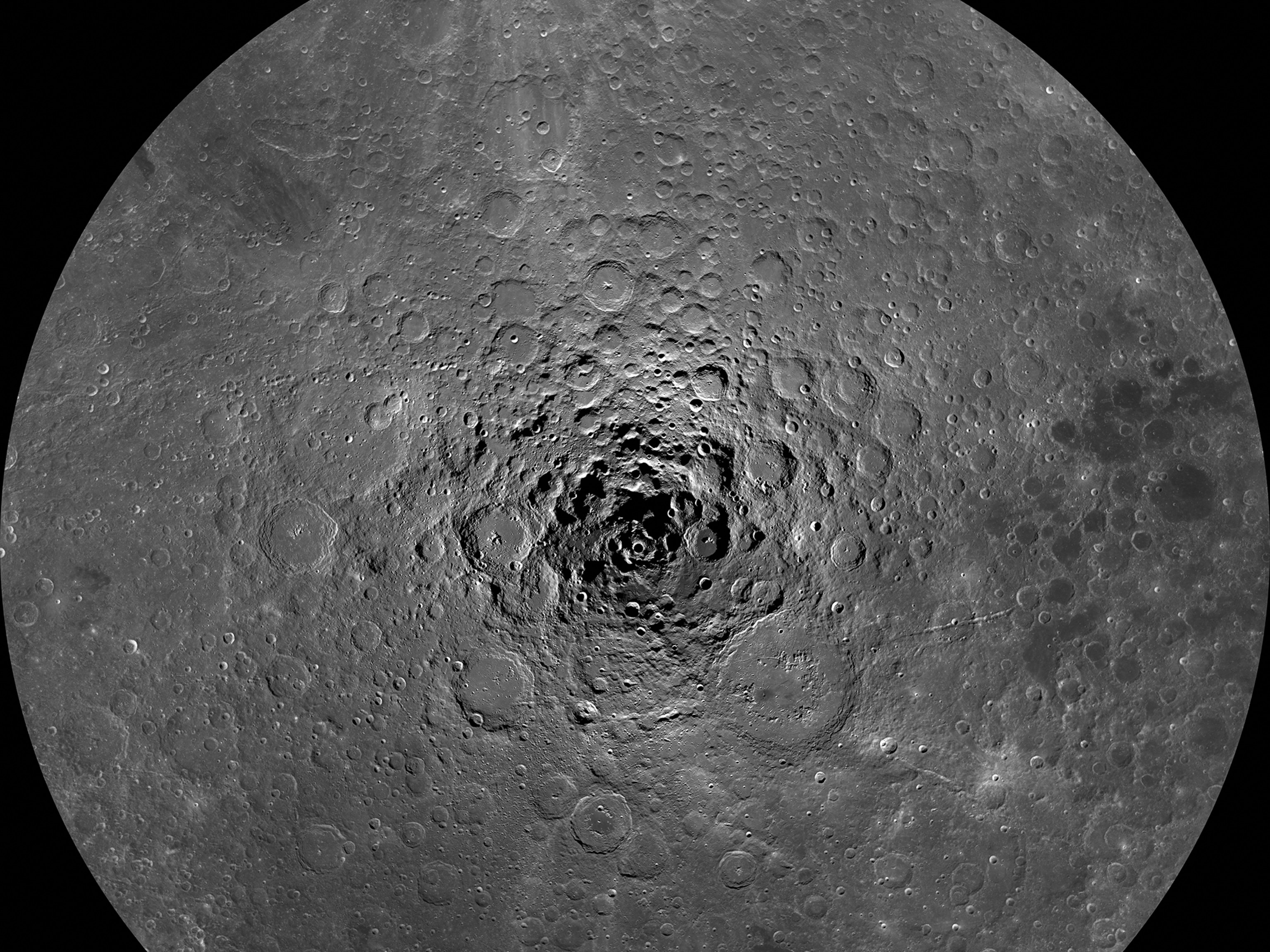
Why did India land near the moon’s south pole?
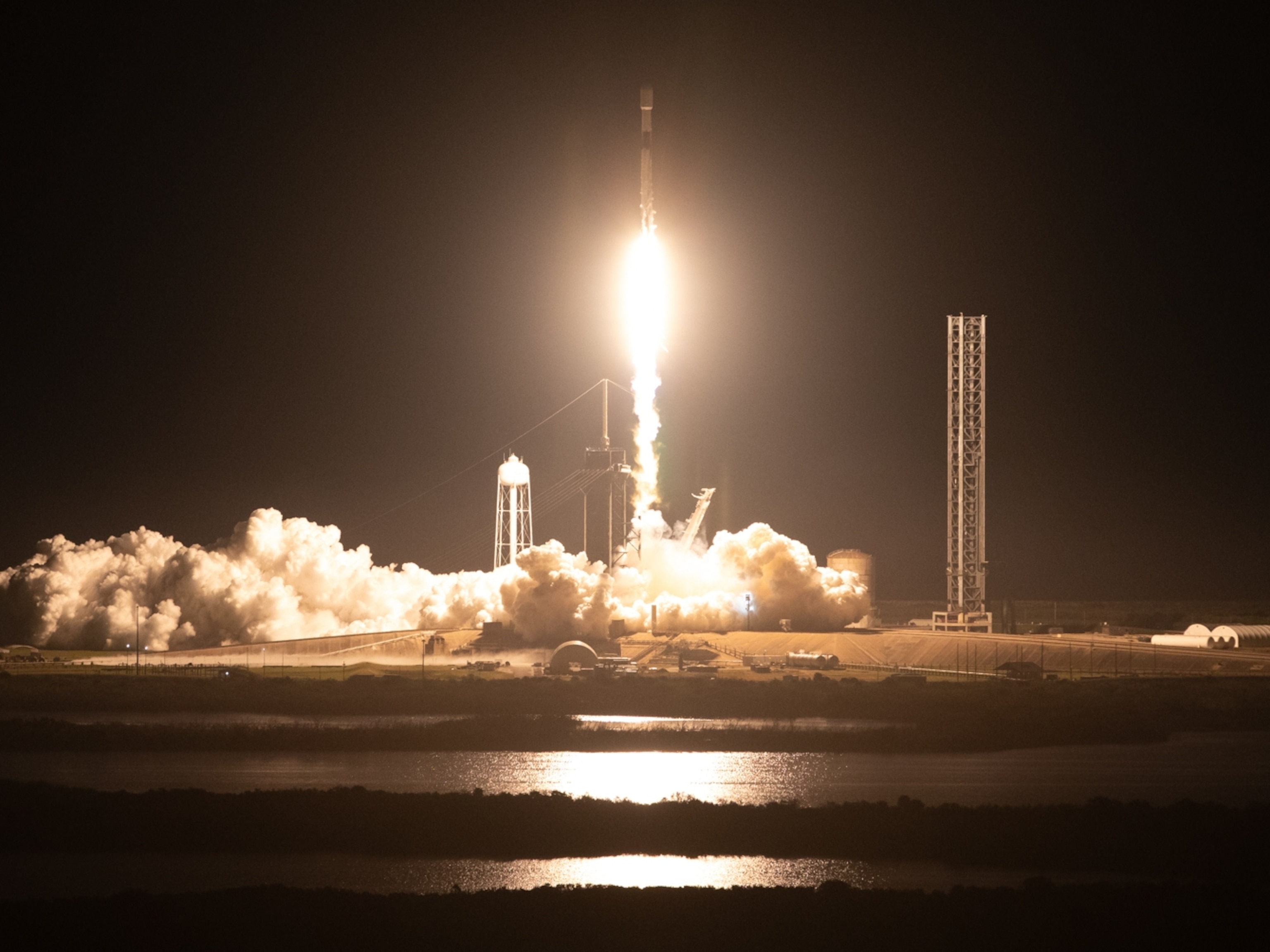
U.S. returns to the moon as NASA's Odysseus successfully touches down
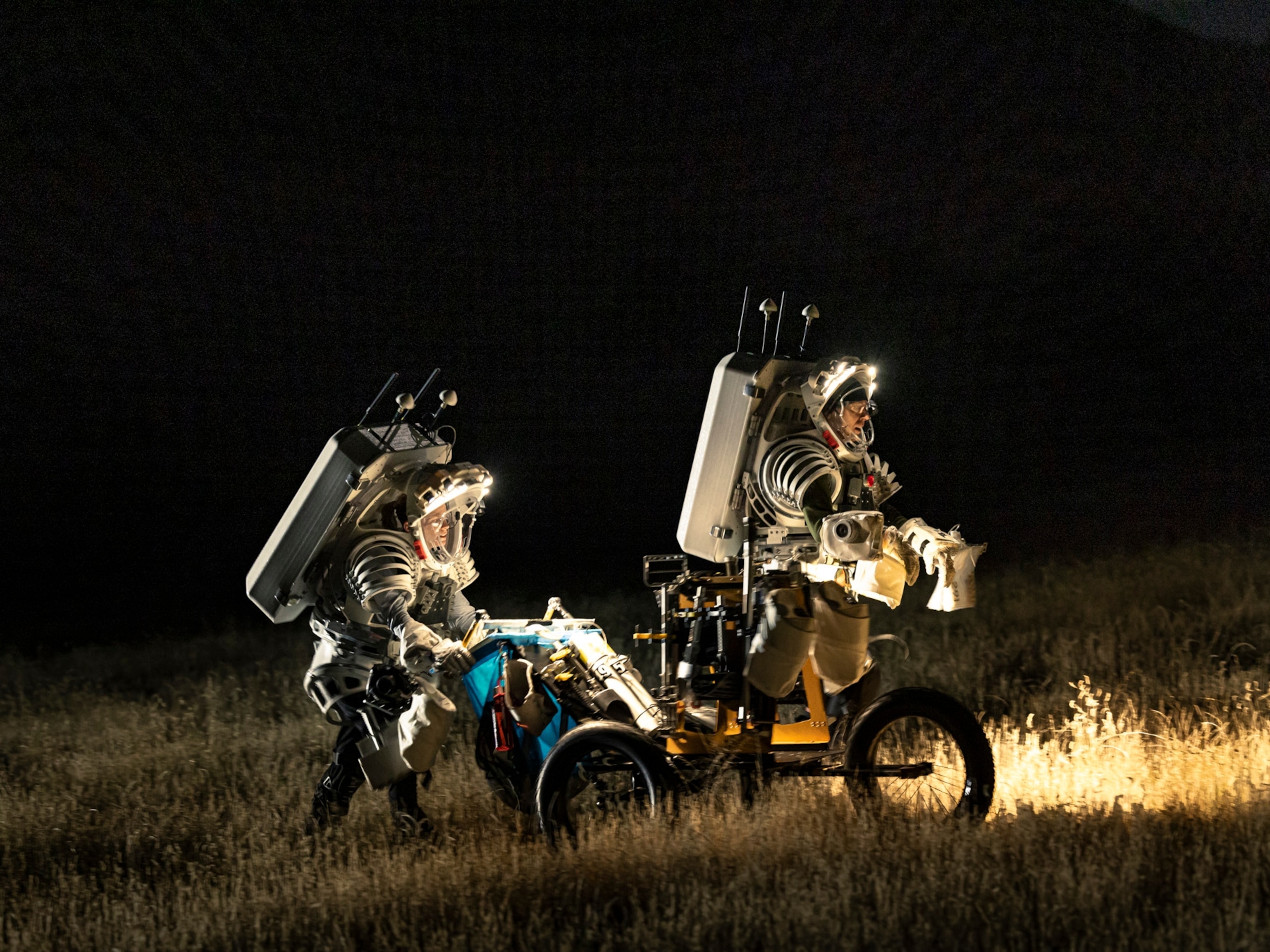
In the Arizona desert, NASA prepares for walking on the moon
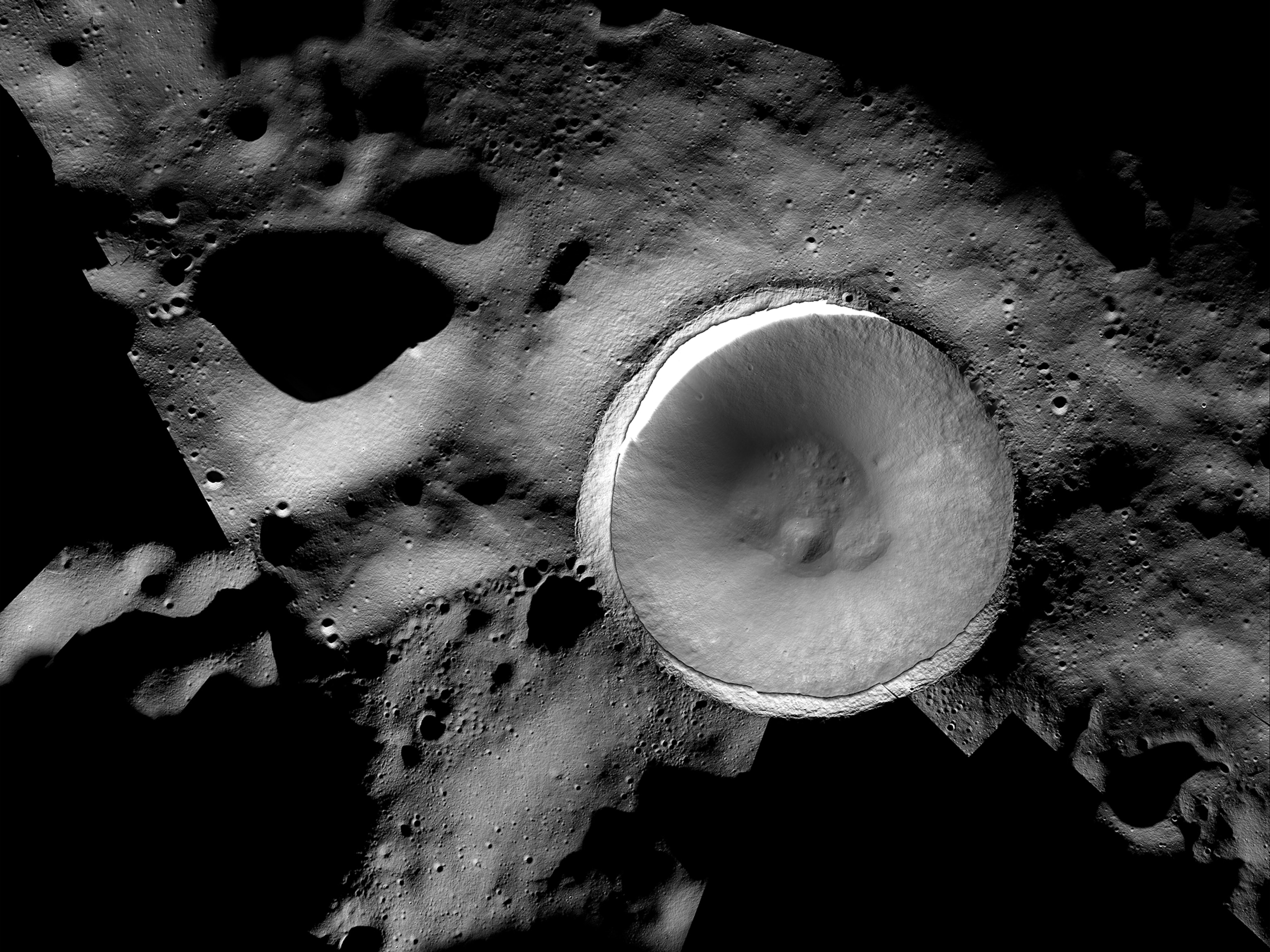
The moon’s darkest corners are a mystery. This image offers a stunning new glimpse.
- Environment
- Perpetual Planet
History & Culture
- History & Culture
- History Magazine
- Mind, Body, Wonder
- Paid Content
- Terms of Use
- Privacy Policy
- Your US State Privacy Rights
- Children's Online Privacy Policy
- Interest-Based Ads
- About Nielsen Measurement
- Do Not Sell or Share My Personal Information
- Nat Geo Home
- Attend a Live Event
- Book a Trip
- Inspire Your Kids
- Shop Nat Geo
- Visit the D.C. Museum
- Learn About Our Impact
- Support Our Mission
- Advertise With Us
- Customer Service
- Renew Subscription
- Manage Your Subscription
- Work at Nat Geo
- Sign Up for Our Newsletters
- Contribute to Protect the Planet
Copyright © 1996-2015 National Geographic Society Copyright © 2015-2024 National Geographic Partners, LLC. All rights reserved
What it would be like to travel to Mars as a space tourist
Following is a transcript of the video.
Imagine you could vacation on Mars. At first glance, Mars appears very different from Earth, but if you take a closer look, you'll discover that the Red Planet isn't all that different from our own.
Our first stop, the North Pole. This ice cap is bigger than Texas, and is mostly covered in water ice and solid carbon dioxide, aka dry ice. Heading south, we'll visit Kasei Valles. It's a vast system of chasms five times longer and 10 times wider than the Grand Canyon, and scientists think it formed in a similar way. Billions of years ago, Mars was warmer, and covered with liquid water, which likely carved out valleys like this one.
Mawrth Vallis
Mawrth Vallis is another valley close by, but it looks very different from our previous spot, thanks to its multicolored layers of clay. These deposits probably formed over millennia as Mars shifted towards a colder, drier climate, and they could provide clues to the history of liquid water and possible ancient life on Mars.
Bacquerel Crater
The next stop is another place that may have been teeming with life long ago, Bacquerel Crater. It's filled with rocks made of sulfate similar to ones on Earth that form after water evaporates, which has led scientists to suspect that this crater may once have been a massive lake over 160 kilometers wide.
Next stop, Iani Chaos. Now, this is one type of terrain you won't find anywhere on Earth. It's a maze of rugged cliffs and pillar-like hills called mesas that extend for 200 kilometers, and since there is nothing like it on Earth, scientists aren't exactly sure how these unique features formed. Its larger neighbor, Hydraotes Chaos, probably formed in a similar mysterious way. It stretches 350 kilometers, the same distance as New York City to Boston.
Valles Marineris
West of Hydraotes, we find one Mars's greatest attractions, Valles Marineris. It's the largest canyon in the solar system, running the length of New York to Los Angeles, and plunging four times as deep as the Grand Canyon.
Hebes Chasma
Following the canyon's main channel north, we reach Hebes Chasma. It's tiny compared to Valles Marineris, but it's worth the trek for a glimpse of the chasma's prominent mesa.
Olympus Mons
And no trip to Mars would be complete without a visit to Olympus Mons, the tallest volcano in the solar system. It covers a region the size of Arizona. It's three times as tall as Mount Everest, and can comfortably fit all the volcanoes in Hawaii.
Promethei Planum
Moving south, we'll see Promethei Planum. It's a plane near the South Pole, covered in a sheet of ice nearly one and a half times as thick as the East Antarctic Ice Sheet.
Rabe Crater
Next, we'll swing around to Rabe Crater. It's covered in giant sand dunes 150 to 200 meters high, almost as tall as the Golden Gate Bridge.
Neukum Crater
Nearing the end of our tour is one of the oldest regions of Mars that dates back to at least 3.9 billion years ago, Neukum Crater. Scientists think this crater formed from a powerful impact early in Mars's history. In fact, you can still see pockmarks left by the crash.
Galle Crater
The last crater on our tour is formally named Galle Crater, but many call it Happy Face Crater for obvious reasons.
Finally, we've reached our last stop, the South Pole. In 2018, scientists found evidence of a liquid lake beneath the ice, which could be filled with saltwater.
But while that's a promising discovery, we still have much to learn about this fascinating world, like whether life once existed in those ancient lakes, or if it still exists today, possibly somewhere underground. But perhaps the biggest question of all, could human life survive here? Maybe in the future, you won't have to imagine your Martian vacation.
Video credit: ESA / Freie Universitat Berlin Planetary Sciences and Remote Sensing / CC BY-SA 3.0 IGO
EDITOR'S NOTE: This video was originally published in February 2019.
More from Science
- Main content
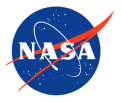
Suggested Searches
- Climate Change
- Expedition 64
- Mars perseverance
- SpaceX Crew-2
- International Space Station
- View All Topics A-Z
Humans in Space
Earth & climate, the solar system, the universe, aeronautics, learning resources, news & events.
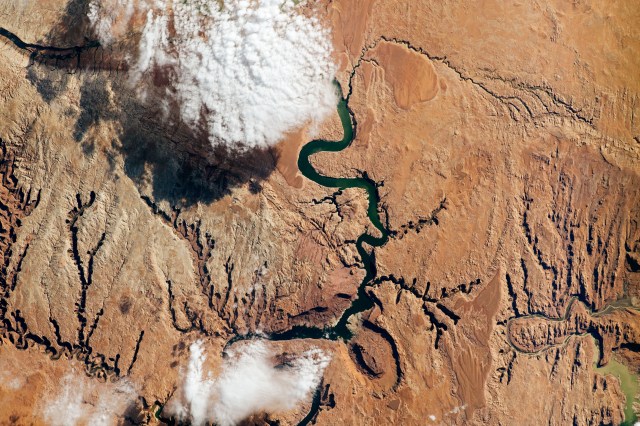
NASA-Led Study Provides New Global Accounting of Earth’s Rivers
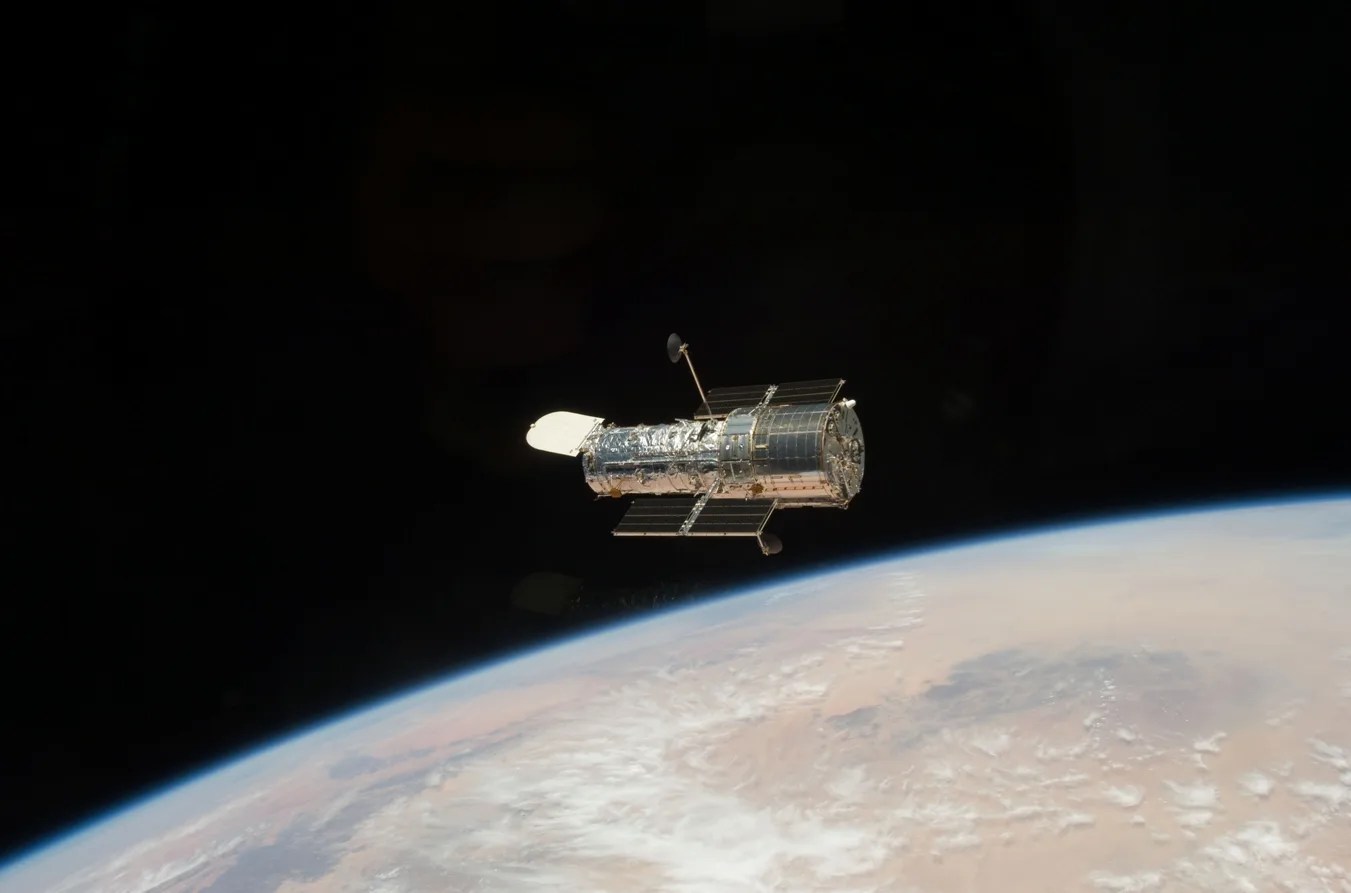
NASA’s Hubble Pauses Science Due to Gyro Issue
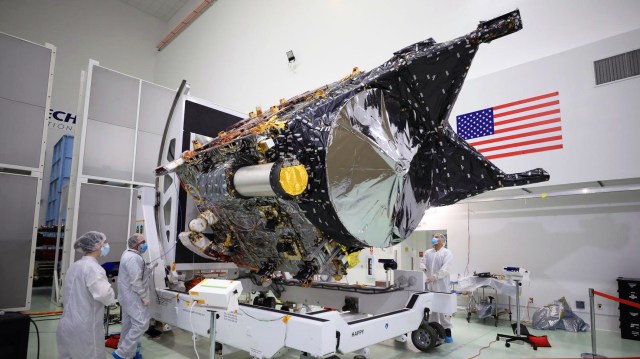
NASA’s Optical Comms Demo Transmits Data Over 140 Million Miles
- Search All NASA Missions
- A to Z List of Missions
- Upcoming Launches and Landings
- Spaceships and Rockets
- Communicating with Missions
- James Webb Space Telescope
- Hubble Space Telescope
- Why Go to Space
- Astronauts Home
- Commercial Space
- Destinations
- Living in Space
- Explore Earth Science
- Earth, Our Planet
- Earth Science in Action
- Earth Multimedia
- Earth Science Researchers
- Pluto & Dwarf Planets
- Asteroids, Comets & Meteors
- The Kuiper Belt
- The Oort Cloud
- Skywatching
- The Search for Life in the Universe
- Black Holes
- The Big Bang
- Dark Energy & Dark Matter
- Earth Science
- Planetary Science
- Astrophysics & Space Science
- The Sun & Heliophysics
- Biological & Physical Sciences
- Lunar Science
- Citizen Science
- Astromaterials
- Aeronautics Research
- Human Space Travel Research
- Science in the Air
- NASA Aircraft
- Flight Innovation
- Supersonic Flight
- Air Traffic Solutions
- Green Aviation Tech
- Drones & You
- Technology Transfer & Spinoffs
- Space Travel Technology
- Technology Living in Space
- Manufacturing and Materials
- Science Instruments
- For Kids and Students
- For Educators
- For Colleges and Universities
- For Professionals
- Science for Everyone
- Requests for Exhibits, Artifacts, or Speakers
- STEM Engagement at NASA
- NASA's Impacts
- Centers and Facilities
- Directorates
- Organizations
- People of NASA
- Internships
- Our History
- Doing Business with NASA
- Get Involved
- Aeronáutica
- Ciencias Terrestres
- Sistema Solar
- All NASA News
- Video Series on NASA+
- Newsletters
- Social Media
- Media Resources
- Upcoming Launches & Landings
- Virtual Events
- Sounds and Ringtones
- Interactives
- STEM Multimedia
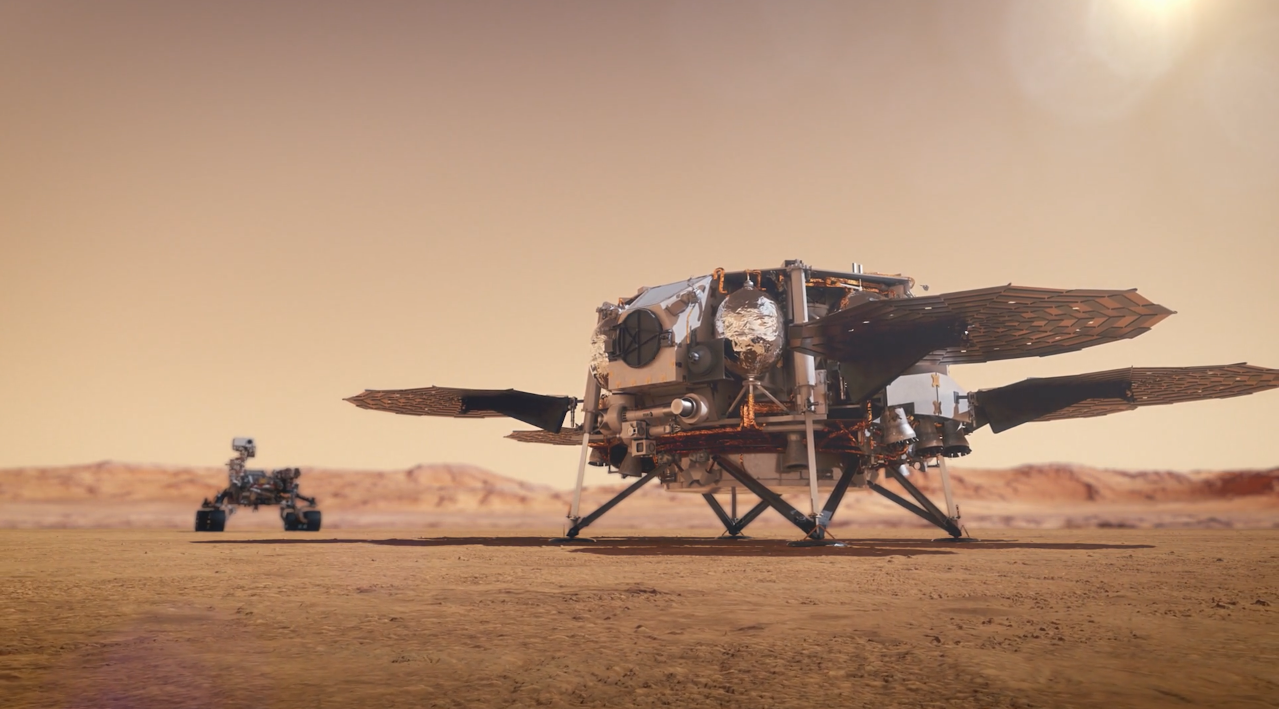
Correction and Clarification of C.26 Rapid Mission Design Studies for Mars Sample Return
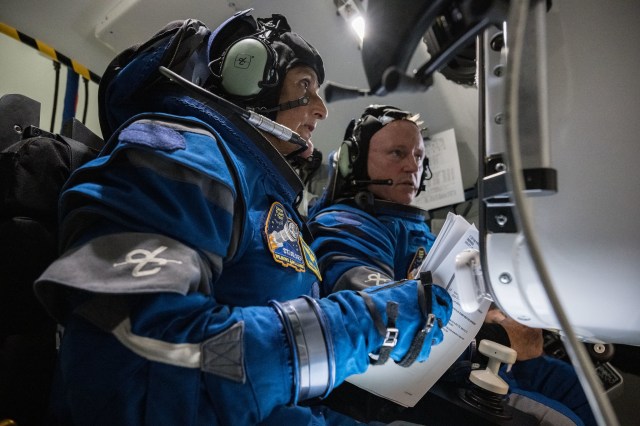
NASA’s Commercial Partners Deliver Cargo, Crew for Station Science
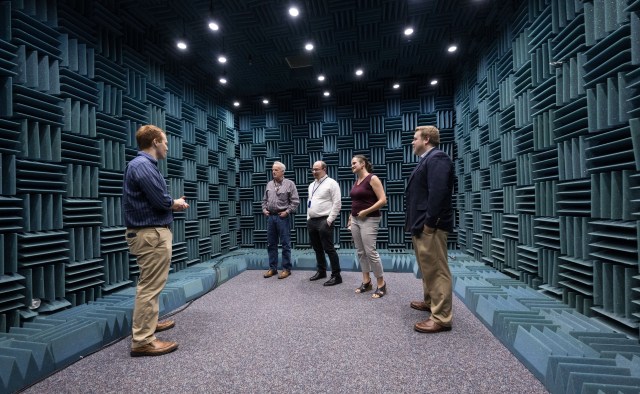
NASA Shares Lessons of Human Systems Integration with Industry

Work Underway on Large Cargo Landers for NASA’s Artemis Moon Missions
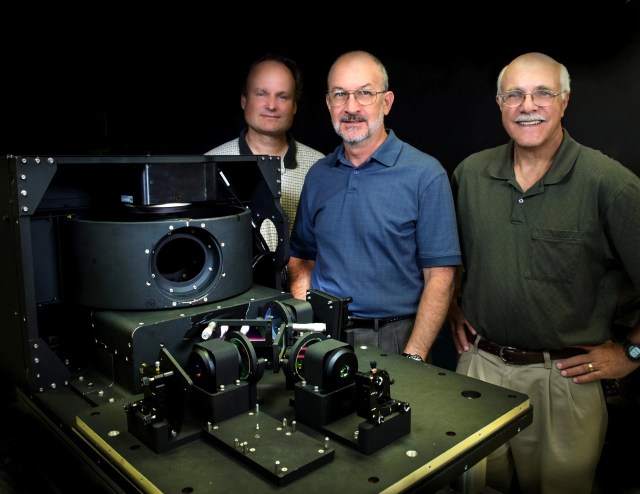
NASA’s ORCA, AirHARP Projects Paved Way for PACE to Reach Space
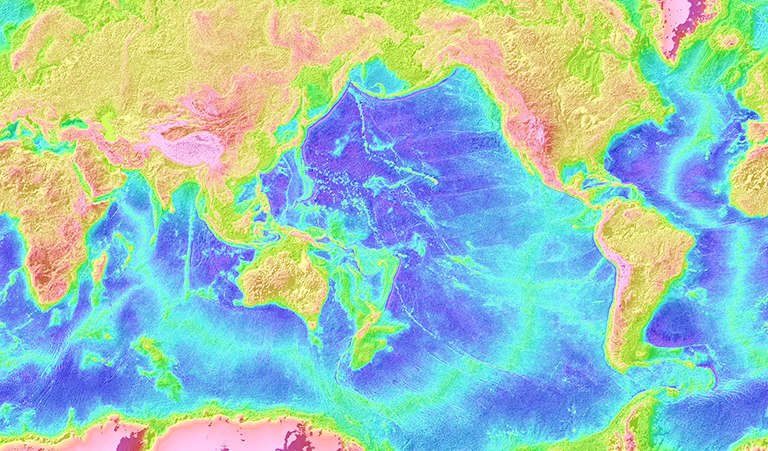
Amendment 11: Physical Oceanography not solicited in ROSES-2024
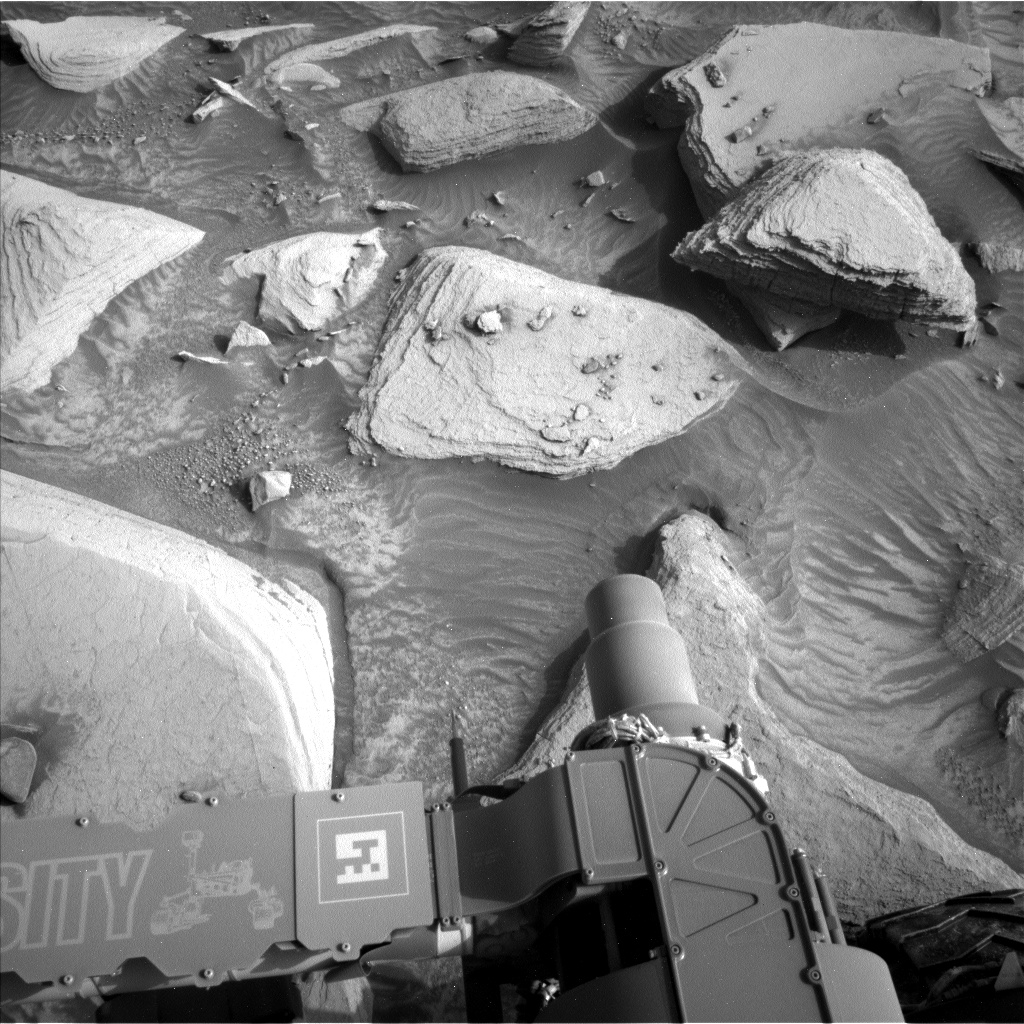
Sols 4166-4167: A Garden Full of Rocks
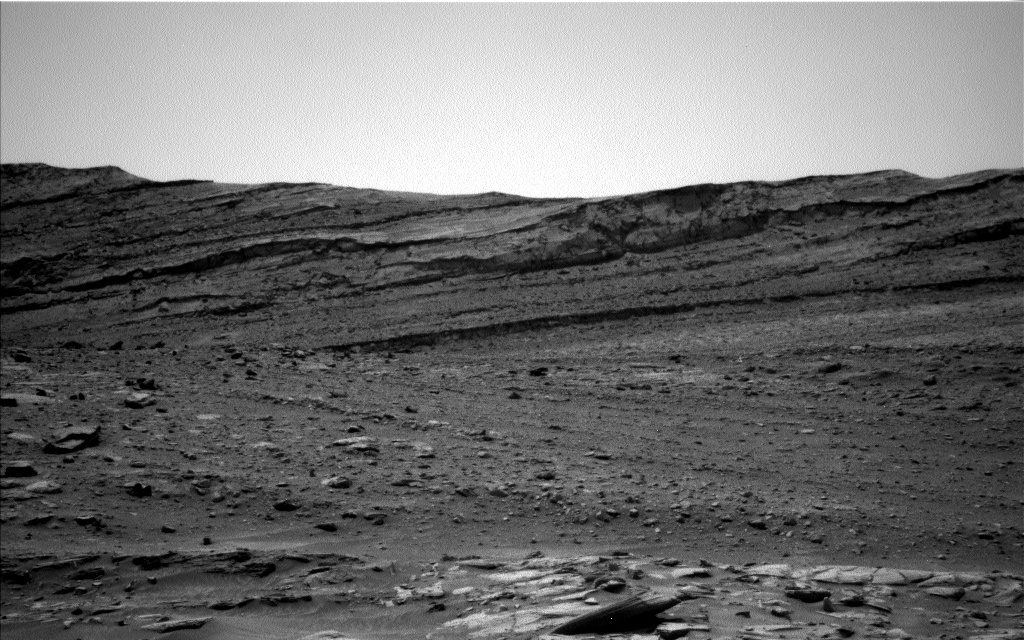
Sols 4164-4165: What’s Around the Ridge-bend?
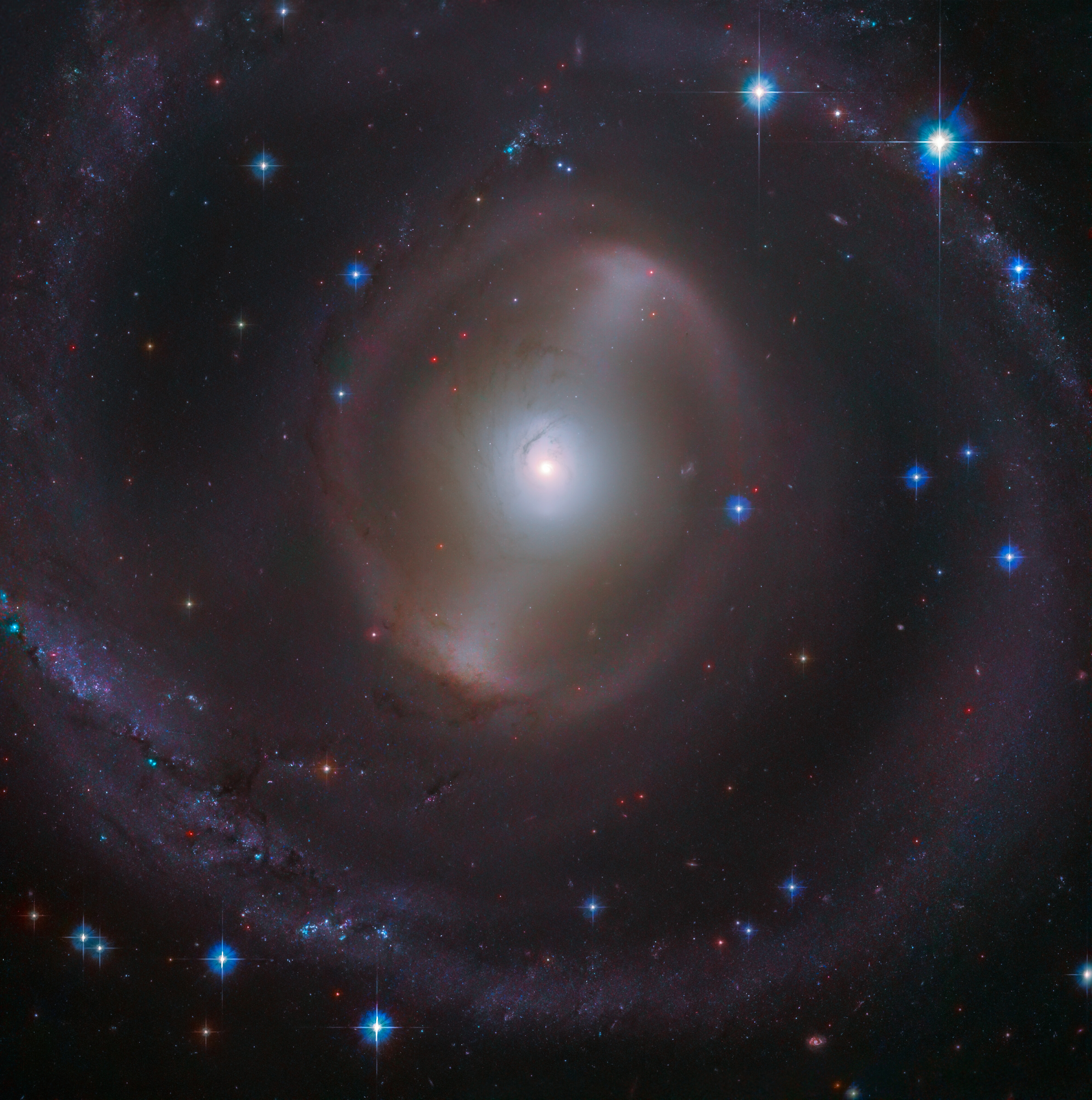
Hubble Spots a Magnificent Barred Galaxy
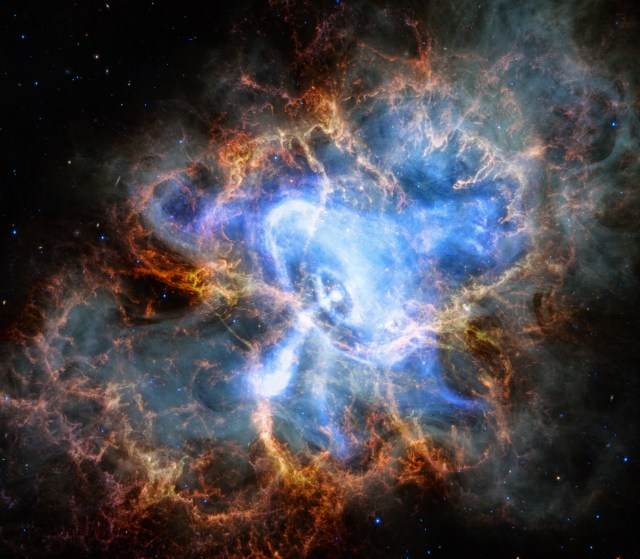
NASA’s Chandra Releases Doubleheader of Blockbuster Hits
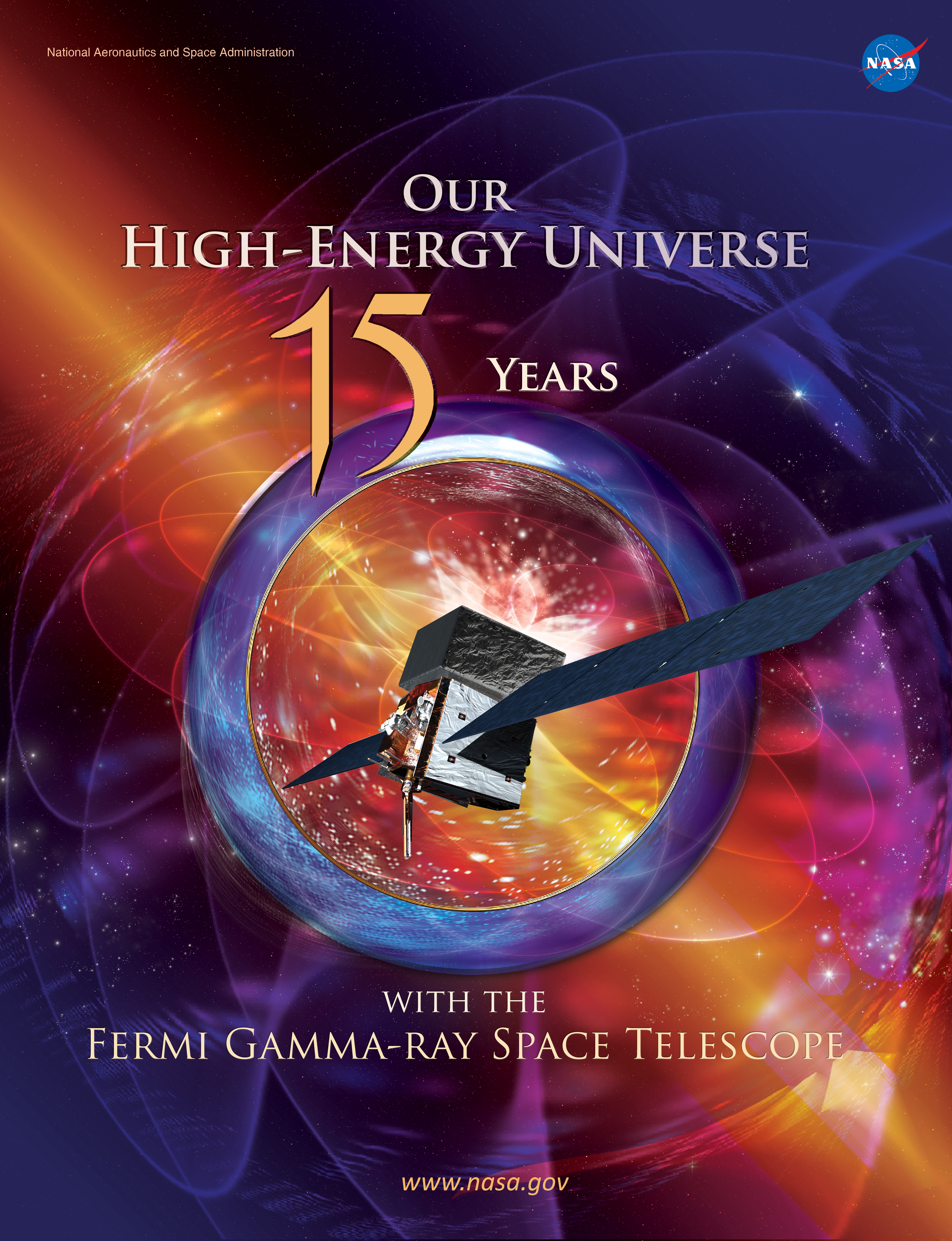
Explore the Universe with the First E-Book from NASA’s Fermi
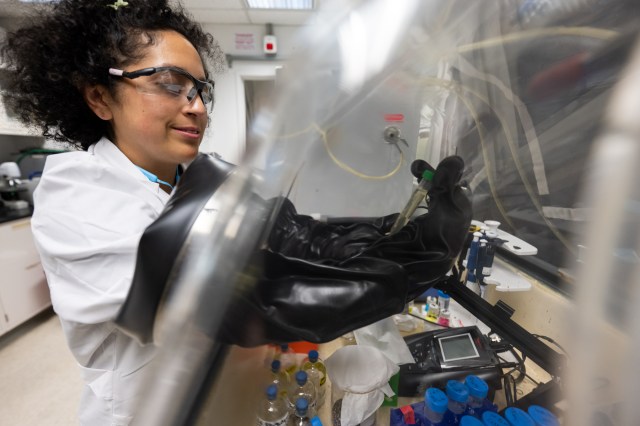
NASA Grant Brings Students at Underserved Institutions to the Stars
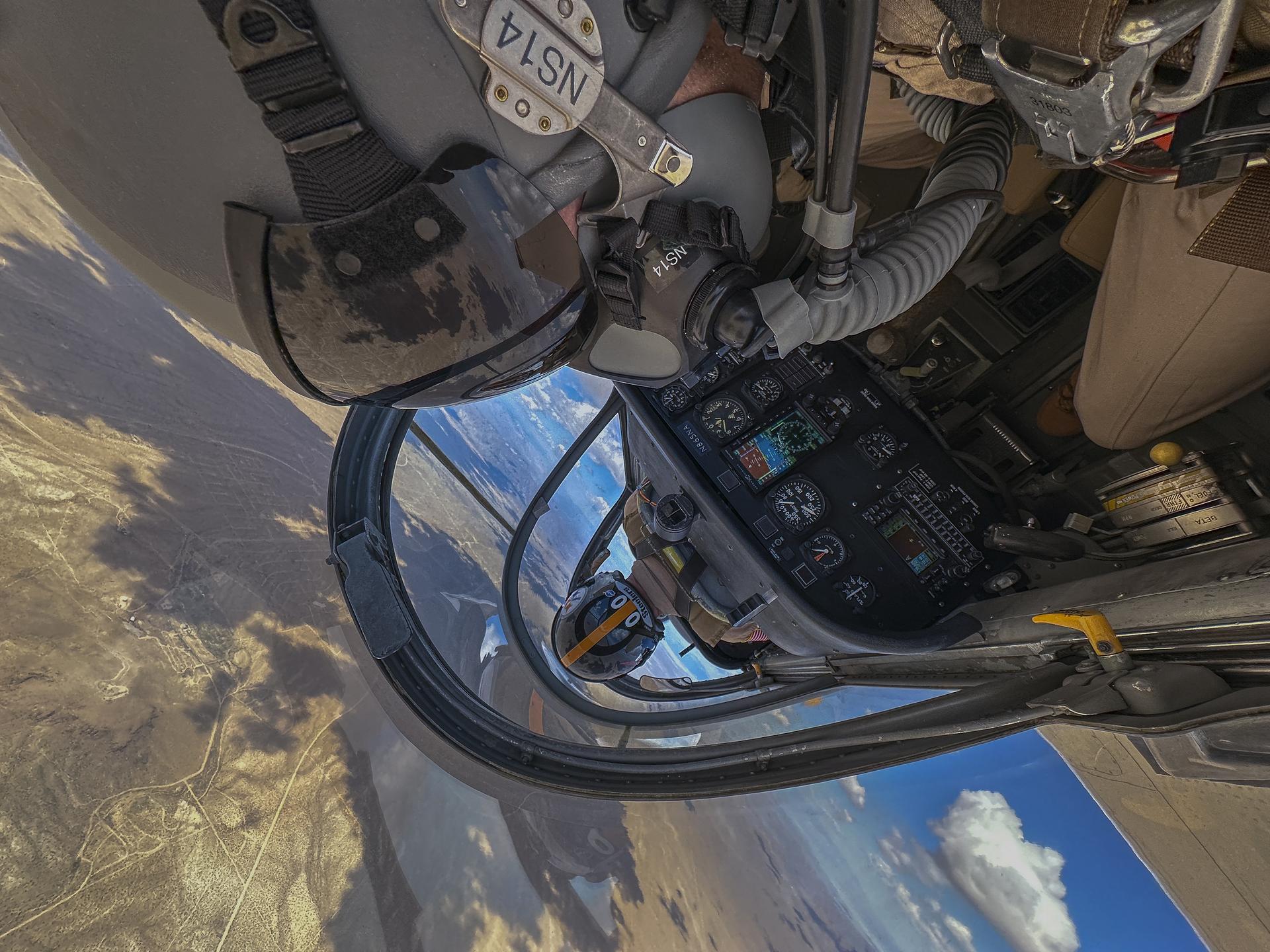
NASA Photographer Honored for Thrilling Inverted In-Flight Image
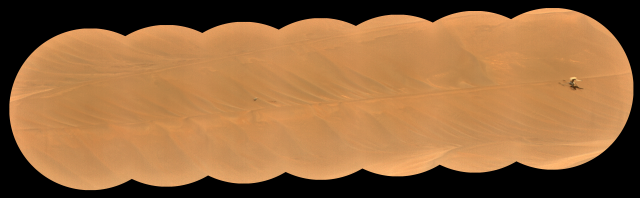
NASA’s Ingenuity Mars Helicopter Team Says Goodbye … for Now
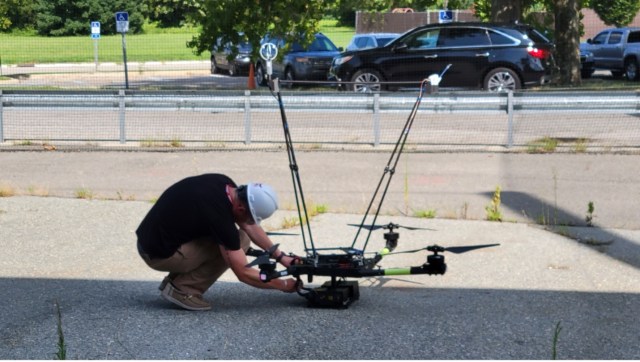
NASA Langley Team to Study Weather During Eclipse Using Uncrewed Vehicles
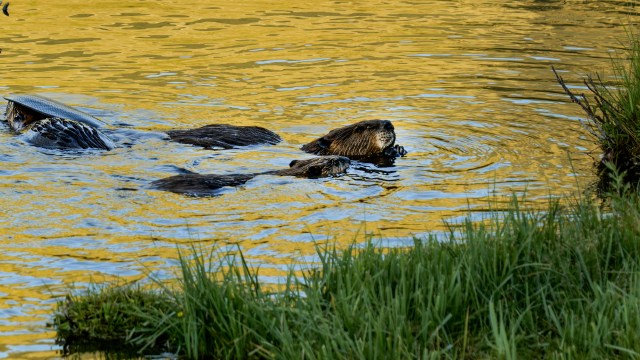
NASA Data Helps Beavers Build Back Streams
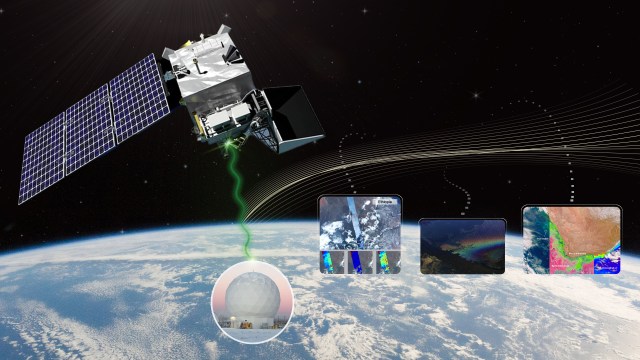
NASA’s Near Space Network Enables PACE Climate Mission to ‘Phone Home’
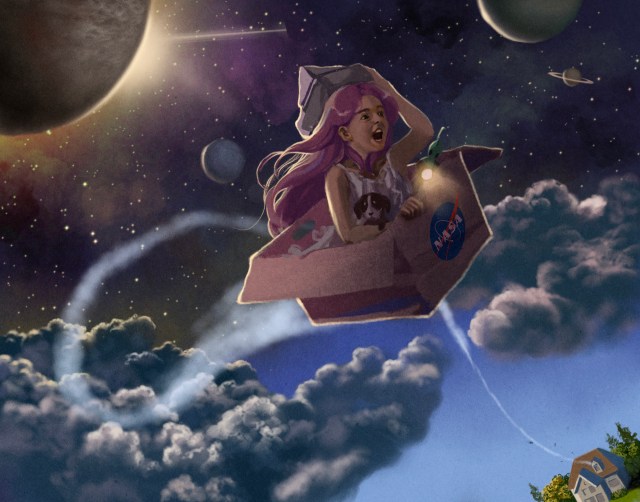
Washington State High Schooler Wins 2024 NASA Student Art Contest
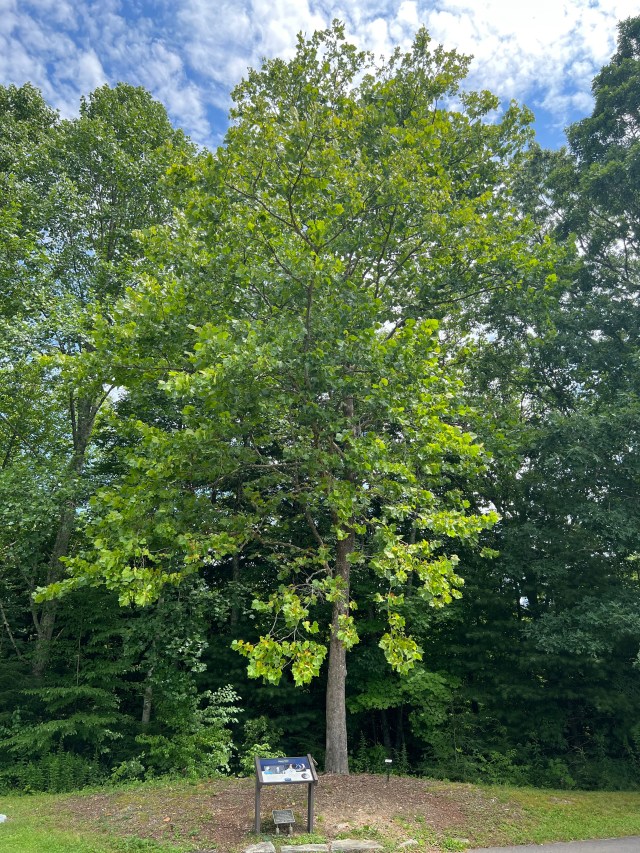
NASA STEM Artemis Moon Trees
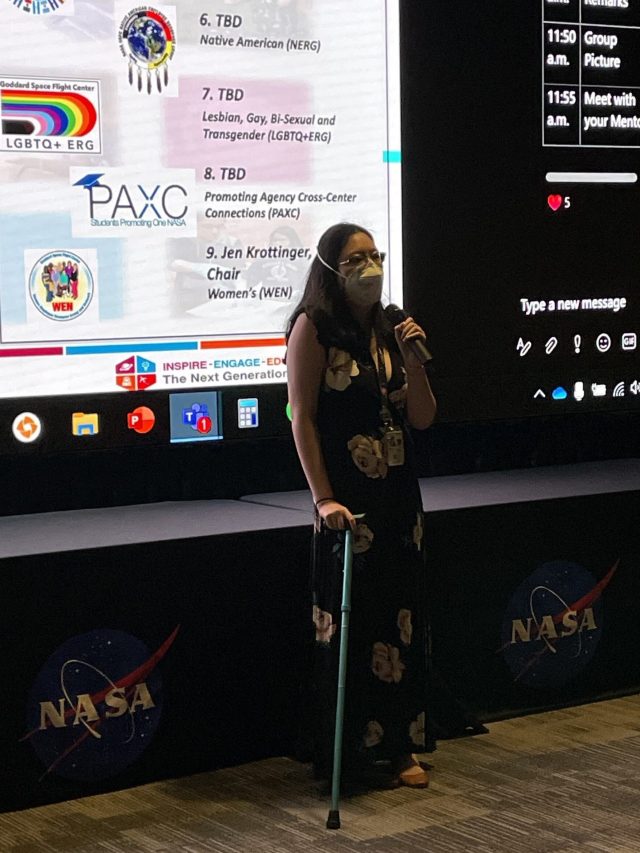
Kiyun Kim: From Intern to Accessibility Advocate
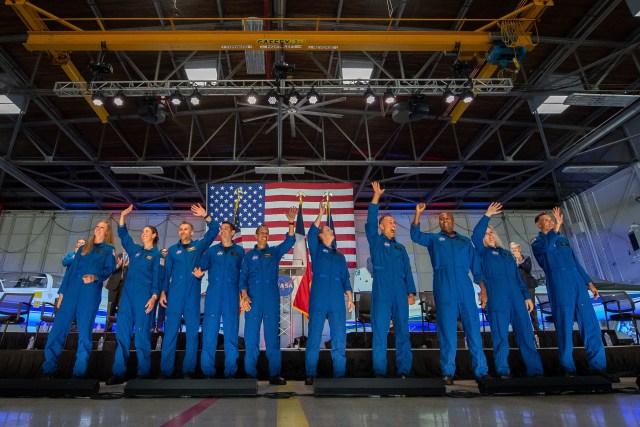
Diez maneras en que los estudiantes pueden prepararse para ser astronautas
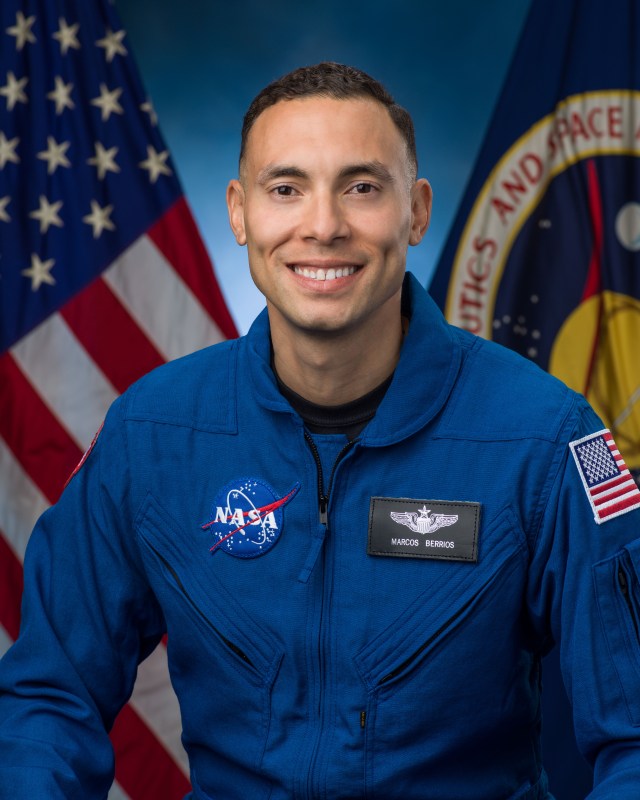
Astronauta de la NASA Marcos Berríos
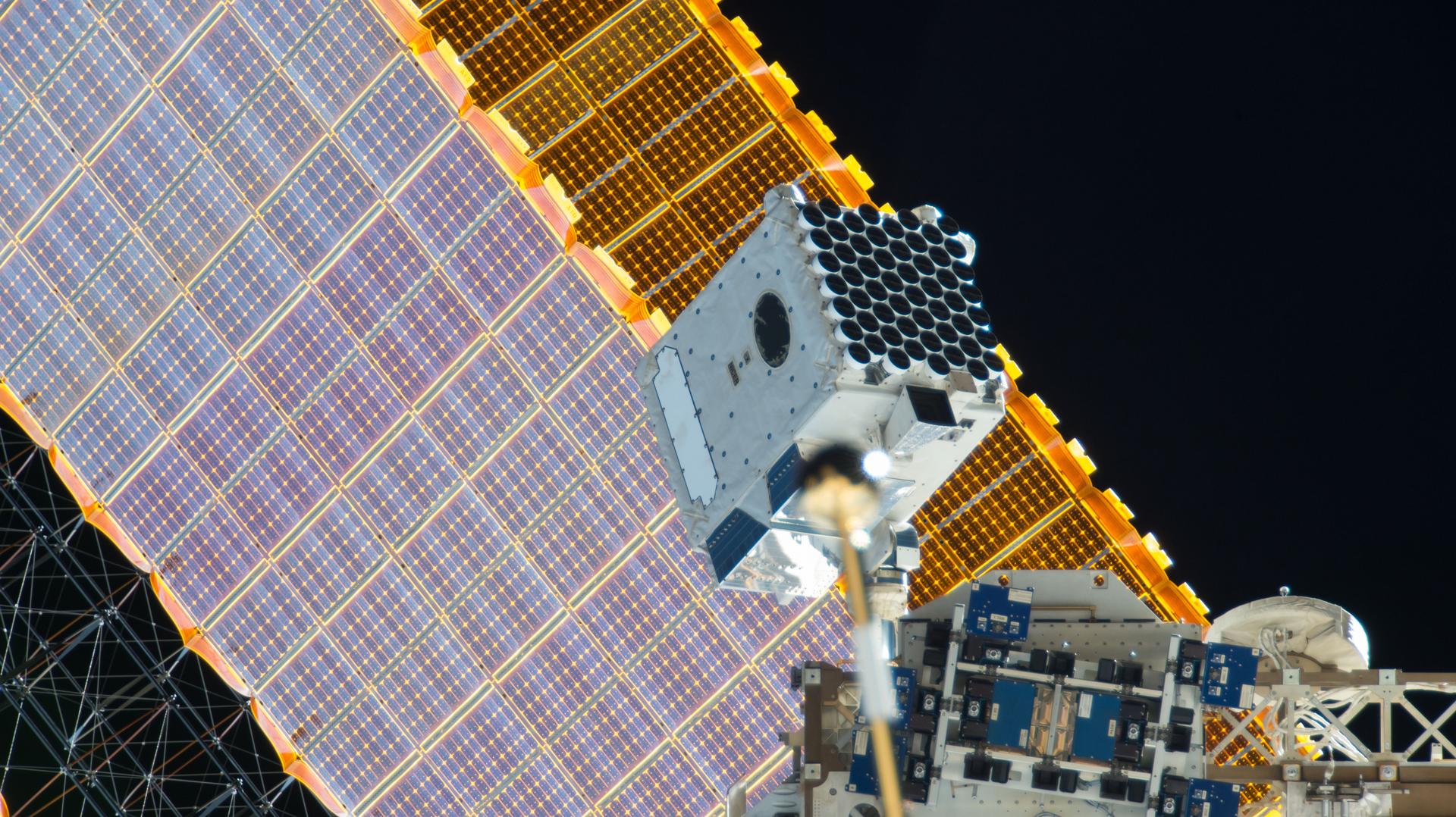
Resultados científicos revolucionarios en la estación espacial de 2023
Take a virtual field trip with access mars.
Educators, Students
Grade Levels
Grades K-4, Grades 5-8, Grades 9-12, Higher Education, Informal Education
Space Science, Technology, Missions to Planets and Moons, Virtual Reality
Other Multimedia, Play and Learn
Are you looking for ways to enhance your online learning? Take remote learning to a new level with free virtual and augmented reality experiences. Use Access Mars to take a field trip to the Red Planet. Visit the Mars Curiosity rover and see important landmarks on the planet’s surface. Users need a cellphone or computer and an internet connection for this interactive, narrated experience.
Planets Take Virtual Shape on Earth with NASA Knowledge and Imagery
Mars - JPL Travel Poster
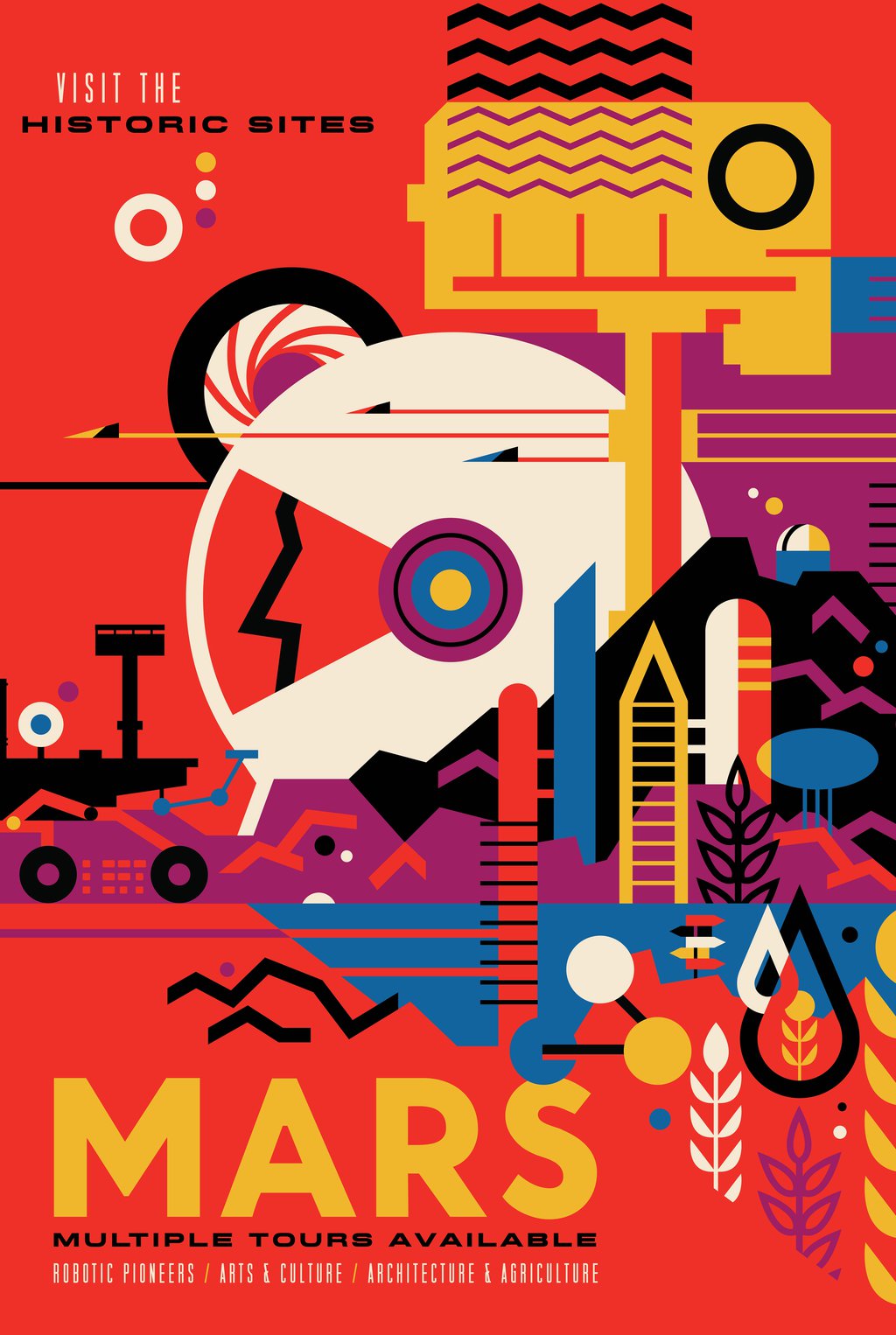
NASA's Mars Exploration Program seeks to understand whether Mars was, is, or can be a habitable world. Missions like Mars Pathfinder, Mars Exploration Rovers, Mars Science Laboratory and Mars Reconnaissance Orbiter, among many others, have provided important information in understanding of the habitability of Mars. This poster imagines a future day when we have achieved our vision of human exploration of Mars and takes a nostalgic look back at the great imagined milestones of Mars exploration that will someday be celebrated as “historic sites.”
Visions of the Future Poster Series
Mars tourism
The possibility of Mars tourism is technology dependent and will vary with time.
- A key economic driver is the cost, if it costs hundreds of thousands of dollars to visit Mars, the number of tourists will be very low. If it costs a few tens of thousands of dollars, then a significant number of people might choose to visit Mars.
- A second driver is time. Travel times to Mars are significant, and a round trip might take a few years, requiring significant commitment.
- An essential precondition is the existence of an interplanetary spaceship.
- Good radiation shielding on the spaceship to minimize the danger of DNA damage and cancer is required in the long term. The notions of risk and informed consent may come into play for early developments.
Early Mars tourism
Until transportation prices go down significantly, it will be an exotic challenge for rich extreme tourists from Earth . An all-inclusive tour to Mars might consist of an extended space travel (back and forth), a temporary integration in the Martian population , optionally with hard work experience and likely include a surface exploration tour. A very high class working holiday, in a sense.
Later Mars tourism
As travel cost go down, the number of tourists should increase. High velocity travel using fusion drives, as part of a large solar system wide society, might eventually allow for routine tourism that is not limited by the synodic period .
- Tourism can be part of a business model for investors and can be part of the Earth-Mars Trade .
- Any enterprise can be profitable if there is demand and a possibility of profit. Tourism might cost millions of dollars per person but if there are takers, then a business plan is possible. Mass market tourism may not be particularly profitable even for a larger market. However, part of the profits from an early stage of tourism might be used to finance the development of lower cost transportation and housing systems.
- Tourism in 2022 is not possible as there is no vehicle capable of providing the service. However, the eventual availability of the SpaceX Starship will create an opportunity for tourism towards Mars.
- Tourism and exploration are similar activities for the initial development of Mars, as long as there is no military or commercial component to the venture. The use of public funds would support exploration, and as long as the scientific return are significant, exploration is probably a better description than tourism for the first travelers towards Mars, even if self funded.
- Renewable rocket technology changes the costs from hundreds of millions of dollars per trip to merely millions.
- Commerce and Economics
- This page was last edited on 30 September 2022, at 08:16.
- Privacy policy
- About Marspedia
- Disclaimers
Navigation menu
Personal tools.
- Request account
- View source
- Needed Articles
- Recent changes
- Random page
Participating Orgs
- The Mars Society
- Mars Foundation
- The Moon Society
- What links here
- Related changes
- Special pages
- Printable version
- Permanent link
- Page information
- Cite this page
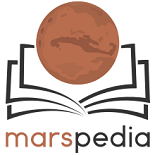
Thank you for visiting nature.com. You are using a browser version with limited support for CSS. To obtain the best experience, we recommend you use a more up to date browser (or turn off compatibility mode in Internet Explorer). In the meantime, to ensure continued support, we are displaying the site without styles and JavaScript.
- View all journals
- My Account Login
- Explore content
- About the journal
- Publish with us
- Sign up for alerts
- Review Article
- Open access
- Published: 05 November 2020
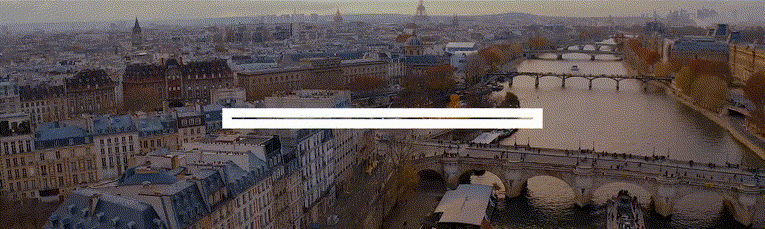
Red risks for a journey to the red planet: The highest priority human health risks for a mission to Mars
- Zarana S. Patel ORCID: orcid.org/0000-0003-0996-6381 1 , 2 ,
- Tyson J. Brunstetter 3 ,
- William J. Tarver 2 ,
- Alexandra M. Whitmire 2 ,
- Sara R. Zwart 2 , 4 ,
- Scott M. Smith 2 &
- Janice L. Huff ORCID: orcid.org/0000-0003-4236-7698 5
npj Microgravity volume 6 , Article number: 33 ( 2020 ) Cite this article
93k Accesses
147 Citations
213 Altmetric
Metrics details
- Cardiovascular diseases
- Eye diseases
- Neurological disorders
- Psychiatric disorders
NASA’s plans for space exploration include a return to the Moon to stay—boots back on the lunar surface with an orbital outpost. This station will be a launch point for voyages to destinations further away in our solar system, including journeys to the red planet Mars. To ensure success of these missions, health and performance risks associated with the unique hazards of spaceflight must be adequately controlled. These hazards—space radiation, altered gravity fields, isolation and confinement, closed environments, and distance from Earth—are linked with over 30 human health risks as documented by NASA’s Human Research Program. The programmatic goal is to develop the tools and technologies to adequately mitigate, control, or accept these risks. The risks ranked as “red” have the highest priority based on both the likelihood of occurrence and the severity of their impact on human health, performance in mission, and long-term quality of life. These include: (1) space radiation health effects of cancer, cardiovascular disease, and cognitive decrements (2) Spaceflight-Associated Neuro-ocular Syndrome (3) behavioral health and performance decrements, and (4) inadequate food and nutrition. Evaluation of the hazards and risks in terms of the space exposome—the total sum of spaceflight and lifetime exposures and how they relate to genetics and determine the whole-body outcome—will provide a comprehensive picture of risk profiles for individual astronauts. In this review, we provide a primer on these “red” risks for the research community. The aim is to inform the development of studies and projects with high potential for generating both new knowledge and technologies to assist with mitigating multisystem risks to crew health during exploratory missions.
Similar content being viewed by others
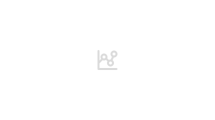
Selected discoveries from human research in space that are relevant to human health on Earth
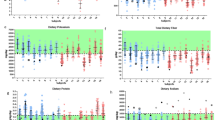
Impact of diet on human nutrition, immune response, gut microbiome, and cognition in an isolated and confined mission environment
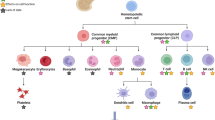
Next generation of astronauts or ESA astronaut 2.0 concept and spotlight on immunity
Introduction.
Spaceflight is a dangerous and demanding endeavor with unique hazards and technical challenges. Ensuring the overall safety of the crew—their physical and mental health and well-being—are vital for mission success. These are large challenges that are further amplified as exploration campaigns extend to greater distances into our solar system and for longer durations. The major health hazards of spaceflight include higher levels of damaging radiation, altered gravity fields, long periods of isolation and confinement, a closed and potentially hostile living environment, and the stress associated with being a long distance from mother Earth. Each of these threats is associated with its own set of physiological and performance risks to the crew (Fig. 1a ) that must be adequately characterized and sufficiently mitigated. Crews do not experience these stressors independently, so it is important to also consider their combined impact on human physiology and performance. This “space exposome” is a unifying framework that reflects the interaction of all the environmental impacts on the human body (Fig. 1b ) and, when combined with individual genetics, will shape the outcomes of space travel on the human system 1 , 2 .
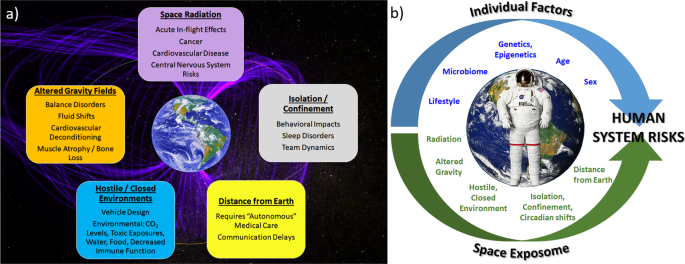
a The key threats to human health and performance associated with spaceflight are radiation, altered gravity fields, hostile and closed environments, distance from Earth, and isolation and confinement. From these five hazards stem the health and performance risks studied by NASA’s Human Research Program. b The space exposome considers the summation of an individual’s environmental exposures and their interaction with individual factors such as age, sex, genomics, etc. - these interactions are ultimately responsible for risks to the human system. Images used in this figure are courtesy of NASA.
The NASA Human Research Program (HRP) aims to develop and provide the knowledge base, technologies, and countermeasure strategies that will permit safe and successful human spaceflight. With agency resources and planning directed toward extended missions both within low Earth orbit (LEO) and outside LEO (including cis-lunar space, lunar surface operations, a lunar outpost, and exploration of Mars) 3 , HRP research and development efforts are focused on mitigation of over 30 categories of health risks relevant to these missions. The HRP’s current research strategy, portfolio, and evidence base are described in the HRP Integrated Research Plan (IRP) and are available online in the Human Research Roadmap, a managed tool used to convey these plans ( https://humanresearchroadmap.nasa.gov/ ). To determine research priorities, NASA uses an evidence-based risk approach to assess the likelihood and consequence (LxC), which gauges the level of each risk for a set of standard design reference missions (Fig. 2 ) 4 . Risks are assigned a rating for their potential to impact in-mission crew health and performance and for their potential to impact long-term health outcomes and quality of life. “Red” risks are those that are considered the highest priority due to their greatest likelihood of occurrence and their association with the most significant risks to crew health and performance for a given design reference mission (DRM). Risks rated “yellow” are considered medium level risks and are either accepted due to a very low probability of occurrence, require in-mission monitoring to be accepted, or require refinement of standards or mitigation strategies in order to be accepted. Risks rated “green” are considered sufficiently controlled either due to lower likelihood and consequence or because the current knowledge base provides sufficient mitigation strategies to control the risk to an acceptable level for that DRM. Milestones and planned program deliverables intended to move a risk rating to an acceptable, controlled level are detailed in a format known as the path to risk reduction (PRR) and are developed for each of the identified risks. The most recent IRP and PRR documents are useful resources for investigators during the development of relevant research approaches and proposals intended for submission to NASA HRP research announcements ( https://humanresearchroadmap.nasa.gov/Documents/IRP_Rev-Current.pdf ).
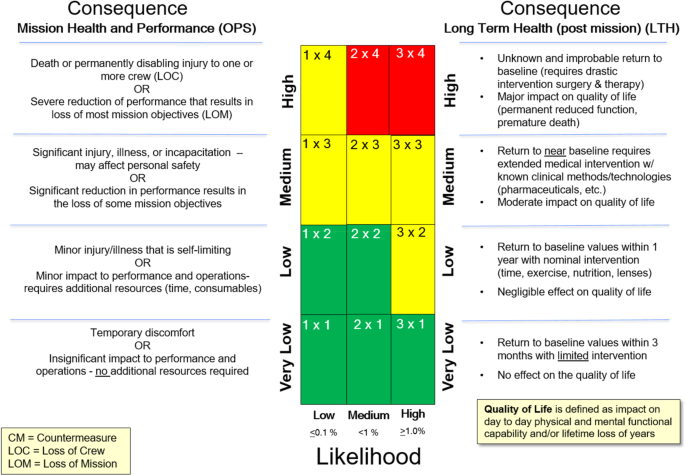
NASA uses an evidence-based approach to assess likelihood and consequence for each documented human system risk. The matrix used for classifying and prioritizing human system risks has two sets of consequences—the left side shows consequences for in-mission risks while the right side is used to evaluate long-term health consequences (Romero and Francisco) 4 .
This work reviews HRP-defined high priority “red” risks for crew health on exploration missions: (1) space radiation health effects that include cancer, cardiovascular disease, and cognitive decrements (2) Spaceflight-Associated Neuro-ocular Syndrome (3) behavioral health and performance decrements, and (4) inadequate food and nutrition. The approaches used to address these risks are described with the aim of informing potential NASA proposers on the challenges and high priority risks to crew health and performance present in the spaceflight environment. This should serve as a primer to help individual proposers develop projects with high potential for generating both new knowledge and technology to assist with mitigating risks to crew health during exploratory missions.
Space radiation health risks
Outside of the Earth’s protective magnetosphere, crews are exposed to pervasive, low dose-rate galactic cosmic rays (GCR) and to intermittent solar particle events (SPEs) 5 . Exposures from GCR are from high charge (Z) and energy (HZE) ions, high-energy protons, and secondary protons, neutrons, and fragments produced by interactions with spacecraft shielding and human tissues. The main components of an SPE are low-to-medium energy protons. In LEO, the exposures are from GCR modulated by the Earth’s magnetic field and from trapped protons in the South Atlantic Anomaly. The absorbed doses for crews on the International Space Station (ISS) on 6- to 12-month missions range from ~30 to 120 mGy. Outside of LEO, without the protection offered by the Earth’s magnetosphere, absorbed radiation doses will be significantly higher. Estimates for a 1 year stay on the lunar surface range from 100 to 120 mGy, and 300 to 450 mGy for an ~3-year Mars mission (transit and surface stay) 6 . The exact dose a crewmember will receive is highly dependent on exact parameters of a given mission, such as detailed vehicle and habitat designs, and mission location and duration 7 . Time in the solar cycle is also a large factor contributing to crew exposure, with highest GCR exposure occurring during periods of minimum solar activity. The lowest GCR exposures occur during periods of maximum solar activity when the heightened magnetic activity of the Sun diverts some cosmic rays; however, during maximum solar activity, the probability of an SPE is higher 8 , 9 . SPEs, which vary in the magnitude and frequency, will obviously also contribute to total mission doses so it is important to note that total mission exposures are only estimates. Further information on the space radiation environment that astronauts will experience is discussed in Simonsen et al. 5 and Durante and Cucinotta 10 .
An important consideration for risk assessment is that the types of radiation encountered in space are very different from the types of radiation exposure we are familiar with here on Earth. HZE ions, although a small fraction of the overall GCR spectrum compared to protons, are more biologically damaging. They differ from terrestrial forms of radiation, such as X-rays and gamma-rays, in both the amount (dose) of exposure as well as in the patterns of DNA double-strand breaks and oxidative damage that they impart as they traverse through tissue and cells (Fig. 3 ) 5 . The highly energetic HZE particles produce complex DNA lesions with clustered double-stranded and single-stranded DNA breaks that are difficult to repair. This damage leads to distinct cellular behavior and intracellular signaling patterns that may be associated with altered disease outcomes compared to those for terrestrial sources of radiation 11 , 12 , 13 . As an example, persistently high levels of oxidative damage are observed in the intestine from mice examined 1 year after exposure to 56 Fe-ion radiation compared to gamma radiation and unirradiated controls 14 , 15 . The higher levels of residual oxidative damage in HZE ion-irradiated tissue is significant because of the association of oxidative stress and damage with the etiology of many human diseases, including cancer, cardiovascular and late neurodegenerative disorders. These types of alterations are believed to contribute to the higher biological effectiveness of HZE particles 10 , 11 .
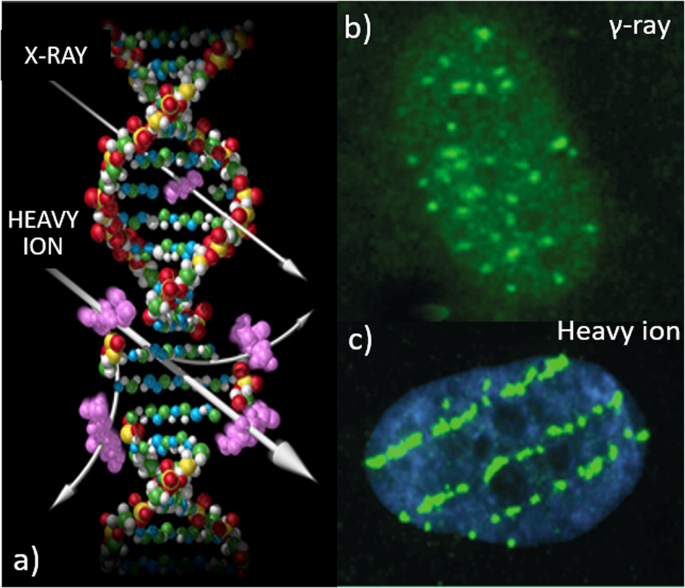
a HZE ions produce dense ionization along the particle track as they traverse a tissue and impart distinct patterns of DNA damage compared to terrestrial radiation such as X-rays. γH2AX foci (green) illuminate distinct patterns of DNA double-strand breaks in nuclei of human fibroblast cells after exposure to b gamma-rays, with diffuse damage, and c HZE ions with single tracks. Image credits: NASA ( a ) and Cucinotta and Durante 97 ( b and c ).
Within the HRP, the Space Radiation Element (SRE) has developed a research strategy involving both vertical translation and horizontal integration, as well as products focused on mitigating space radiation risks across all phases of a mission. Vertical translation involves the integration of benchtop research with preclinical studies and clinical data. Horizontal integration involves a multidisciplinary approach that includes a range of expertize from physicians to clinicians, epidemiologists to computational modelers 16 . The suite of tools includes computational models of the space radiation environment, mission design tools, models for risk projection, and tools and technologies for accurate simulation of the space radiation environment for radiobiology investigations. Ongoing research is focused on radiation quality, age, sex, and healthy worker effects, medical countermeasures to reduce or eliminate space radiation health risks, understanding the complex nature of individual sensitivity, identification and validation of biomarkers (translational, surrogate, predictive, etc.) and integration of personalized risk assessment and mitigation approaches. Owing to the lack of human data for heavy ion exposure on Earth and the complications of obtaining reliable data for space radiation health effects from flight studies, SRE conducts research at the NASA Space Radiation Laboratory (NSRL) at Brookhaven National Laboratory. The NSRL is a ground-based analog for space radiation, where a beamline and associated experimental facilities are dedicated to the radiobiology and physics of a range of ions from proton and helium ions to the typical GCR ions such as carbon, silicon, titanium, oxygen, and iron 5 , 17 , 18 .
Radiation carcinogenesis
Central evidence for association between radiation exposure and the development of cancer and other non-cancer health effects comes from epidemiological studies of humans exposed to radiation 19 , 20 , 21 , 22 . Scaling factors are used by NASA and other space agencies in the analysis of cancer (and other risks) to account for differences between terrestrial radiation exposures and cosmic radiation exposures 23 . The risk of radiation carcinogenesis is considered a “red” risk for exploration-class missions due to both the high likelihood of occurrence, as well as the high potential for detrimental impact on both quality of life and disease-free survival post flight. The major cancers of concern are epithelial in origin (particularly cancers of the lung, breast, stomach, colon, and bladder), as well as leukemias ( https://humanresearchroadmap.nasa.gov/Evidence/reports/Cancer.pdf ). Owing to the lack of human epidemiology directly relevant to the types of radiation found in space, current research utilizes a translational approach that incorporates rodent and advanced human cell-based model systems exposed to space radiation simulants along with comparison of molecular pathways across these systems to the human.
A key question that impacts risk assessment and mitigation is how HZE tumors compare to either radiogenic tumors induced by ground-based radiation or spontaneous tumors. As a unifying concept, NASA studies have sought to examine how space radiation exposure modifies the key genetic and epigenetic modifications noted as the hallmarks of cancer (Fig. 4 ) 24 , 25 , 26 , 27 . This approach provides data for development of translational scaling factors (relative biological effectiveness values, quality factors, dose-rate effectiveness factor) to relate the biological effects of space radiation to effects from similar exposures to ground-based gamma- and X-rays and extrapolation of results to large human epidemiology cohorts. It also supports acquisition of mechanistic information required for successful identification and implementation of medical countermeasure strategies to lower this risk to an acceptable posture for space exploration, and it is relevant for the future development of biologically based dose-response models and integrated systems biology approaches 25 . Cancer is a long-term health risk and although it is rated as “red”, most research in this area is currently delayed, as HRP research priorities focus on in-mission risks.
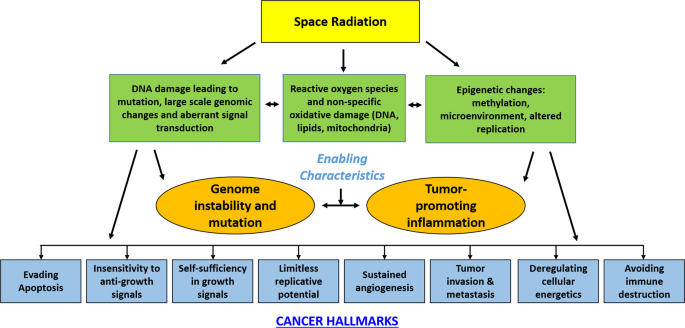
Shown are the enabling characteristics and possible mechanisms of radiation damage that lead to these changes observed in all human tumors. (Adapted from Hanahanand Weinberg) 24 .
Risk of cardiovascular disease and other degenerative tissue effects from radiation exposure and secondary spaceflight stressors
A large number of degenerative tissue (non-cancer) adverse health outcomes are associated with terrestrial radiation exposure, including cardiovascular and cerebrovascular diseases, cataracts, digestive and endocrine disorders, immune system decrements, and respiratory dysfunction ( https://humanresearchroadmap.nasa.gov/Evidence/other/Degen.pdf ). For cardiovascular disease (CVD), a majority of the evidence comes from radiotherapy cohorts receiving high-dose mediastinal exposures that are associated with an increased risk for heart attack and stroke 28 . Recent evidence shows risk at lower doses (<0.5 Gy), with an estimated latency of 10 years or more 29 , 30 , 31 . For a Mars mission, preliminary estimates suggest that circulatory disease risk may increase the risk of exposure induced death by ~40% compared to cancer alone 32 . NASA is also concerned about in-flight risks to the cardiovascular system ( https://humanresearchroadmap.nasa.gov/Evidence/other/Arrhythmia.pdf ), when considering the combined effects of radiation exposure and other spaceflight hazards (Fig. 5 ) 33 . The Space Radiation Element is focused on accumulating data specific to the space radiation environment to characterize and quantify the magnitude of the degenerative disease risks. The current efforts are on establishing dose thresholds, understanding the impact of dose-rate and radiation quality effects, uncovering mechanisms and pathways of radiation-associated cardiovascular and cerebrovascular diseases, and subsequent risk modeling for astronauts. Uncovering the mechanistic underpinnings governing disease processes supports the development of specific diagnostic and therapeutic approaches, is a necessary step in the translation of insights from animal models to humans, and is the basis of personalized medicine approaches.
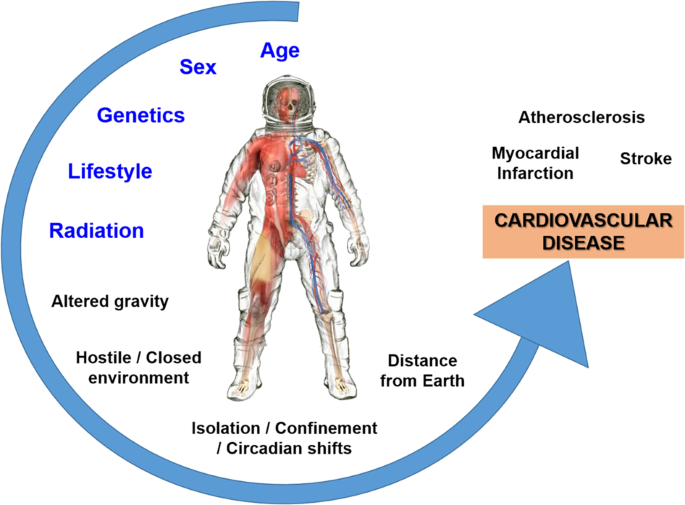
In blue are the known risk factors for CVD and in black are the other spaceflight stressors that may also contribute to disease development. Image used in this figure is courtesy of NASA.
This information will provide a means to reduce the uncertainty in current permissible exposure limits (PELs), quantify the impact to disease-free survival years, and determine if additional protection or mitigation strategies are required. The research portfolio includes evaluation of current clinical standard-of-care biomarkers for their relevance as surrogate endpoints for radiation-induced disease outcomes. Studies are also addressing the possible role of chronic inflammation and increased oxidative stress in the etiology of radiation-induced CVD, as well as identification of key events in disease pathways, like endothelial dysfunction, that will guide the most effective medical countermeasures. Products include validated space radiation PELs, models to quantify the risk of CVD for the astronaut cohort, and countermeasures and evidence to inform development of appropriate recommendations to clinical guidelines for diagnosis and mitigation of this risk.
Elucidating the role that radiation plays in degenerative disease risks is problematic because multiple factors, including lifestyle and genetic influences, are believed to play a major role in the etiology of these diseases. This confounds epidemiological analyses, making it difficult to detect significant differences from background disease without a large study population 34 . This issue is especially significant in astronaut cohorts because those studies have small sample sizes 35 . There is also a general lack of experimental data that specifically addresses the role of radiation at low, space-relevant doses 36 . Selection of experimental models needs to be carefully considered and planned to ensure that the cardiovascular disease mechanisms and study endpoints are clinically relevant and translatable to humans 37 , 38 . Combined approaches using data from wildtype and genetically modified animal models with accelerated disease development will likely be necessary to elucidate mechanisms and generate the body of knowledge required for development of accurate permissible exposure limits, risk assessment models, and to develop effective mitigation approaches.
Risk of acute (in-flight) and late CNS effects from space radiation exposure
The possibility of acute (in-flight) and late risks to the central nervous system (CNS) from GCR and SPEs are concerns for human exploration of space ( https://humanresearchroadmap.nasa.gov/Evidence/reports/CNS.pdf ). Acute CNS risks may include altered neurocognitive function, impaired motor function, and neurobehavioral changes, all of which may affect human health and performance during a mission. Late CNS risks may include neurological disorders such as Alzheimer’s disease, dementia, or accelerated aging. Detrimental CNS changes from radiation exposure are observed in humans treated with high doses of gamma-rays or proton beams and are supported by a large body of experimental evidence showing neurocognitive and behavioral effects in animal models exposed to lower doses of HZE ions. Rodent studies conducted with HZE ions at low, mission-relevant doses and time frames show a variety of structural and functional alterations to neurons and neural circuits with associated performance deficits 39 , 40 , 41 , 42 , 43 , 44 . Fig. 6 shows an example of changes in dendritic spine density following HZE ion radiation. However, the significance and relationship of these results to adverse outcomes in astronauts is unclear, as similar decrements are not seen with comparable doses of terrestrial radiation. Therefore, scaling to human epidemiology data, as is done for cancer and cardiovascular disease, is not possible. It is also important to note that to date, no radiation-associated clinically significant operational or long-term deficits have been identified in astronauts receiving similar doses via long-duration ISS missions. It is clear that further development of standardized translational models, research paradigms, and appropriate scaling approaches are required to determine significance in humans 45 , 46 . In addition, elucidation of how space radiation interacts with other mission hazards to impact neurocognitive and behavioral health and performance is critical to defining appropriate PELs and countermeasure strategies. The current research approach is a combined effort of SRE, the human factors and behavioral performance element, and the human health countermeasures element in support of an integrated CBS (CNS/behavioral medicine/sensorimotor) plan ( https://humanresearchroadmap.nasa.gov/Risks/risk.aspx?i=99 ). Further information on this risk area is presented below in the Behavioral Health and Performance section and can also be found at the Human Research Roadmap.
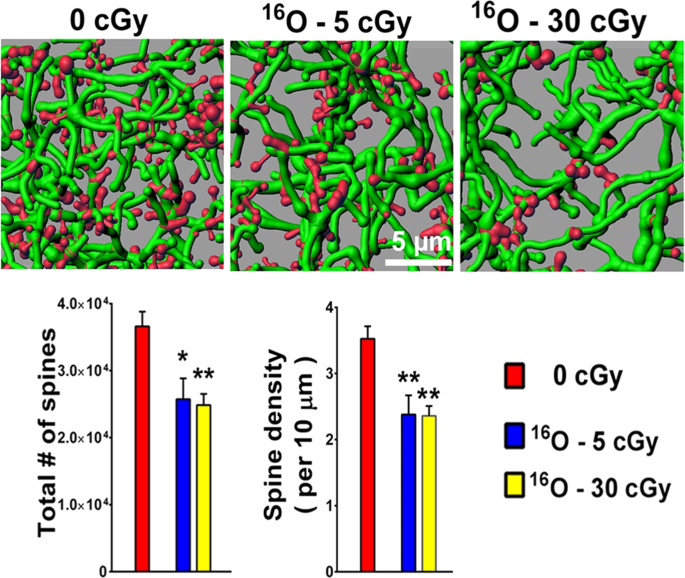
Representative digital images of 3D reconstructed dendritic segments (green) containing spines (red) in unirradiated (0 cGy) and irradiated (5 and 30 cGy) mice brains. Multiple comparisons show that total spine numbers (left bar chart) and spine density (right bar chart) are significantly reduced after exposure to 5 or 30 cGy of 16 O particles. Data are expressed as mean ± SEM. * P < 0.05, ** P < 0.01 versus control; ANOVA. Adapted from Parihar et al. 39 . Permission to reproduce open-source figure per the Creative Commons Attribution 4.0 International License. https://creativecommons.org/licenses/by/4.0 .
To summarize, the health risks posed by the omnipresent exposure to space radiation are significant and include the “red” risks of cancer, cardiovascular diseases, and cognitive and behavioral decrements. While research on the late health risk of cancer is currently delayed, research on the in-flight effects of radiation on the cardiovascular system and CNS within the context of the space exposome are considered the highest priority and are the focus of investigations. Major knowledge gaps include the effects of radiation quality, dose-rate, and translation from animal models to human systems and evaluation of the requirement for medical countermeasure approaches to reduce the risk.
Spaceflight-Associated Neuro-ocular Syndrome
The Risk of Spaceflight-Associated Neuro-ocular Syndrome (SANS), originally termed the Risk of Vision Impairment Intracranial Pressure (VIIP), was first discovered about 15 years ago. VIIP was the original name used because the syndrome most noticeably affects a crewmember’s eyes and vision, and its signs can appear like those of the terrestrial condition idiopathic intracranial hypertension (IIH; which is due to increased intracranial pressure). Over time, it was realized that the VIIP name required an update. Most notably, SANS is not associated with the classic symptoms of increased intracranial pressure in IIH (e.g., severe headaches, transient vision obscurations, double vision, pulsatile tinnitus), and it has never induced vision changes that meet the definition of vision impairment, as defined by the National Eye Institute. In 2017, VIIP was renamed to SANS, a term that welcomes additional pathogenesis theories and serves as a reminder that this syndrome could affect the CNS well beyond the retina and optic nerve.
SANS presents with an array of signs, as documented in the HRP Evidence Report ( https://humanresearchroadmap.nasa.gov/evidence/reports/SANS.pdf ). Primarily, these include edema (swelling) of the optic disc and retinal nerve fiber layer (RNFL), chorioretinal folds (wrinkles in the retina), globe flattening, and refractive error shifts 47 . Flight duration is thought to play a role in the pathogenesis of SANS, as nearly all cases have been diagnosed during or immediately after long-duration spaceflight (i.e., missions of 30 days duration or longer), although signs have been discovered as early as mission day 10 48 . Because of SANS, ocular data are nominally collected during ISS missions. For most ISS crewmembers, this testing includes optical coherence tomography (OCT), retinal imaging, visual acuity, a vision symptom questionnaire, Amsler grid, and ocular ultrasound (Fig. 7 ).
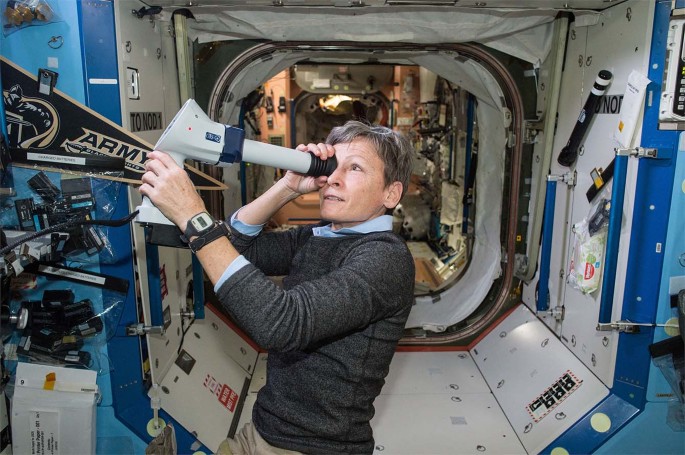
Image courtesy of NASA.
From a short-term perspective (e.g., a 6-month ISS deployment), SANS presents four main risks to crewmembers and their mission: optic disc edema (ODE), chorioretinal folds, shifts in refractive error, and globe flattening 49 . Approximately 69% of the US crewmembers on the ISS experience a > 20 µm increase in peripapillary retinal thickness in at least one eye, indicating the presence of ODE. With significant levels of ODE, a crewmember can experience an enlargement of his/her blind spots and a corresponding loss in visual function. To date, blind spots are uncommon and have not had an impact on mission performance.
If chorioretinal folds are severe enough and located near the fovea (the retina associated with central vision), a crewmember may experience visual distortions or reduced visual acuity that cannot be corrected with glasses or contact lenses, as noted in the SANS Evidence Report. Despite a prevalence of 15–20% in long-duration crewmembers, chorioretinal folds have not yet impacted astronauts’ visual performance during or after a mission. An on-orbit shift in refractive error is due to a shortening of the eye’s axial length (distance between the cornea and the fovea), and it occurs in about 16% of crewmembers during long-duration spaceflight. This risk is mitigated by providing deploying crewmembers with several pairs of “Space Anticipation Glasses” (or contact lenses) of varying power. On-orbit, the crewmember can then select the appropriate lenses to restore best-corrected visual acuity. Approximately 29% of long-duration crewmembers experience a posterior eyeball flattening, which is typically centered around the insertion of the optic nerve into the globe. Globe flattening can induce chorioretinal folds and shifts in refractive error, posing the respective risks described above.
From a longer-term perspective, SANS presents two main risks to crewmembers: ODE and chorioretinal folds. It is unknown if a multi-year spaceflight (e.g., a Mars mission) will be associated with a higher prevalence, duration, and/or severity of ODE compared to what has been experienced onboard the ISS. Since the retina and optic nerve are part of the CNS, if ODE is severe enough, the crewmember risks a permanent loss of optic nerve and RNFL tissue and thus, a permanent loss of visual function. It should be stressed that no SANS-related permanent loss of visual function has yet been discovered in any astronauts.
For choroidal folds, improvement generally occurs post-flight in affected crewmembers; however, significant folds can persist for 10 or more years after long-duration missions. Using MultiColor Imaging and autofluorescence capabilities of the latest OCT device, it was discovered recently that one crewmember’s longstanding (>5 years) post-flight choroidal folds have induced disruption to its overlying retinal pigment epithelium (RPE) 50 . The RPE is a monolayer of pigmented cells located between the vascular-rich choroid and the photoreceptor outer segments. This layer forms the posterior blood-brain barrier for the retina and is essential for maintaining the health of the posterior retina via the transport of nutrients and fluids, among other key functions. If the RPE is damaged, it could potentially lead to a degeneration of the local retina and progress to vision impairment.
Recent long-duration head-down tilt studies have shown potential for recreating SANS signs in terrestrial cohorts 51 . However, SANS is considered a pathology unique to spaceflight. In microgravity, fluid within the body is free to redistribute uniformly. This means that much of the fluid that normally pools in a person’s feet and legs due to gravity can transfer upward towards the head and cause a general congestion of the cerebral venous system. The central pathogenesis theories of SANS are based on these facts, but the actual cause(s) and pathophysiology of SANS are yet unknown 49 . The most publicized theory for SANS has been that cerebral spinal fluid outflow might be impeded, causing an overall increase in intracranial pressure (ICP) 47 , 52 . Other potential mechanisms (see Fig. 8 ) include cerebral venous congestion or altered folate-dependent 1-carbon metabolism via a cascade of mechanisms that may ultimately increase ICP or affect the response of the eye to fluid shifts 53 , 54 . Potential confounding variables for SANS pathogenesis include resistive exercise, high-sodium dietary intake, and high carbon dioxide levels.
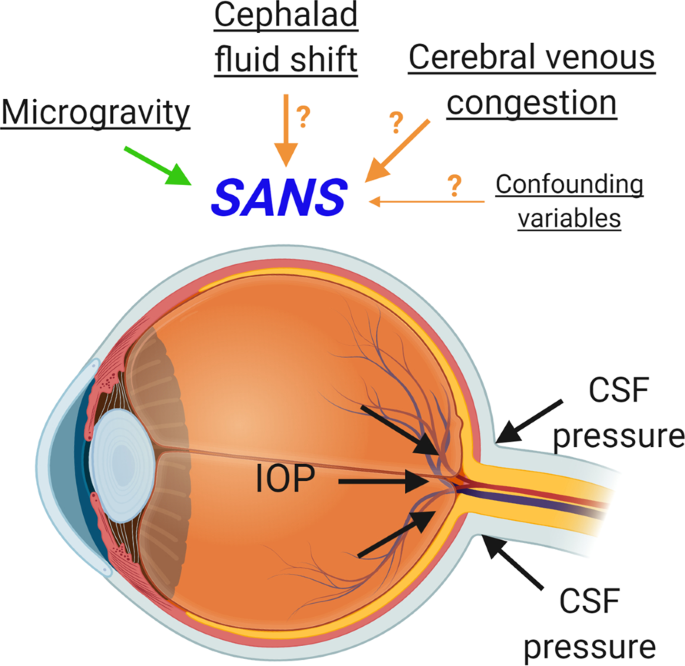
Image created with BioRender.com.
Discovering patterns and trends in the SANS population has been difficult due to the relatively low number of crewmembers who have completed long-duration spaceflight. This is especially true for female astronauts. However, there is now enough evidence to state—emphatically—that SANS is not a male-only syndrome. OCT has been utilized onboard the ISS since late 2013, and it has revolutionized NASA’s ability to objectively detect and monitor SANS and build a high-resolution database of retinal and optic nerve head images. Through this technology, it has been recently discovered that that a majority of long-duration astronauts (including females) present with some level of ODE and engorgement of the choroidal vasculature 48 , 55 . The trends and patterns of these ocular anatomical changes may hold the key to deciphering the pathophysiology of SANS 48 , 55 .
Beginning in 2009 in response to SANS, all NASA crewmembers receive pre- and post-flight 3 Tesla magnetic resonance imaging of the brain and orbits. Based on these images, there is growing evidence that brain structural changes also occur during long-duration spaceflight. Most notably, a 10.7–14.6% ventricular enlargement (i.e., approximately a 2–3 ml increase) has been detected in astronauts and cosmonauts by multiple investigators 56 , 57 , 58 , 59 . On-orbit and post-flight cognitive testing have not revealed any systemic cognitive decrements associated with these anatomical changes. Moreover, additional research is required to determine if spaceflight-associated brain structural changes are related to ocular structural changes (i.e., SANS) or if the two are initiated by a common cause. Thus, until a relationship is established, SANS will be defined by ocular signs.
Future SANS medical operations, research, and surveillance will focus on: 1) determining the pathogenesis of the syndrome, 2) developing small-footprint diagnostic devices for expeditionary spaceflight, 3) establishing effective countermeasures, 4) monitoring for any long-term health consequences, and 5) discovering what factors make certain individuals more susceptible to developing the syndrome.
In summary, SANS is a top risk and priority to NASA and HRP. The primary SANS-related risk is ODE, due to the possibility of permanent vision impairment; however, choroidal folds also present a short- and long-term risk to astronaut vision. Shifts in refractive error are relatively common in long-duration missions, but crewmembers do not experience a loss of visual acuity if adequate correction is available. SANS affects female astronauts, not just males, although it is not yet known if SANS prevalence is equal between the sexes. There are no terrestrial pathologies identical to SANS, including IIH. Long-duration spaceflight is also associated with brain anatomical changes; however, it is not yet known whether these changes are related to SANS. Finally, the pathogenesis of SANS remains elusive; however, the main theories are related to increased intracranial pressure, ocular venous congestion, and individual anatomical/genetic variability.
Behavioral health and performance
The Risk of Adverse Cognitive and Behavioral Conditions and Psychiatric Disorders (BMed) focuses on characterizing and mitigating potential decrements in performance and psychological health resulting from multiple spaceflight hazards, including isolation and distance from earth. Spaceflight radiation is also recognized as contributing factor, particularly relative to a deep space planetary mission. The potential of additive or synergistic effects on the CNS resulting from simultaneous exposures to radiation, isolation and confinement, and prolonged weightlessness, is also of emerging concern ( https://humanresearchroadmap.nasa.gov/Risks/risk.aspx?i=99 ).
The official risk statement in the BMed Evidence Report notes, “ given the extended duration of future missions and the isolated, confined and extreme environments, there is a possibility that (a) adverse cognitive or behavioral conditions will occur affecting crew health and performance; and (b) mental disorders could develop should adverse behavioral conditions be undetected and unmitigated ” ( https://humanresearchroadmap.nasa.gov/Evidence/reports/BMED.pdf ). Primary outcomes for this risk include decrements in cognitive function, operational performance, and psychological and behavioral states, with the development of psychiatric disorders representing the least likely but one of the most consequential outcomes crew could experience in extended spaceflight. BMed is considered a “red” risk for planetary missions, given the long-duration of isolation, extended confinement, and exposure to additional stressors, including increased radiation exposure. The Human Factors and Behavioral Performance Element within HRP utilizes a research strategy that incorporates flight studies on astronauts, research in astronaut-like individuals and teams in ground analogs, and works with the Space Radiation Element to use animal models supporting research on combined spaceflight stressors.
While astronauts successfully accomplish their mission objectives and report very positive experiences living and working in space, some anecdotal accounts from current and past astronauts suggest that psychological adaptation in the long-duration spaceflight environment can be challenging. However, clinically significant operational decrements have not been documented to date, as noted in the BMed Evidence Report. Discrete events that have been documented include accounts of adverse responses to workload by Shuttle payload specialists, and descriptions of ‘hostile’ and ‘irritable’ crew in the 84-day Skylab 4 mission, as well as symptoms of depression reported on Mir by 2 of the 7 NASA astronauts.
Currently, potential stressors affiliated with missions to the ISS include extended periods of high workload and/or schedule shifting, physiological adaptation including fluid shifts caused by weightlessness and possibly, exposure to other environmental factors such as elevated carbon dioxide (see the BMed Evidence Report). While still physically isolated from home, the presence of the ISS in LEO facilitates a robust ground behavioral health and performance support team who offer services such as bi-weekly private psychological conferences and regular delivery of novel goods and surprises from home in crew care packages. Coupled with the relatively ample volume in the ISS, near-constant real-time communication with Earth, new crewmembers rotating periodically throughout missions, and relatively low levels of radiation exposure, —it is expected that behavioral challenges experienced today do not represent those that future crews will face during exploration missions.
Nevertheless, the few completed behavioral studies on the ISS suggest that subjective perceptions of stress increase over time for some crewmembers, as shown by an in-flight study collecting subjective ratings of well-being and objective measures of fatigue 60 . Notably, it was found that astronaut ratings of sleep quality and sleep duration (also measured through visual analog scales) were found to be inversely related to ratings of stress. Another in-flight investigation seeking to characterize behavioral responses to spaceflight is the “Journals” study by Stuster 61 . This investigation provided a systematic approach to examining a rich set of qualitative data by evaluating astronaut journal entries for temporal patterns of across different behavioral states over the course of a mission (Fig. 9 ). Based on findings, some categories suggest temporal patterns while other categories of outcomes do not suggest a pattern relative to time, which may be due to no temporal relationship between outcomes and time, and/or various contextual factors within missions that negate the presence of such a relationship (e.g., visiting crew). An overall assessment by Stuster of negative comments relative to positive comments over time suggests evidence of a third quarter phenomenon in Adjustment alone, a category which reflects individual morale 61 .
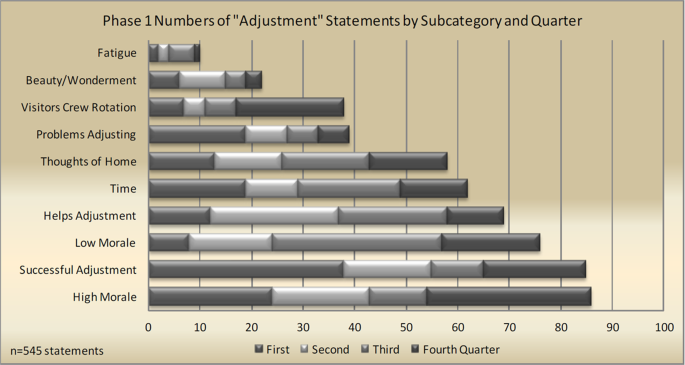
Example bar graph showing distribution of journal entries related to general adjustment to the spaceflight enivronment during each quarter of an ISS mission 61 .
Other in-flight investigations support and expand upon contributors to increased stress on-orbit, including studies documenting reductions in sleep duration 62 , 63 and evaluation of crew responses to habitability and human factors during spaceflight 64 . While no studies have assessed potentially relevant mechanisms for behavioral or other reported symptoms, a recently completed investigation suggests neurostructural changes may be occurring in the spaceflight environment 56 . Magnetic resonance imaging scans were conducted on astronauts pre- and post-flight on both long-duration missions to the ISS or short-duration Shuttle missions. Assessments from a subgroup of participants ( n = 12) showed a slight upward shift of the brain after all long-duration flights but not after short-duration flights ( n = 6), and they also showed narrowing of cerebral spinal fluid spaces at the vertex after all long-duration flights ( n = 6) and in 1 of 6 crew after short-duration flights. A retrospective analysis of free water volume in the frontal, temporal, and occipital lobes before versus after spaceflight suggests alterations in free water distribution 65 . Whether there is a functionally relevant outcome as a result of such changes remains to be determined. Hence, while certain aspects of the spaceflight environment have been shown to increase some behavioral responses (e.g., reduced sleep owing to workload), the direct role of spaceflight-specific factors (such as fluid shifts and weightlessness) on behavioral outcomes or functional performance has not yet been established.
Future long-duration missions will pose threats to behavioral health and performance, such as extreme confinement in a small volume and communication delays, that are distinct from what is currently experienced on missions to the ISS. Analog research is concurrently underway to help further characterize the likelihood and consequence of an adverse behavioral outcome, and the effectiveness of potential countermeasures. Ground analogs, such as the Human Exploration Research Analog (HERA) at NASA Johnson Space Center, provide a test bed where controlled studies of small teams for periods up to 45 days, can be implemented (Fig. 10 ). HERA can be used to provide scenarios and environments analogous to space (e.g., isolation and confinement, communication delays, space food, and daily tasks and schedules) to investigate their effects on behavioral health, human factors, exploration medical capabilities, and communication and autonomy. Research in locations such as Antarctica also offer a unique opportunity to conduct research in less controlled but higher fidelity conditions. In general, these studies show an increased risk in deleterious effects such as decreased mood and increased stress, and in some instances, psychiatric outcomes (see the BMed Evidence Report).
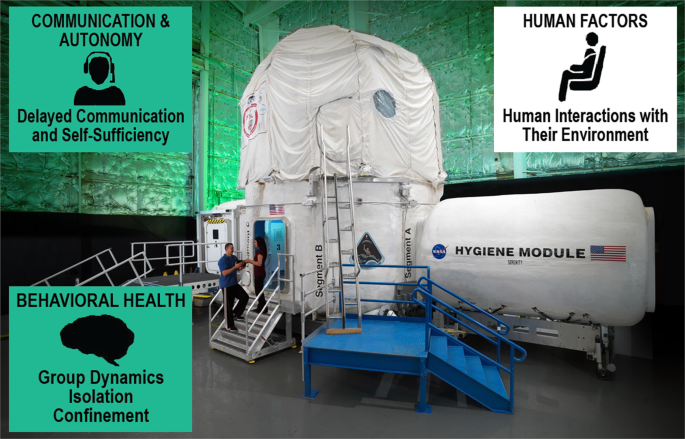
HERA is used to simulate environments and mission scenarios analogous to spaceflight to investigate a variety of behavioral and human factors issues. Images courtesy of NASA.
In 2014, Basner and colleagues 62 completed an assessment of crew health and performance in a 520-day mission at an isolation chamber in Moscow at the Institute for Biomedical Problems (IBMP). During this simulated mission to Mars, the crew of six completed behavioral questionnaires and additional testing weekly. One of six (20%) crew reported depressive symptoms based on the Beck Depression Inventory in 93% of mission weeks, which reached mild-to-moderate levels in >10% of mission weeks. Additional indications of changes in mood were observed via the Profile of Mood States. Additionally, two crewmembers who had the highest ratings of stress and physical exhaustion accounted for 85% of the perceived conflicts, and other crew demonstrated dysregulation in their circadian entrainment and sleep difficulties. Two of the six crewmembers reported no adverse behavioral symptoms during the missions 62 . Building on this work, the NASA HRP and the IBMP have ongoing studies in the SIRIUS project, a series of long-duration ground-analog missions for understanding the effects of isolation and confinement on human health and performance ( http://www.nasa.gov/analogs/nek/about ).
Finally, more recent research in the HERA analog at Johnson Space Center is underway to assess not only individual, psychiatric outcomes but also changes in team dynamics and team performance over time (Fig. 10 ). A recent publication reported that conceptual team performance (e.g., creativity) seems to decrease over time, while performance requiring cognitive function and coordinated action improved 66 . While results from additional team studies in HERA are currently under review, the Teams Risk Evidence Report ( https://humanresearchroadmap.nasa.gov/Evidence/reports/Team.pdf ) provides a thorough overview of the evidence surrounding team level outcomes.
In summary, evidence from spaceflight and spaceflight analogs suggests that the BMed Risk poses a high likelihood and high consequence risk for exploration. Given the possible synergistic effects of prolonged isolation and confinement, radiation exposure, and prolonged weightlessness, mitigating such enhanced risks faced by future crews are of highest priority to the NASA HRP.
Inadequate food and nutrition
Historically, nutrition has driven the success—and often the failure—of terrestrial exploration missions. For space explorers, nutrition provides indispensable sustenance, provides potential countermeasures to some of the negative effects of space travel on human physiology, and also presents a multifaceted risk to the health and safety of astronauts ( https://humanresearchroadmap.nasa.gov/Evidence/other/Nutrition-20150105.pdf ).
At a minimum, the need to prevent nutrient deficiencies is absolute. This was proven on voyages during the Age of Sail, where scurvy—caused by vitamin C deficiency— killed more sailors than all other causes of death. On a closed (or even semi-closed) food system, the risk of nutrient deficiency is increased. On ISS missions, arriving vehicles typically bring some fresh fruits and/or vegetables to the crew. While limited in volume and shelf-life, these likely provide a valuable source of nutrients and phytochemicals every month or two. One underlying concern is that availability of these foods may be mitigating nutrition issues of the nominal food system, and without this external source of nutrients on exploration-class missions, those issues will be more likely to surface.
As a cross-cutting science, nutrition interfaces with many, if not all, physiological systems, along with many of the elements associated with space exploration, including the spacecraft environment (Fig. 11 ). Thus, beyond the basics of preventing deficiency of specific nutrients, at best, nutrition can serve as a countermeasure to mitigate risks to other systems. Conversely, at worst, diet and nutrition can exacerbate risks to other physiological systems and crew health. For example, many of the diseases of concern as related to space exploration are nutritionally modifiable on Earth, including cancer, cardiovascular disease, osteoporosis, sarcopenia, and cataracts.
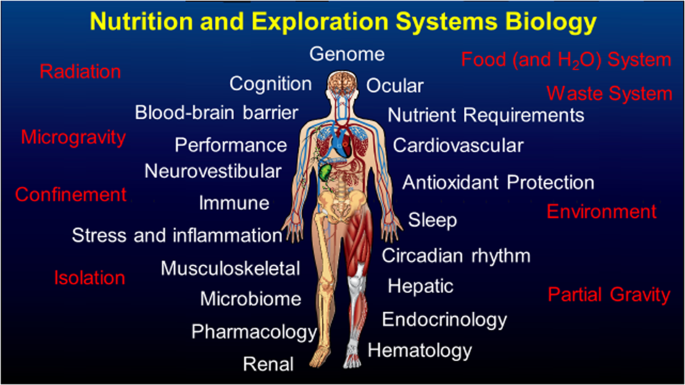
Many of the physiological systems and performance characteristics that are touched by nutrition are shown in white text, while the unique elements of spacecraft and space exploration are shown in red text.
The NASA Nutritional Biochemistry Laboratory approaches astronaut health with both operational and research efforts. These efforts aim to keep current crews healthy while working to understand and define optimal nutrition for future crews, to maximize performance and overall health while minimizing damaging effects of spaceflight exposure.
A Clinical Nutrition Assessment is conducted for ISS astronauts dating back to ISS Expedition 1 67 , 68 , which includes pre- and post-flight biochemical analyses conducted on blood and urine samples, along with in-flight monitoring of dietary intake and body mass. The biochemical assessments include a wide swath of nutritional indicators such as vitamins, minerals, proteins, hematology, bone markers, antioxidant markers, general chemistry, and renal stone risk. These data are reported to the flight surgeon soon after collection for use in the clinical care of the astronaut. Initial findings from the Clinical Nutritional Assessment protocol identified evidence of vitamin D deficiency, altered folate status, loss of body mass, increased kidney stone risk, and more 69 , 70 . These initial findings led to several research efforts (described below), including the Nutritional Status Assessment flight project, and research in the Antarctic on vitamin D supplementation 71 , 72 .
In addition to in-flight dietary intake monitoring, research to understand the impact and involvement of nutrition with other spaceflight risks such as bone loss and visual impairments, and interaction with exercise and spacecraft environment, are performed by the Nutrition Team using both flight and ground-analog research efforts. Tracking body mass is a very basic but nonetheless indispensable element of crew health 73 . Loss of body mass during spaceflight and in ground analogs of spaceflight is associated with exacerbated bone and muscle loss, cardiovascular degradation, increased oxidative stress, and more 70 , 73 , 74 . Historically, it was often assumed that some degree of body mass loss was to be expected, and that this was a typical part of adaptation to microgravity. Fluid loss is often assumed to be a key factor, but research has documented this to be a relatively small contributor, of approximately 1% of weight loss being fluid 74 , 75 . While on average, crewmembers on ISS missions have lost body mass over the course of flight, not all do 74 . Importantly, those that did not lose body mass managed to maintain bone mineral density (discussed below) 76 .
Bone loss has long been a concern for space travelers 77 , 78 , 79 , 80 , 81 . It has been shown that an increase in bone resorption was the likely culprit and that bone formation was largely unchanged in microgravity or ground analogs 77 , 78 , 79 . The search for a means to counteract this bone loss, and this hyper-resorptive state specifically, has been extensive. The potential for nutrition to mitigate this bone loss was identified early but studies of increasing intakes of calcium, or fluoride, or phosphate, were unsuccessful 74 , 77 , 79 , 82 , 83 , 84 .
Exercise provides a multisystem countermeasure, and heavy resistive exercise specifically provides for loading of bone to help mitigate weightlessness-induced bone loss.
In evaluating the data from astronauts using the first “interim” resistive exercise device (iRED) on ISS compared to a later, “advanced” resistive exercise device (ARED) (Fig. 12 ), it was quickly realized that exercise was not the only difference in these two groups of astronauts. ARED crews had better dietary intakes (as evidenced by maintenance of body mass) and better vitamin D status as a result of increased dose of supplementation and awareness of the importance of these supplements starting in 2006 76 . Bone mineral density was protected in these astronauts 76 , proving that diet and exercise are a powerful countermeasure combination. Follow-on evaluations showed similar results and further that the effects of microgravity exposure on bone health in men and women were similar 85 despite differences in pre-flight bone mass.
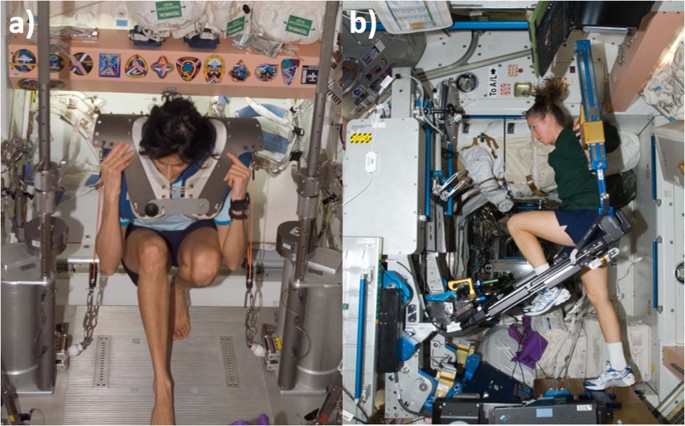
Sunita Williams exercising on the iRED ( a ), and on a later mission, Sandy Magnus exercises on the much improved ARED device ( b ). Images courtesy of NASA.
From a purely nutrition perspective, ISS and associated ground analog research has identified several specific dietary effects on bone health. Fish intake, likely secondary to omega-3 fatty acid intake, is beneficial for bone health 86 . Conversely, high intakes of dietary protein 87 , 88 , iron 89 and sodium 90 are detrimental to bone. The mechanism of the effect of protein and sodium on bone are likely similar, with both contributing to the acidogenic potential of the diet, leading to bone dissolution 91 , 92 . This effect was recently documented in a diet and bone health study on ISS, where the acidogenic potential of the diet correlated with post-flight bone losses 93 . The data from terrestrial research, along with the more limited spaceflight research, clearly identifies nutrition as important in maintenance of bone health and in the mitigation of bone loss. While initial evaluations of dietary quality and health are underway at NASA, much work remains to document the full potential of nutrition to mitigate bone loss and other disease processes in space travelers.
Another health risk with nutrition underpinnings is SANS, which was described earlier. When this issue first arose, an examination of data from the aforementioned ISS Nutrition project was conducted. This analysis revealed that affected crewmembers had significantly higher circulating concentrations of homocysteine and other one-carbon pathway metabolites when compared to non-cases and that these differences existed before flight 53 . Many potential confounding factors were ruled out, including: sex, kidney function, vitamin status, and coffee consumption, among others. After identifying differences in one-carbon biochemistry, the next logical step was to examine the genetics—single-nucleotide polymorphisms (SNPs)—involved in this pathway as possible causes of the biochemical differences, but perhaps also their association with the astronaut ocular pathologies. An initial study examined a small set of SNPs—five to be exact—and when the data were statistically modeled, it was found that B-vitamin status and genetics were significant predictors of many of the observed ophthalmic outcomes in astronauts 94 . Interestingly, the same SNPs identified in astronauts to be associated with ophthalmic changes after flight were associated with greater changes in total retina thickness after a strict head-down tilt with 0.5% CO 2 bed rest study 54 . A follow-on study is underway to evaluate a much broader look at one-carbon pathway and associated SNPs, potentially to help better characterize this relationship.
A hypothesis was developed to plausibly link these genetics and biochemical differences with these ophthalmic outcomes, as there is no existing literature regarding such a relationship. This multi-hit hypothesis posits that one-carbon pathway genetics is an indispensable factor, and that the combination with one or more other factors (e.g., fluid shifts, carbon dioxide, radiation, endocrine effects) lead to these pathologies. This has been detailed in a hypothesis paper 95 and in a recent review 96 . In brief, the hypothesis is that genetics and B-vitamin status contribute to endothelial dysfunction, as folate (and other B-vitamins) play critical roles in nitric oxide synthesis and endothelial function. A disruption in nitric oxide synthesis can also lead to an activation of matrix metalloproteinase activation, increasing the turnover and breakdown of structural elements of the sclera, altering retinal elasticity and increasing susceptibility to fluid shifts to induce ophthalmic pathologies like optic disc edema and choroidal folds 54 . This is likely exacerbated cerebrally due to limitations of transport of B-vitamins across the blood-brain barrier. In or around the orbit, endothelial dysfunction, oxidative stress, and potentially individual anatomical differences contribute to leaky blood vessels, and subsequent edema. This can impinge on cerebrospinal fluid drainage from the head, increasing those fluid pressures, which can impinge upon the optic nerve and eye itself, yielding the aforementioned ophthalmic pathologies. These are hypotheses proposed as starting points for further research. Given the irrefutable biochemical and genetic findings to date, this research should be a high priority to either prove or dismiss these as contributing factors in SANS to mitigate that “red” risk.
Another intriguing element from this research is that there is a clinical population that has many of the same characteristics of affected astronauts (or characteristics that they are purported to have), and that is women with polycystic ovary syndrome (PCOS) 95 , 96 . Women with PCOS have higher circulating homocysteine concentrations (as do their siblings and fathers), and also have cardiovascular pathology, including endothelial dysfunction. Studies are underway between NASA and physicians at the Mayo Clinic in Minnesota to evaluate this further. If validated, women with PCOS might represent an analog population for astronaut ocular issues, and research to counteract this could benefit both populations 87 . This research may lead to the identification of one-carbon pathway genetic influences on cardiovascular function in astronauts (and women with PCOS). This information will not be used in any sort of selection process, for several reasons, but as a means to identify countermeasures. Given the effects are intertwined with vitamin status, and likely represent higher individual vitamin requirements, targeted B-vitamin supplementation is the most obvious, and lowest risk, countermeasure that needs to be tested. There is tremendous potential for nutrition research to solve one of the key risks to human health on space exploration missions.
To summarize, nutrition is a cross-cutting field that has influence on virtually every system in the body. While we need to understand nutrition to avoid frank deficiencies, we need to understand how optimizing nutrition might also help mitigate other spaceflight-induced human health risks. Examples of this are myriad, ranging from effects of dietary intake on cognition, performance, and morale, inadequate intake on cardiovascular performance, excess nutrient intakes, leading to excess storage and increased oxidative stress, nutrient insufficiencies, leading to bone loss, insufficient fruit and vegetable intake on bone health, radiation protection, and cardiovascular health, to name a just few. Throughout history, nutrition has served, or failed, many a journey to explore. We need to dare to use and expand our twenty-first century knowledge of nutrition, uniting medical and scientific teams, to enable future exploration beyond LEO, while simultaneously benefitting humanity.
The NASA Human Research Program is focused on developing the tools and technologies needed to control the high priority “red” risks to an acceptable level—a great challenge as the risks do not exist in the vacuum of space as standalone entities. They are inherently interconnected and represent the intersection points where the five hazards of spaceflight overlap, and nature meets nurture. This is the space exposome: the total sum of spaceflight and lifetime exposures and how they relate to individual genetics and determine the whole-body outcome. The space exposome will be an important unifying concept as the hazards and risks of spaceflight are evaluated in a systems biology framework to fully uncover the emergent effects of the extraterrestrial experience on the human body. This framework will provide a path forward for mitigating detrimental health and performance outcomes that may stand in the way of successful, long-duration space travel, especially as NASA plans for a return to the Moon, to stay, and beyond to Mars.
Wild, C. P. The exposome: from concept to utility. Int. J. Epidemiol. 41 , 24–32 (2012).
Article PubMed Google Scholar
Crucian, B. E. et al. Immune system dysregulation during spaceflight: potential countermeasures for deep space exploration missions. Front. Immunol. 9 , 1–21 (2018).
Article CAS Google Scholar
Cassell, A. M. Forward to the Moon: NASA’s Strategic Plan for Human Exploration (NASA Ames Research Center ARC-E-DAA-TN73512, 2019).
Romero, E. & Francisco, D. The NASA human system risk mitigation process for space exploration. Acta Astronaut. 175 , 606–615 (2020).
Article Google Scholar
Simonsen, L. C., Slaba, T. C., Guida, P. & Rusek, A. NASA’s first ground-based Galactic Cosmic Ray Simulator: enabling a new era in space radiobiology research. PLOS Biol. 18 , e3000669 (2020).
Article CAS PubMed Central PubMed Google Scholar
Norbury, J. W. et al. Galactic cosmic ray simulation at the NASA Space Radiation Laboratory. Life Sci. Space Res. (Amst.) 8 , 38–51 (2016).
NCRP. Report No. 183—Radiation Exposure in Space and the Potential for Central Nervous System Effects: Phase II . (National Council on Radiation Protection, 2019).
Slaba, T., Mertens, C. J. & Blattnig, S. R. Radiation Shielding Optimization on Mars . (NASA/TP–2013-217983, 2013).
Simonsen, L. C. & Nealy, J. E. Radiation Protection for Human Missions to the Moon and Mars . (NASA-TP-3079, 1991).
Durante, M. & Cucinotta, F. A. Heavy ion carcinogenesis and human space exploration. Nat. Rev. Cancer 8 , 465–472 (2008).
Article CAS PubMed Google Scholar
Li, M. et al. Health risks of space exploration: targeted and nontargeted oxidative injury by high-charge and high-energy particles. Antioxid. Redox Signal. 20 , 1501–1523 (2014).
Sridharan, D. M., Chappell, L. J., Whalen, M. K., Cucinotta, F. A. & Pluth, J. M. Defining the biological effectiveness of components of High-LET track structure. Radiat. Res. 184 , 105–119 (2015).
Rose, Li,Y. et al. Mutational signatures in tumours induced by high and low energy radiation in Trp53 deficient mice. Nat. Commun. 11 , 394 (2020).
Datta, K., Suman, S., Kallakury, B. V. S. & Fornace, A. J. Exposure to heavy ion radiation induces persistent oxidative stress in mouse intestine. PLoS ONE 7 , e42224 (2012).
Kumar, S., Suman, S., Fornace, A. J. & Datta, K. Space radiation triggers persistent stress response, increases senescent signaling, and decreases cell migration in mouse intestine. Proc. Natl Acad. Sci. USA 115 , E9832–E9841 (2018).
Article CAS PubMed PubMed Central Google Scholar
National Academies of Sciences, Engineering, and Medicine of Sciences. A Midterm Assessment of Implementation of the Decadal Survey on Life and Physical Sciences Research at NASA . (The National Academies Press, 2018).
La Tessa, C., Sivertz, M., Chiang, I.-H., Lowenstein, D. & Rusek, A. Overview of the NASA space radiation laboratory. Life Sci. Space Res. (Amst.) 11 , 18–23 (2016).
Schimmerling, W. Genesis of the NASA space radiation laboratory. Life Sci. Space Res. (Amst.) 9 , 2–11 (2016).
Ozasa, K., Cullings, H. M., Ohishi, W., Hida, A. & Grant, E. J. Epidemiological studies of atomic bomb radiation at the radiation effects research foundation. Int. J. Radiat. Biol. 95 , 879–891 (2019).
Boice, J. D. et al. The past informs the future: an overview of the million worker study and the mallinckrodt chemical works cohort. Health Phys. 114 , 381–385 (2018).
Richardson, D. B. et al. Site-specific solid cancer mortality after exposure to ionizing radiation. Epidemiology 29 , 31–40 (2018).
Article PubMed Central PubMed Google Scholar
Kamiya, K. et al. Long-term effects of radiation exposure on health. Lancet 386 , 469–478 (2015).
Cucinotta, F. A., Kim, M.-H. Y. & Chappell, L. J. Space Radiation Cancer Risk Projections and Uncertainties–2012 . Vol. 186 (2013).
Hanahan, D. & Weinberg, R. A. Hallmarks of cancer: the next generation. Cell 144 , 646–674 (2011).
Barcellos-Hoff, M. H. et al. Concepts and challenges in cancer risk prediction for the space radiation environment. Life Sci. Space Res. (Amst.) 6 , 92–103 (2015).
Barcellos-Hoff, M. H. & Mao, J.-H. HZE radiation non-targeted effects on the microenvironment that mediate mammary carcinogenesis. Front. Oncol. 6 , 57 (2016).
Sridharan, D. M. et al. Understanding cancer development processes after HZE-particle exposure: roles of ROS, DNA damage repair and inflammation. Radiat. Res. 183 , 1–26 (2015).
Sylvester, C. B., Abe, J.-I., Patel, Z. S. & Grande-Allen, K. J. Radiation-induced cardiovascular disease: mechanisms and importance of linear energy transfer. Front. Cardiovasc. Med. 5 , 5 (2018).
Article PubMed Central CAS PubMed Google Scholar
Darby, S. C. et al. Risk of ischemic heart disease in women after radiotherapy for breast cancer. N. Engl. J. Med. 368 , 987–998 (2013).
Little, M. P. et al. Systematic review and meta-analysis of circulatory disease from exposure to low-level ionizing radiation and estimates of potential population mortality risks. Environ. Health Perspect. 120 , 1503–1511 (2012).
ICRP. ICRP statement on tissue reactions and early and late effects of radiation in normal tissues and organs-threshold doses for tissue reactions in a radiation protection context. ICRP Publication 118. Ann. ICRP 41 , 1–322 (2012).
Google Scholar
Cucinotta, F. A., Kim, M.-H. Y., Chappell, L. J. & Huff, J. L. How safe is safe enough? Radiation risk for a human mission to Mars. PLoS ONE 8 , e74988 (2013).
Hughson, R. L., Helm, A. & Durante, M. Heart in space: effect of the extraterrestrial environment on the cardiovascular system. Nat. Rev. Cardiol. 15 , 167–180 (2018).
Kreuzer, M. et al. Low-dose ionising radiation and cardiovascular diseases-Strategies for molecular epidemiological studies in Europe. Mutat. Res. Rev. Mutat. Res. 764 , 90–100 (2015).
Elgart, S. R. et al. Radiation exposure and mortality from cardiovascular disease and cancer in early NASA astronauts. Sci. Rep. 8 , 8480 (2018).
Boerma, M. An introduction to space radiation and its effects on the cardiovascular system. THREE , 1–12 (2016).
Camacho, P., Fan, H., Liu, Z. & He, J.-Q. Small mammalian animal models of heart disease. Am. J. Cardiovasc. Dis. 6 , 70–80 (2016).
CAS PubMed Central PubMed Google Scholar
Ko, K. A. et al. Developing a reliable mouse model for cancer therapy-induced cardiovascular toxicity in cancer patients and survivors. Front. Cardiovasc. Med. 5 , 1–13 (2018).
Parihar, V. K. et al. Cosmic radiation exposure and persistent cognitive dysfunction. Sci. Rep. 6 , 34774 (2016).
Parihar, V. K. et al. Persistent nature of alterations in cognition and neuronal circuit excitability after exposure to simulated cosmic radiation in mice. Exp. Neurol. 305 , 44–55 (2018).
Hinkle, J. J., Olschowka, J. A., Love, T. M., Williams, J. P. & O’Banion, M. K. Cranial irradiation mediated spine loss is sex-specific and complement receptor-3 dependent in male mice. Sci. Rep. 9 , 18899 (2019).
Liu, B. et al. Space-like 56Fe irradiation manifests mild, early sex-specific behavioral and neuropathological changes in wildtype and Alzheimer’s-like transgenic mice. Sci. Rep. 9 , 12118 (2019).
Raber, J. et al. Combined Effects of Three High-Energy Charged Particle Beams Important for Space Flight on Brain, Behavioral and Cognitive Endpoints in B6D2F1 Female and Male Mice. Front. Physiol. 10 , 1–15 (2019).
Rosi, S. The final frontier: transient microglia reduction after cosmic radiation exposure mitigates cognitive impairments and modulates phagocytic activity. Brain Circ. 4 , 109–113 (2018).
Kiffer, F., Boerma, M. & Allen, A. Behavioral effects of space radiation: a comprehensive review of animal studies. Life Sci. Space Res. (Amst.) 21 , 1–21 (2019).
Whoolery, C. W. et al. Multi-domain cognitive assessment of male mice shows space radiation is not harmful to high-level cognition and actually improves pattern separation. Sci. Rep. 10 , 2737 (2020).
Mader, T. H. et al. Optic disc edema, globe flattening, choroidal folds, and hyperopic shifts observed in astronauts after long-duration space flight. Ophthalmology 118 , 2058–2069 (2011).
Macias, B. et al. Anterior and posterior ocular structures change during long-duration spaceflight and one year after landing. Invest. Ophthalmol. Vis. Sci. 59 , 722 (2018).
Lee, A. G. et al. Spaceflight associated neuro-ocular syndrome (SANS) and the neuro-ophthalmologic effects of microgravity: a review and an update. NPJ Microgravity 6 , 1–10 (2020).
Brunstetter, T. J. & Tarver, W. J. Spaceflight Associated Neuro-ocular Syndrome (SANS): Current Clinical Insight & Questions of Interest (Translational Research Institute for Space Health - The Red Risk School, 2018).
Lee, J. K. et al. Head down tilt bed rest plus elevated CO 2 as a spaceflight analog: effects on cognitive and sensorimotor performance. Front. Hum. Neurosci. 13 , 1–11 (2019).
Lee, A. G., Mader, T. H., Gibson, C. R. & Tarver, W. Space flight-associated neuro-ocular syndrome. JAMA Ophthalmol. 135 , 992–994 (2017).
Zwart, S. R. et al. Vision changes after spaceflight are related to alterations in folate- and vitamin B-12-dependent one-carbon metabolism. J. Nutr. 142 , 427–431 (2012).
Zwart, S. R. et al. Association of genetics and B vitamin status with the magnitude of optic disc edema during 30-day strict head-down tilt bed rest. JAMA Ophthalmol. 137 , 1195–1200 (2019).
Patel, N., Pass, A., Mason, S., Gibson, C. R. & Otto, C. Optical coherence tomography analysis of the optic nerve head and surrounding structures in long-duration international space station astronauts. JAMA Ophthalmol. 136 , 193–200 (2018).
Roberts, D. R. et al. Effects of spaceflight on astronaut brain structure as indicated on MRI. N. Engl. J. Med. 377 , 1746–1753 (2017).
Van Ombergen, A. et al. Brain ventricular volume changes induced by long-duration spaceflight. Proc. Natl Acad. Sci. USA 116 , 10531–10536 (2019).
Alperin, N., Bagci, A. M. & Lee, S. H. Spaceflight-induced changes in white matter hyperintensity burden in astronauts. Neurology 89 , 2187–2191 (2017).
Kramer, L. A. et al. Intracranial effects of microgravity: a prospective longitudinal MRI Study. Radiology 295 , 640–648 (2020).
Dinges, D. F. et al. PVT on ISS: reaction self-test (RST) from 6-month missions. (Human Research Program Investigators’ Workshop, 2017).
Stuster, J. Behavioral Issues Associated with Isolation and Confinement: Review and Analysis of Astronaut Journals (NASA Human Research Program NASA/TM-2010-216130, 2016).
Basner, M. et al. Psychological and behavioral changes during confinement in a 520-day simulated interplanetary mission to mars. PLoS ONE 9 , e93298 (2014).
Barger, L. K. et al. Prevalence of sleep deficiency and use of hypnotic drugs in astronauts before, during, and after spaceflight: an observational study. Lancet Neurol. 13 , 904–912 (2014).
Greene, M. et al. ISS Habitability Data Collection and Preliminary Findings (Houston Human Factors and Ergonomic Society , 2018).
Lee, J. K. et al. Spaceflight-associated brain white matter microstructural changes and intracranial fluid redistribution. JAMA Neurol. 76 , 412–419 (2019).
Larson, L. et al. Team performance in space crews: Houston, we have a teamwork problem. Acta Astronaut. 161 , 108–114 (2019).
NASA Johnson Space Center. Nutritional Status Assessment for Extended-duration Space Flight . (NASA JSC Document #JSC-28566, Revision 1, 1999).
Smith, S. M. et al. Nutritional status assessment in semiclosed environments: ground-based and space flight studies in humans. J. Nutr. 131 , 2053–2061 (2001).
Smith, S. M., Zwart, S. R., Block, G., Rice, B. L. & Davis-Street, J. E. The nutritional status of astronauts is altered after long-term space flight aboard the International Space Station. J. Nutr. 135 , 437–443 (2005).
Smith, S. M., Zwart, S. R., Kloeris, V. & Heer, M. Nutritional Biochemistry of Space Flight . (Nova Science Publishers, 2009).
Smith, S. M., Gardner, K. K., Locke, J. & Zwart, S. R. Vitamin D supplementation during Antarctic winter. Am. J. Clin. Nutr. 89 , 1092–1098 (2009).
Zwart, S. R. et al. Response to vitamin D supplementation during Antarctic winter is related to BMI, and supplementation can mitigate Epstein-Barr Virus Reactivation. J. Nutr. 141 , 692–697 (2011).
Zwart, S. R. et al. Body mass changes during long-duration spaceflight. Aviat. Space Environ. Med. 85 , 897–904 (2014).
Smith, S. M., Zwart, S. R. & Heer, M. Human Adaptation to Spaceflight: the Role of Nutrition . (NASA Johnson Space Center #NP-2014-10-018-JSC, 2014).
Leach, C. S. et al. Regulation of body fluid compartments during short-term spaceflight. J. Appl. Physiol. 81 , 105–116 (1996).
Smith, S. M. et al. Benefits for bone from resistance exercise and nutrition in long-duration spaceflight: evidence from biochemistry and densitometry. J. Bone Miner. Res. 27 , 1896–1906 (2012).
Smith, S. M. et al. Fifty years of human space travel: implications for bone and calcium research. Annu. Rev. Nutr. 34 , 377–400 (2014).
Sibonga, J. D. et al. Adaptation of the skeletal system during long-duration spaceflight. Clin. Rev. Bone Miner. Metab. 5 , 249–261 (2008).
Smith, S. M., Heer, M. & Zwart, S. R. in Nutrition and bone health, 2nd edn . (eds. Holick, M. & Nieves, J.) 687–705 (Springer, 2015).
Sibonga, J. D., Spector, E. R., Johnston, S. L. & Tarver, W. J. Evaluating bone loss in ISS astronauts. Aerosp. Med. Hum. Perform. 86 (suppl. 1), 38–44 (2015).
LeBlanc, A. D., Spector, E. R., Evans, H. J. & Sibonga, J. D. Skeletal responses to space flight and the bed rest analog: a review. J. Musculoskelet. Neuronal Interact. 7 , 33–47 (2007).
CAS PubMed Google Scholar
Schneider, V. S. et al. The Prevention of Bone Mineral Changes Induced by Bed Rest: Modification by Static Compression Simulating Weight Bearing, Combined Supplementation of Oral Calcium and Phosphate, Calcitonin Injections, Oscillating Compression, the Oral Diphosphonate Disodium Etidronate, and Lower Body Negative Pressure (Final Report) . (NASA CR-141453, 1974).
Maheshwari, U. R. et al. Comparison of fluoride balances during ambulation and bed rest. Proc. West. Pharmacol. Soc. 24 , 151–153 (1981).
Baecker, N., Frings-Meuthen, P., Smith, S. M. & Heer, M. Short-term high dietary calcium intake during bedrest has no effect on markers of bone turnover in healthy men. Nutrition 26 , 522–527 (2010).
Smith, S. M. et al. Men and women in space: bone loss and kidney stone risk after long-duration spaceflight. J. Bone Miner. Res. 29 , 1639–1645 (2014).
Zwart, S. R., Pierson, D., Mehta, S., Gonda, S. & Smith, S. M. Capacity of omega-3 fatty acids or eicosapentaenoic acid to counteract weightlessness-induced bone loss by inhibiting NF-kappaB activation: from cells to bed rest to astronauts. J. Bone Miner. Res. 25 , 1049–1057 (2010).
Heer, M. et al. Effects of high-protein intake on bone turnover in long-term bed rest in women. Appl. Physiol. Nutr. Metab. 42 , 537–546 (2017).
Zwart, S. R. et al. Amino acid supplementation alters bone metabolism during simulated weightlessness. J. Appl. Physiol. 99 , 134–140 (2005).
Zwart, S. R., Morgan, J. L. & Smith, S. M. Iron status and its relations with oxidative damage and bone loss during long-duration space flight on the International Space Station. Am . J. Clin. Nutr. 98 , 217–223 (2013).
Frings-Meuthen, P. et al. High sodium chloride intake exacerbates immobilization-induced bone resorption and protein losses. J. Appl. Physiol. 111 , 537–542 (2011).
Frings-Meuthen, P., Baecker, N. & Heer, M. Low-grade metabolic acidosis may be the cause of sodium chloride-induced exaggerated bone resorption. J. Bone Miner. Res. 23 , 517–524 (2008).
Heer, M. et al. Increasing sodium intake from a previous low or high intake affects water, electrolyte and acid-base differently. Br. J. Nutr. 101 , 1286–1294 (2009).
Zwart, S. R. et al. Dietary acid load and bone turnover during long-duration spaceflight and bed rest. Am. J. Clin. Nutr. 107 , 834–844 (2018).
Zwart, S. R. et al. Genotype, B-vitamin status, and androgens affect spaceflight-induced ophthalmic changes. FASEB J. 30 , 141–148 (2016).
Zwart, S. R. et al. Astronaut ophthalmic syndrome. FASEB J. 31 , 3746–3756 (2017).
Smith, S. M. & Zwart, S. R. Spaceflight-related ocular changes: the potential role of genetics, and the potential of B vitamins as a countermeasure. Curr. Opin. Clin. Nutr. Metab. Care 21 , 481–488 (2018).
Cucinotta, F. A. & Durante, M. Cancer risk from exposure to galactic cosmic rays: implications for space exploration by human beings. Lancet Oncol. 7 , 431–435 (2006).
Download references
Acknowledgements
This review was supported in part by a grant to Dr. Patel from the Translational Research Institute for Space Health (TRISH) from the Baylor College of Medicine (The Red Risk School). It was also supported by funding through NASA Human Health and Performance Contract #NNJ15HK11B (Z.S.P., S.R.Z., J.L.H.) and NASA directly (T.J.B., W.J.T., A.M.W., S.M.S., J.L.H.).
Author information
Authors and affiliations.
KBR, Houston, TX, USA
Zarana S. Patel
NASA Lyndon B. Johnson Space Center, Houston, TX, USA
Zarana S. Patel, William J. Tarver, Alexandra M. Whitmire, Sara R. Zwart & Scott M. Smith
U.S. Navy, NASA Lyndon B. Johnson Space Center, Houston, TX, USA
Tyson J. Brunstetter
University of Texas Medical Branch at Galveston, Galveston, TX, USA
Sara R. Zwart
NASA Langley Research Center, Hampton, VA, USA
Janice L. Huff
You can also search for this author in PubMed Google Scholar
Contributions
Drs. Z.S.P. and J.L.H. compiled and edited the overall manuscript and drafted the radiation risk overviews. Drs. T.J.B. and W.J.T. drafted the SANS risk overview, Dr. A.M.W. drafted the behavioral health risks overview, and Drs. S.R.Z. and S.M.S. drafted the nutrition risk overview. Data are available upon request.
Corresponding author
Correspondence to Zarana S. Patel .
Ethics declarations
Competing interests.
All of the authors declare that they have no “competing interests” related to funding, person, or financial interest. Although the authors work directly (T.J.B., W.J.T., A.M.W., S.M.S., J.L.H.) as employees or indirectly as contractors (Z.S.P., S.R.Z.) for NASA, the views and opinions expressed here are those of the authors and do not necessarily reflect the views of NASA or the United States government.
Additional information
Publisher’s note Springer Nature remains neutral with regard to jurisdictional claims in published maps and institutional affiliations.
Rights and permissions
Open Access This article is licensed under a Creative Commons Attribution 4.0 International License, which permits use, sharing, adaptation, distribution and reproduction in any medium or format, as long as you give appropriate credit to the original author(s) and the source, provide a link to the Creative Commons license, and indicate if changes were made. The images or other third party material in this article are included in the article’s Creative Commons license, unless indicated otherwise in a credit line to the material. If material is not included in the article’s Creative Commons license and your intended use is not permitted by statutory regulation or exceeds the permitted use, you will need to obtain permission directly from the copyright holder. To view a copy of this license, visit http://creativecommons.org/licenses/by/4.0/ .
Reprints and permissions
About this article
Cite this article.
Patel, Z.S., Brunstetter, T.J., Tarver, W.J. et al. Red risks for a journey to the red planet: The highest priority human health risks for a mission to Mars. npj Microgravity 6 , 33 (2020). https://doi.org/10.1038/s41526-020-00124-6
Download citation
Received : 09 July 2020
Accepted : 30 September 2020
Published : 05 November 2020
DOI : https://doi.org/10.1038/s41526-020-00124-6
Share this article
Anyone you share the following link with will be able to read this content:
Sorry, a shareable link is not currently available for this article.
Provided by the Springer Nature SharedIt content-sharing initiative
This article is cited by
Physiological evidence of stress reduction during a summer antarctic expedition with a significant influence of previous experience and vigor.
- Lucie Ráčková
- Tomáš Pompa
- Julie Bienertová-Vašků
Scientific Reports (2024)
Dynamic changes in perivascular space morphology predict signs of spaceflight-associated neuro-ocular syndrome in bed rest
- Sutton B. Richmond
- Rachael D. Seidler
- Juan Piantino
npj Microgravity (2024)
Muscle stiffness indicating mission crew health in space
- Britt Schoenrock
- Paul E. Muckelt
- Dieter Blottner
A potential compensatory mechanism for spaceflight associated neuro-ocular changes from microgravity: current understanding and future directions
- Benjamin Soares
- Andrew G. Lee
SANS-CNN: An automated machine learning technique for spaceflight associated neuro-ocular syndrome with astronaut imaging data
- Sharif Amit Kamran
- Khondker Fariha Hossain
- Alireza Tavakkoli
Quick links
- Explore articles by subject
- Guide to authors
- Editorial policies
Sign up for the Nature Briefing: Cancer newsletter — what matters in cancer research, free to your inbox weekly.

Mars Travel Time: Estimating the Journey to the Red Planet
- February 23rd, 2024
- No Comments
Table Of Contents
Venturing to Mars is a challenging and lengthy endeavour, requiring sophisticated technology, meticulous planning, and a deep understanding of the physics involved in long-distance space travel. On this journey spanning roughly 225 million kilometres, a spacecraft endures a multitude of factors, from the gravitational pulls of celestial bodies to the alignment of Earth and Mars. Timing is crucial, as the optimal travel windows, known as launch windows, occur only every 26 months when the two planets are favourably aligned.
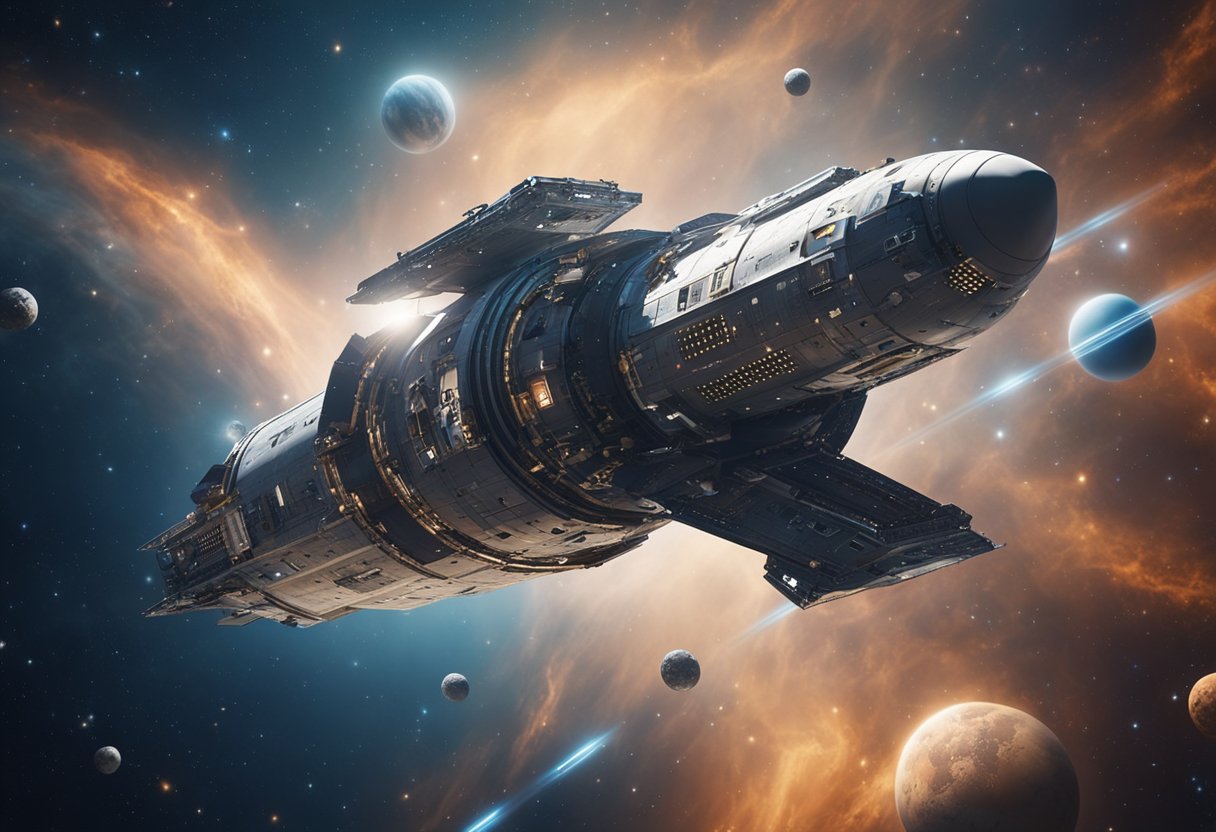
Our calculations and past missions suggest that a one-way journey to the Red Planet can vary significantly in duration, usually taking between six to nine months. This variability is dependent on the speed of the spacecraft, the specific trajectory taken, and the positions of the planets at the time of launch. The spacecraft’s propulsion technology and energy sources play a vital role in the speed and efficiency of the trip, with nuclear fission and solar power being among the options considered for ensuring a steady energy supply to the spacecraft navigating this interplanetary voyage.
Key Takeaways
- Travel to Mars requires careful timing and can take between six to nine months depending on planetary alignment.
- Propulsion technology and energy sources are fundamental for the spacecraft’s speed and journey efficiency.
- Optimising travel windows and trajectories is essential to successfully reaching the Red Planet.
Historical Missions to Mars
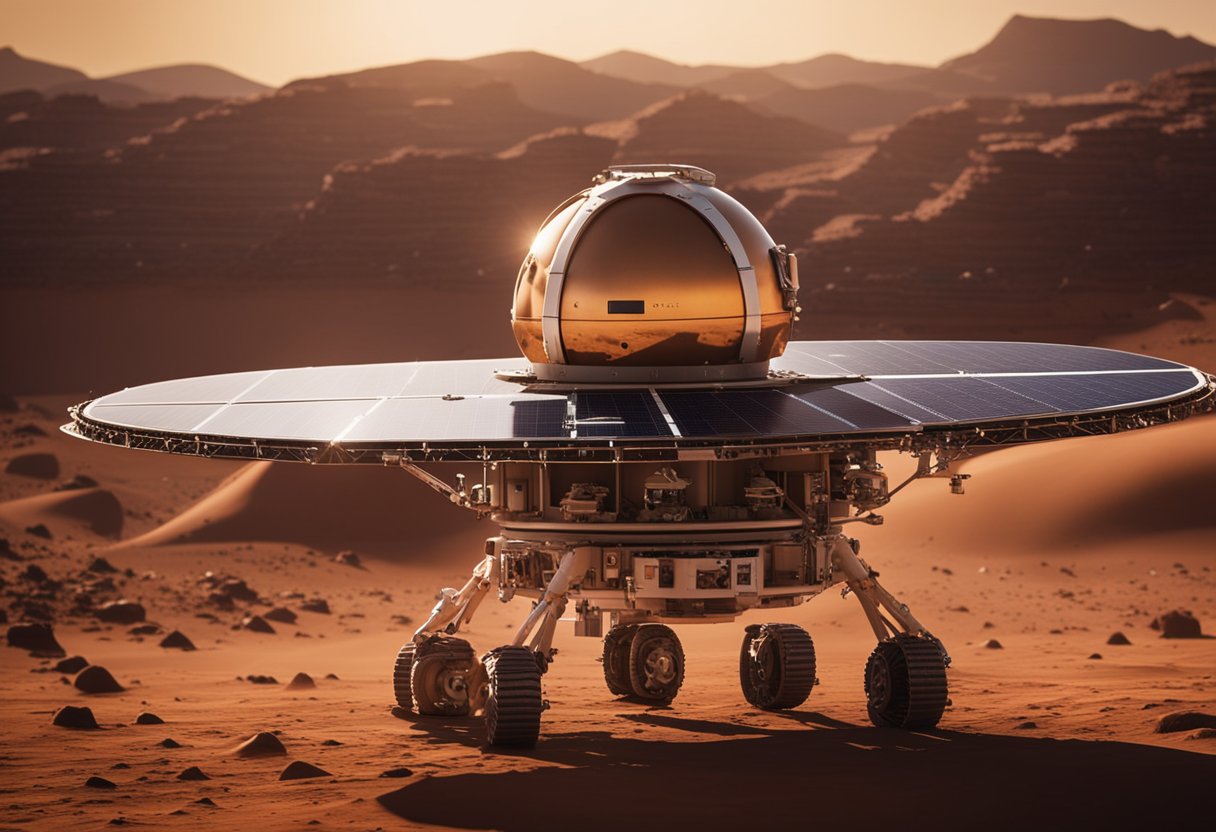
As we explore the chronicles of Martian exploration, we recall the achievements and aspirations that have propelled us from early flybys to the quest for signs of ancient life.
Early Exploration
Our journey to Mars commenced with the trailblazing Mariner 4 , which in 1965 furnished us with the first close-up photographs of the Martian surface. Following this, Mariner 7 and Mariner 9 advanced our understanding, with Mariner 9 becoming the first spacecraft to orbit another planet in 1971. This achievement marked the transition from mere flyby missions to extended observational studies, allowing our engineers to scrutinise Mars with new technologies and more sophisticated instruments.
Recent and Upcoming Missions
In the vanguard of these missions, the Viking 1 and Viking 2 landers in 1975 set a precedent for future missions by searching for signs of life and returning detailed insights of the Martian soil and atmosphere. We advanced further with the Mars Reconnaissance Orbiter , which has been surveying the Red Planet since 2006, significantly enhancing our topographical data.
The indomitable Perseverance rover , with a mission to seek signs of ancient life, represents our latest feat, setting its wheels upon Martian soil in 2021. These instruments, alongside a host of landers, orbiters, and rovers, have paved the way for the technologies that could one day support human and robotic exploration alike.
Future missions are poised to unravel more of Mars’s secrets, and with budding enterprises like SpaceVoyageVentures.com , the notion of space tourism is nearing the cusp of reality—a testament to human ingenuity and the relentless pursuit of knowledge.
Physics of Mars Travel
Travelling to Mars involves complex physics, where every aspect from speed to energy becomes critical for a successful mission. We’ll unpack the fundamental properties involved in such an interplanetary journey.
Orbital Mechanics
To understand our trajectory to Mars, we must first grasp the principles of orbital mechanics . These laws dictate how we manoeuvre a spacecraft from Earth’s orbit to intersect Mars’. A Hohmann transfer orbit is often employed; this is an energy-efficient method of travelling between two orbiting bodies. The Hohmann transfer involves two key points in its orbit, the periapsis , where the spacecraft is closest to Earth, and the apoapsis , where it extends out to touch Mars’ orbit. To execute this, we need precise calculations of the velocity required to launch and enter into this transfer orbit.
Transfer Windows
Transfer windows are specific times when Earth and Mars align favourably, making the trip feasible. This alignment occurs approximately every 26 months. To take advantage of this, we must calculate when Mars and Earth will be in positions that minimise the energy required for travel. This involves considering the gravitational pull of both planets and the Sun, as they influence the spacecraft’s journey through the Solar System . The speed of a Mars-bound spacecraft is approximately 24,600 mph (~39,600 kph) after leaving Earth’s orbit, with the entire trip spanning a distance that can exceed 300 million miles (480 million kilometres), which is well over an astronomical unit , the standard measure of distance within our Solar System. The speed of light is not a constraint in current propulsion methods, but advancements in technology, such as the theoretical photonic propulsion, could revolutionise these parameters. During close approaches , when Mars and Earth are nearest to each other, the required travel distance is decreased, making it the most optimal time for launch.
Mars Orbit and Environment
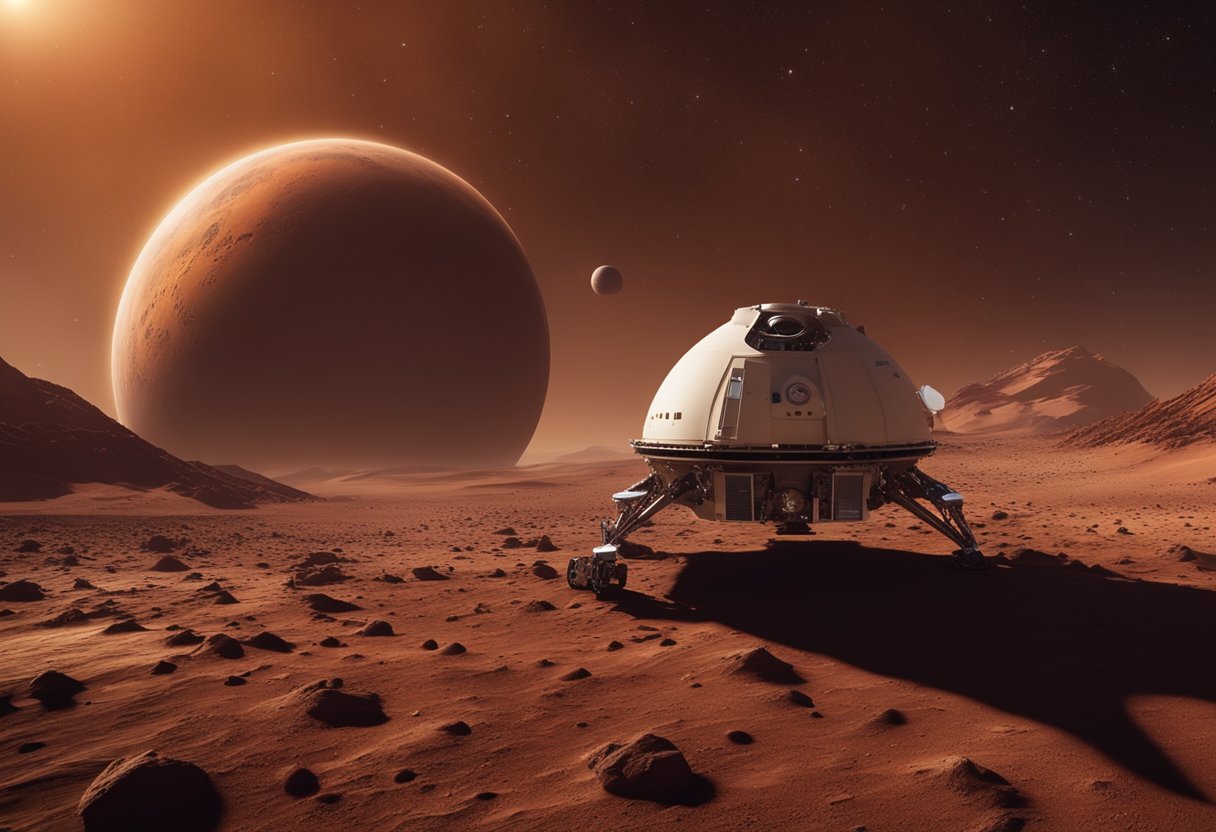
As we examine the journey to Mars, it’s crucial to understand its orbit around the Sun and the environmental conditions present. Mars completes an orbit every 687 Earth days, indicating a longer Martian year, and has seasons due to its 25.19-degree axial tilt.
Atmospheric Conditions
Mars’ atmosphere is vastly different from Earth’s, being thin and composed primarily of carbon dioxide . Average temperatures on the Martian surface can vary greatly, with a maximum of about 20°C at the equator during midday, to a minimum of below -125°C near the poles during winter. Despite its thin atmosphere, Mars experiences weather , including massive dust storms that can engulf the entire planet and significantly impact temperatures and visibility.
Surface and Geological Features
The Martian landscape is both striking and diverse, characterised by a rocky surface with the largest volcano in the solar system, Olympus Mons, and the Valles Marineris — an expansive canyon system. Evidence of water in Mars’ past is written into the surface features, including river valleys and lakebeds. Mars has two small moons , named Phobos and Deimos , which are thought to be captured asteroids. Despite being only about half the size of Earth with a diameter of 6,779 km, Mars possesses a gravity about 38% of Earth’s which, combined with its solid core , contributes to the overall geology of the planet.
Spacecraft and Technologies
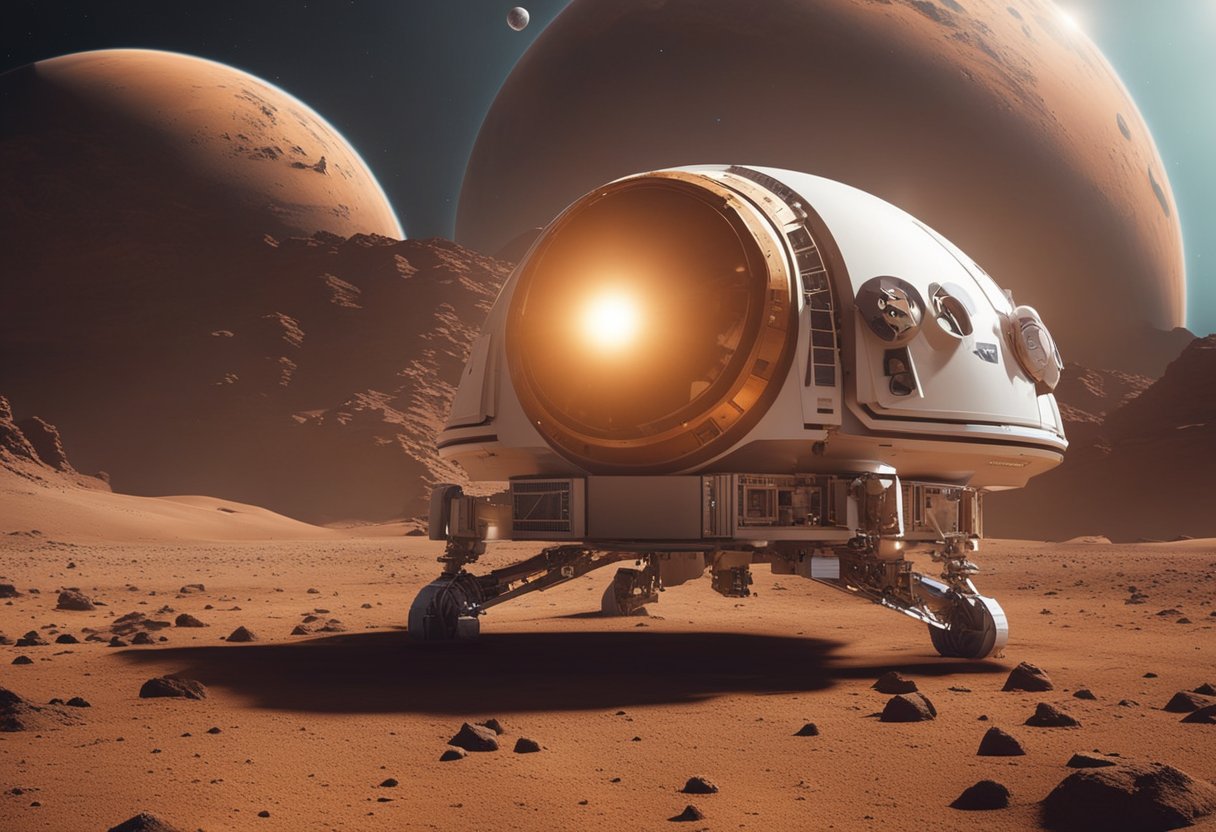
In preparations for Mars travel, we focus heavily on the development and optimisation of spacecraft and supporting technologies. These advancements are imperative to ensure that our journey to the Red Planet is feasible, efficient, and safe for all involved.
Propulsion Systems
Propulsion technologies are at the heart of interplanetary travel . Our engineers are working on more powerful propulsion systems using advanced propellants , which are crucial for reducing travel time to Mars. These systems are designed to provide the significant energy required to escape Earth’s gravity and then navigate the spacecraft the approximately 140 million miles to Mars. By improving fuel efficiency and engine performance, we not only aim to shorten the trip but also enhance the safety and reliability of the mission.
Life Support Systems
Ensuring the sustainment of life in the vast expanse of space is no small feat. Our life support systems are engineered to meticulously recycle water and oxygen , creating a habitable environment for astronauts on long-duration spaceflights. Innovative technologies are being developed to manage resources more effectively, including high-efficiency systems that convert waste into usable energy and nutrients. Maintaining a stable life support system is essential for the success of our Mars missions and ensures the well-being of the crew.
Risks and Challenges of Mars Travel
As we prepare for the monumental task of sending humans to Mars, it is crucial to understand the significant risks and challenges that such a journey presents. Our technological advancements have provided us with a path, but the sheer distance and unknowns of space make it an endeavour fraught with hazards that we must meticulously navigate.
Radiation Exposure
One of the most critical hazards of interplanetary travel is the high level of radiation exposure. Unlike Earth, which has a protective magnetic field and atmosphere, space offers no such shield. During the journey to Mars, astronauts will be exposed to galactic cosmic rays and solar particle events that can lead to severe health risks, including cancer and acute radiation sickness.
- Cosmic Radiation: Consists of high-energy particles from outside our solar system.
- Solar Flares: Erupt from the sun, releasing particles and radiation.
Our current technology is being developed to mitigate these risks with shielding and medical countermeasures. However, this remains a primary concern for the safety of astronauts during their expedition.
Psychological Effects
The psychological impact of a Mars mission poses another set of challenges. Dealing with isolation, confinement, and distance from Earth for extended periods—potentially up to three years—can have significant effects on the mental health of the crew.
- Isolation: Limited contact with family and friends, dependency on delayed communication with Earth.
- Confined Spaces: Spending a long time in small, enclosed living quarters.
These factors necessitate careful psychological screening and the development of robust support systems to ensure the well-being of astronauts. Measures include comprehensive training and the simulation of long-duration missions here on Earth.
Our understanding of these risks is continuously evolving as we gather more data from ongoing research and simulations, bringing the dream of human exploration on Mars closer to becoming a reality. Our journey to the Red Planet will be one of the most significant leaps for human life —a remarkable testament to our resilience and explorative spirit.
Life on Mars
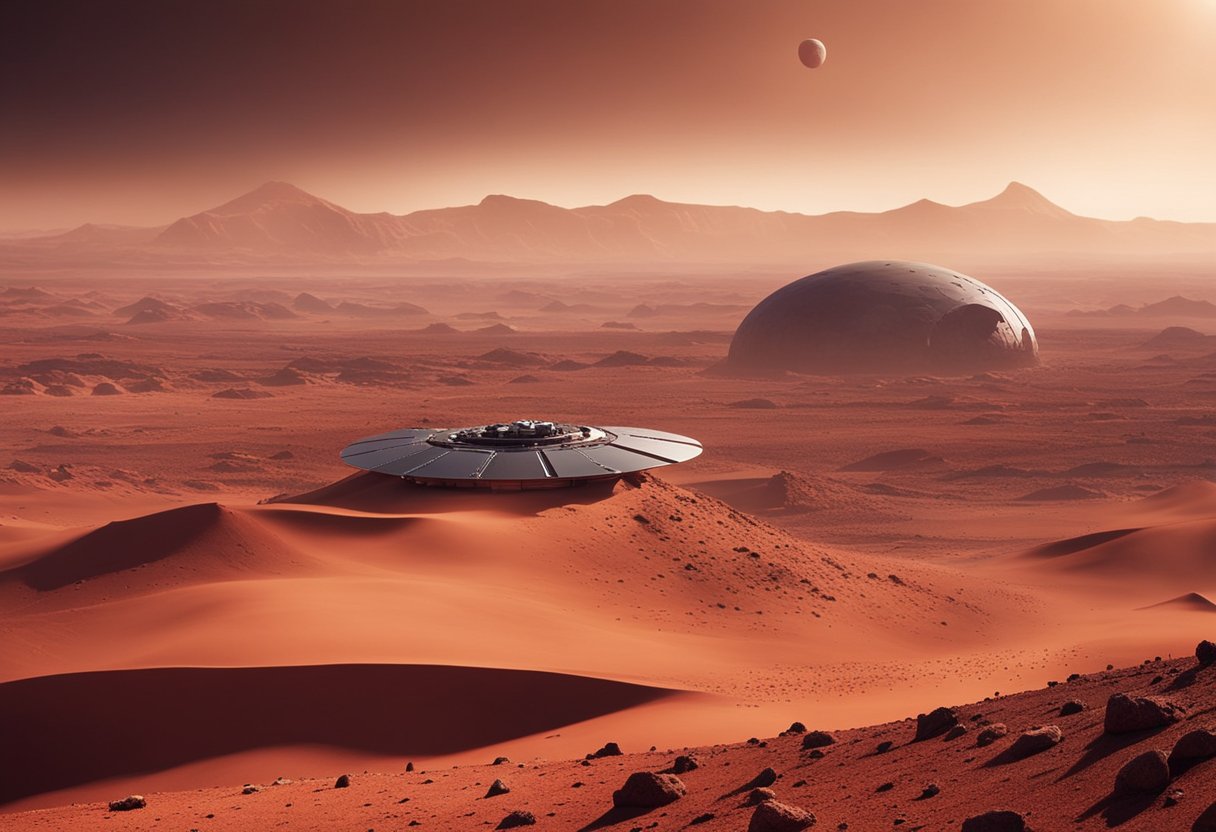
Exploration of Mars presents a tantalising glimpse into the possibility of life beyond our Earth, specifically targeting evidence of ancient life and assessing the planet’s suitability for future human habitation.
Search for Past Life
We understand that Mars holds significant scientific interest due to indications that it once harboured liquid water , a crucial ingredient for life as we know it. Robotic missions, such as NASA’s Perseverance rover, are equipped to scrutinise sediments for biomarkers of ancient life. These missions aim to uncover signs that, billion of years ago when water was abundant, Mars might have been home to microbial life.
Conditions for Human Habitation
As we anticipate human missions to Mars, possibly in the 2030s , understanding current conditions on Mars is essential. The atmosphere is thin and composed of 96% carbon dioxide , with extremely low levels of oxygen . Temperatures can fluctuate greatly, from a high of about 20°C to lows of -130°C. Mars’s gravity is approximately 38% of Earth’s, raising questions about its effects on human health. The development of life support systems that can effectively produce water and oxygen is critical for prolonged human presence on the Red Planet. Our investigations aim to figure out how to safely provide for human needs in this harsh environment, a topic documented by websites such as SpaceVoyageVentures.com .
Future Mars Missions
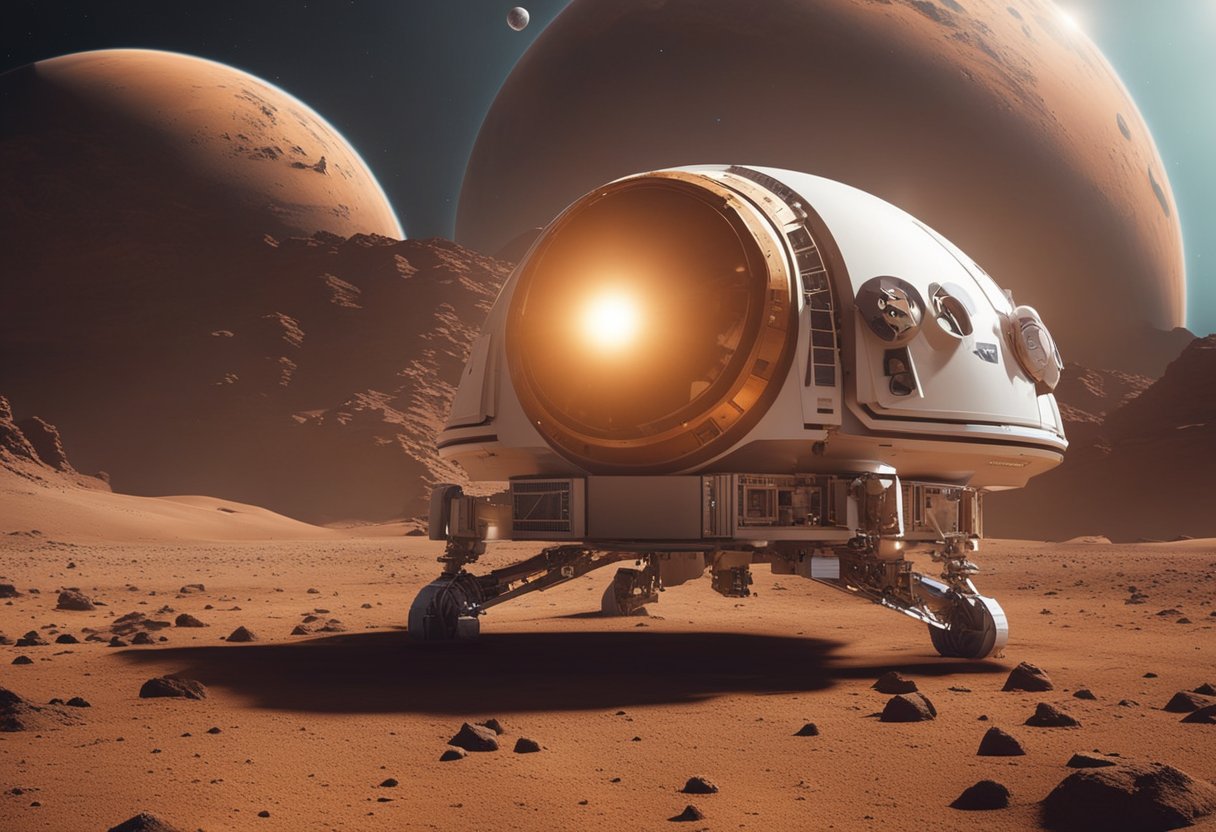
As we look ahead, the anticipation for Mars exploration is marked by significant advancements in technology and burgeoning private sector participation, setting the stage for ambitious human expeditions to the Red Planet, potentially within this decade.
Planned Human Exploration
We understand that NASA has set its sights on the 2030s for launching human missions to Mars. Engineers and scientists are working on critical deep space technologies that will ensure a safe and sustainable journey. The round-trip travel, including a 30-day stay, is expected to last about 500 days . A considerable part of the mission planning is focused on perfecting spacecraft systems capable of supporting life far from Earth’s conveniences and protections.
Private Sector Involvement
In parallel, the private sector, led by individuals like Elon Musk , has shown substantial interest in Mars exploration. Musk’s company, SpaceX, is developing the Starship rocket, which is envisioned to launch both cargo and humans to Mars. Their ambitious vision goes as far as to posit the establishment of a self-sustaining settlement on Mars. SpaceVoyageVentures.com, while documenting early space tourism efforts, keeps tabs on these developments, highlighting the imminent transition from mere orbital travel to the colonisation of other planets.
Mars Travel Timelines and Distance
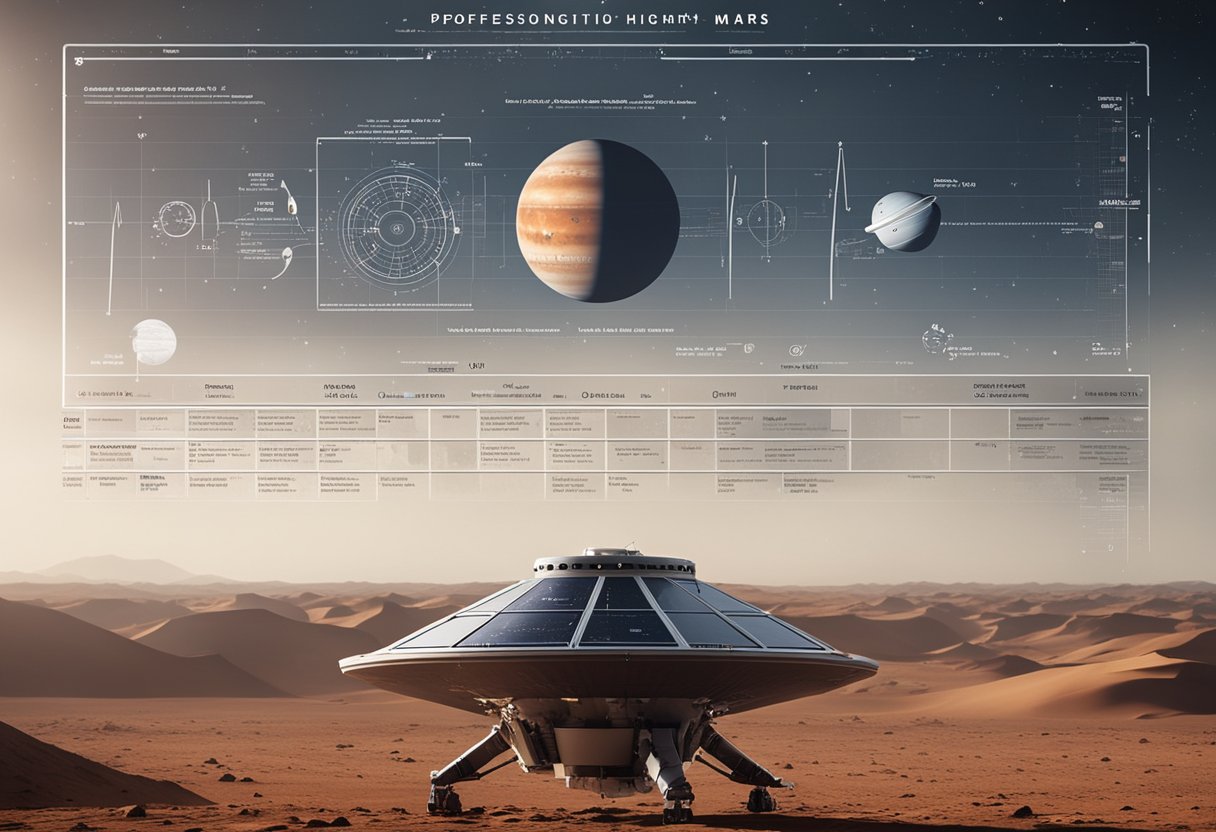
As we embark on the venture of space exploration, understanding the travel time and distance to Mars is crucial for successful missions. Our discussion covers the intricacies of these factors.
Optimal Launch Periods
Optimal launch windows for missions to Mars are dictated by the relative positions of Earth and Mars in their orbits. We look for a specific alignment known as the Hohmann transfer orbit , which occurs approximately every 26 months. This is when Mars and Earth are positioned in their orbits to allow the most energy-efficient path to the Red Planet.
Estimating Journey Time
The journey time to Mars can vary significantly based on the speed of the spacecraft and its chosen trajectory. Typically, travelling to Mars takes about seven months, a period during which a spacecraft covers the distance of roughly 480 million kilometres. Achieving a balance between speed and the amount of fuel carried is a delicate task that influences the total travel time . We might see these times decrease as advancements in propulsion technology are made, but for now, travellers must prepare for a substantial trip.
By adhering to these timelines and leveraging optimal alignment and launch periods, we ensure the most efficient use of resources and the greatest chance of mission success.
Frequently Asked Questions
In this section, we address some of the most common inquiries regarding the duration of Martian travel and the prospective advancements in space technology that underline our journey to the Red Planet.
How long does a journey to Mars typically take for unmanned spacecraft?
The travel time to Mars for unmanned spacecraft can vary but usually takes about six to nine months. This timespan fluctuates due to the relative positions of Earth and Mars along their orbits.
What is the shortest duration achieved for a space mission to Mars?
Historically, the quickest missions to Mars, such as the one undertaken by the New Horizons probe, have theoretically proposed the possibility of reaching the Martian surface in as little as 39 days, although practical application of such speed has yet to be implemented for Mars transit.
Why is the travel time to Mars often said to be close to two years?
The round trip to Mars is often cited as nearly two years due to the need to wait for a proper planetary alignment for the return journey, along with the stay on Mars itself, which adds to the total mission duration.
What advancements are expected from NASA’s Mars mission slated for 2026?
NASA is continuously exploring technological advances for its 2026 Mars mission, focusing on reducing travel time and improving spacecraft capabilities for greater autonomy and efficiency during the journey.
When is the first crewed mission to the Red Planet anticipated?
Various space agencies and private companies aim to send astronauts to Mars within the 2030s, with some early coordination and test missions serving as precursors to these ambitious crewed endeavours.
Can a trip to Mars truly take three decades, and what factors could cause such a duration?
A martian mission spanning three decades would be highly unusual and could only result from a long-term human settlement plan, extended research missions, unforeseen delays, or significant technical and logistical challenges that extend well beyond routine space travel operations.
Leave a Reply Cancel reply
Your email address will not be published. Required fields are marked *
Artemis Program Tourism: Gateway to Lunar Exploration for Civilians
Lunar orbit experiences: insights into space travel dynamics, the psychological effects of space travel on tourists: insights into the astronaut experience, the evolution of space tourism: how cosmic travel became attainable, the role of private companies in advancing space exploration initiatives, spaceports around the world: unveiling global launch sites.
Mars' subsurface is 'burping' out methane and scientists aren't sure why
Salt might play a key role in the mysterious behavior of methane seeps on Mars.
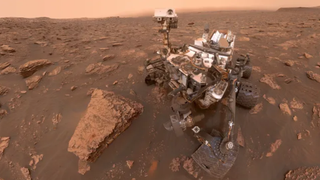
Since 2012, NASA's Curiosity rover has repeatedly detected methane on Mars, specifically near its landing site inside the 96-mile-wide (154 kilometers) Gale Crater.
But that Mars methane is behaving erratically. It only appears at night, it fluctuates seasonally and it spikes unexpectedly to levels 40 times higher than usual. To make things more puzzling, the gas isn't present in appreciable amounts high in the Martian atmosphere , and it hasn't been detected near the surface in other Red Planet locales. So what's going on at Gale Crater?
A group of NASA researchers led by planetary scientist Alexander Pavlov may now have at least a partial answer. The team suggests the Mars methane is trapped beneath a crust of solidified salt within the regolith at Gale. Warm daytime temperatures could weaken the crust, allowing methane to slip out at night. And the weight of a heavy rover driving over the crust could crack the crust, too, allowing methane to burst out in a concentrated puff. (Yes, it's akin to burping a baby.)
Related: NASA's Mars rover Curiosity: The ultimate guide
The researchers tested their hypothesis here on Earth , using a simulated Martian regolith; a salt called perchlorate, which exists widely on Mars; and neon as an analog for methane. Their tests, performed inside a Mars simulation chamber at NASA's Goddard Space Flight Center in Maryland, showed that a salt crust could form under certain conditions, trapping methane beneath it.
While a layer of solidified salt might explain the irregular behavior of Martian methane, scientists still don't know why methane even exists on Mars in the first place. On Earth, methane is primarily produced by living organisms — but we still haven't found signs of life on Mars .
And, to be clear, methane is not a surefire sign of life; the gas can be produced by geological processes as well.
Get the Space.com Newsletter
Breaking space news, the latest updates on rocket launches, skywatching events and more!
— Future Mars plane could help solve Red Planet methane mystery
— Mars methane mystery may be starting to clear up
— Future astronauts could make methane rocket fuel on Mars
"It's a story with a lot of plot twists," Ashwin Vasavada, Curiosity's project scientist at NASA's Jet Propulsion Laboratory in Southern California, said in a statement . "Some of the methane work will have to be left to future surface spacecraft that are more focused on answering these specific questions."
A paper on the team's research was published on March 9, 2024 , in the Journal of Geophysical Research.
Join our Space Forums to keep talking space on the latest missions, night sky and more! And if you have a news tip, correction or comment, let us know at: [email protected].
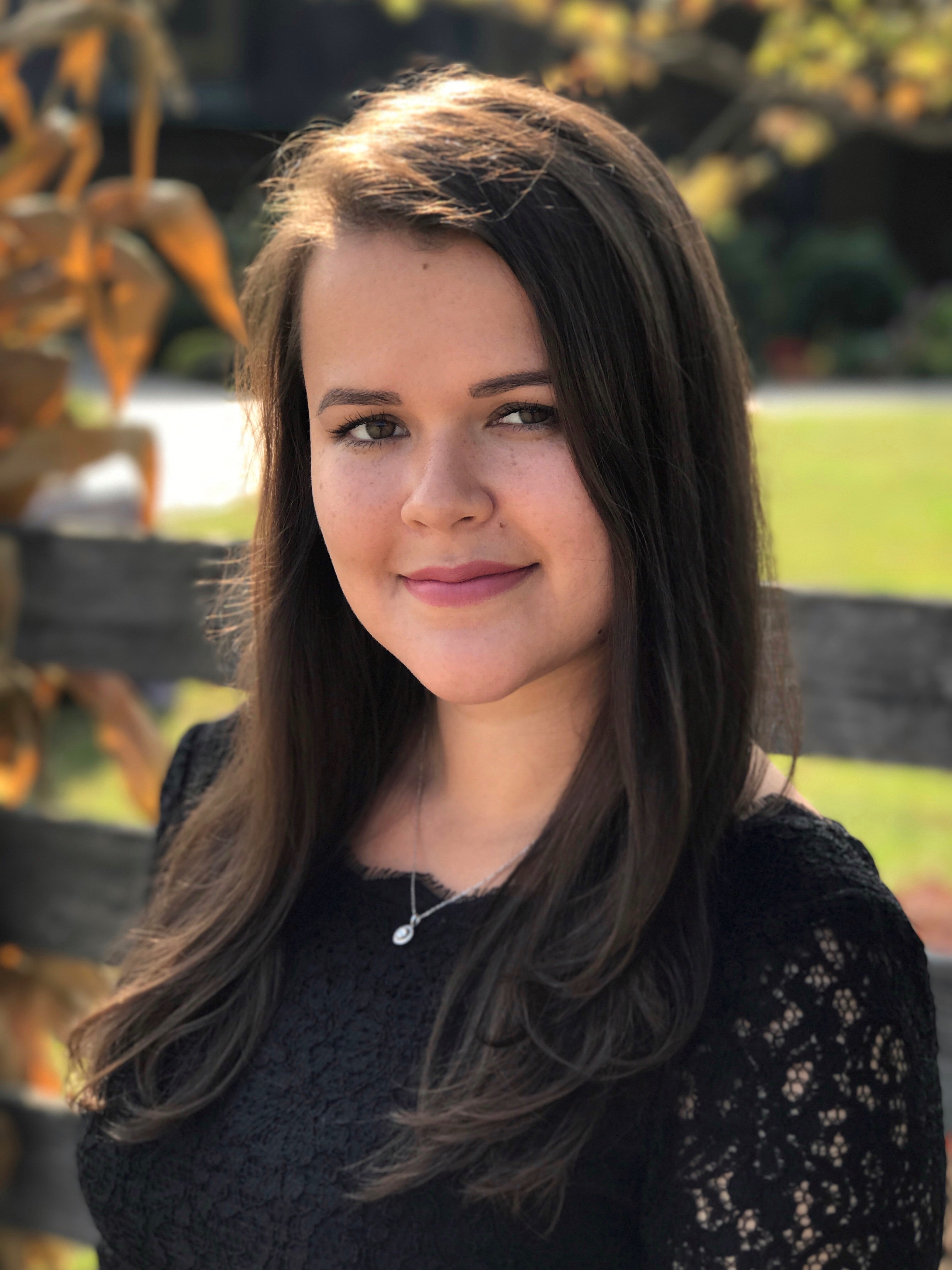
Space.com contributing writer Stefanie Waldek is a self-taught space nerd and aviation geek who is passionate about all things spaceflight and astronomy. With a background in travel and design journalism, as well as a Bachelor of Arts degree from New York University, she specializes in the budding space tourism industry and Earth-based astrotourism. In her free time, you can find her watching rocket launches or looking up at the stars, wondering what is out there. Learn more about her work at www.stefaniewaldek.com .
Satellites spot clusters of 'spiders' sprawled across Mars' Inca City (photo)
Ingenuity's travels: New NASA video tracks Mars helicopter's 72 flights
US Space Force picks Rocket Lab for 2025 Victus Haze space domain awareness mission
- Unclear Engineer And then there is this https://www.livescience.com/space/mars/hundreds-of-black-spiders-spotted-in-mysterious-inca-city-on-mars-in-new-satellite-photos . Get past the click bait headline, and see the description of the environmental physics that create these patterns. Reply
- View All 1 Comment
Most Popular
- 2 Wow! Private space-junk probe snaps historic photo of discarded rocket in orbit
- 3 Laser on NASA's Psyche asteroid probe beams data from 140 million miles away
- 4 Satellites spot clusters of 'spiders' sprawled across Mars' Inca City (photo)
- 5 5 sci-fi movies that weren't great but still deserve a sequel
- Articles >
The Moscow Metro Museum of Art: 10 Must-See Stations
There are few times one can claim having been on the subway all afternoon and loving it, but the Moscow Metro provides just that opportunity. While many cities boast famous public transport systems—New York’s subway, London’s underground, San Salvador’s chicken buses—few warrant hours of exploration. Moscow is different: Take one ride on the Metro, and you’ll find out that this network of railways can be so much more than point A to B drudgery.
The Metro began operating in 1935 with just thirteen stations, covering less than seven miles, but it has since grown into the world’s third busiest transit system ( Tokyo is first ), spanning about 200 miles and offering over 180 stops along the way. The construction of the Metro began under Joseph Stalin’s command, and being one of the USSR’s most ambitious building projects, the iron-fisted leader instructed designers to create a place full of svet (radiance) and svetloe budushchee (a radiant future), a palace for the people and a tribute to the Mother nation.
Consequently, the Metro is among the most memorable attractions in Moscow. The stations provide a unique collection of public art, comparable to anything the city’s galleries have to offer and providing a sense of the Soviet era, which is absent from the State National History Museum. Even better, touring the Metro delivers palpable, experiential moments, which many of us don’t get standing in front of painting or a case of coins.
Though tours are available , discovering the Moscow Metro on your own provides a much more comprehensive, truer experience, something much less sterile than following a guide. What better place is there to see the “real” Moscow than on mass transit: A few hours will expose you to characters and caricatures you’ll be hard-pressed to find dining near the Bolshoi Theater. You become part of the attraction, hear it in the screech of the train, feel it as hurried commuters brush by: The Metro sucks you beneath the city and churns you into the mix.
With the recommendations of our born-and-bred Muscovite students, my wife Emma and I have just taken a self-guided tour of what some locals consider the top ten stations of the Moscow Metro. What most satisfied me about our Metro tour was the sense of adventure . I loved following our route on the maps of the wagon walls as we circled the city, plotting out the course to the subsequent stops; having the weird sensation of being underground for nearly four hours; and discovering the next cavern of treasures, playing Indiana Jones for the afternoon, piecing together fragments of Russia’s mysterious history. It’s the ultimate interactive museum.
Top Ten Stations (In order of appearance)
Kievskaya station.
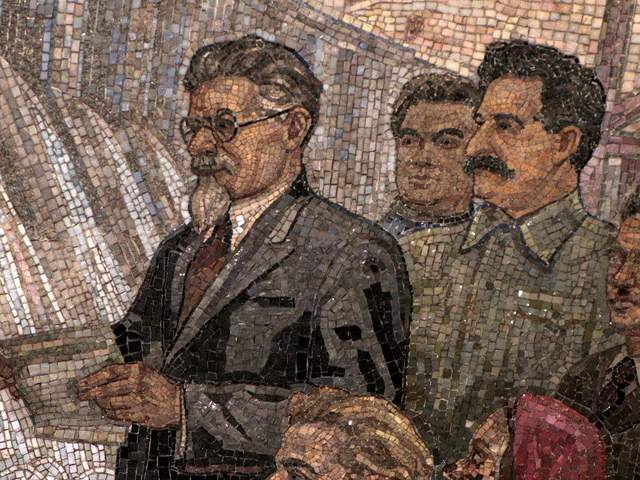
Kievskaya Station went public in March of 1937, the rails between it and Park Kultury Station being the first to cross the Moscow River. Kievskaya is full of mosaics depicting aristocratic scenes of Russian life, with great cameo appearances by Lenin, Trotsky, and Stalin. Each work has a Cyrillic title/explanation etched in the marble beneath it; however, if your Russian is rusty, you can just appreciate seeing familiar revolutionary dates like 1905 ( the Russian Revolution ) and 1917 ( the October Revolution ).
Mayakovskaya Station
Mayakovskaya Station ranks in my top three most notable Metro stations. Mayakovskaya just feels right, done Art Deco but no sense of gaudiness or pretention. The arches are adorned with rounded chrome piping and create feeling of being in a jukebox, but the roof’s expansive mosaics of the sky are the real showstopper. Subjects cleverly range from looking up at a high jumper, workers atop a building, spires of Orthodox cathedrals, to nimble aircraft humming by, a fleet of prop planes spelling out CCCP in the bluest of skies.
Novoslobodskaya Station
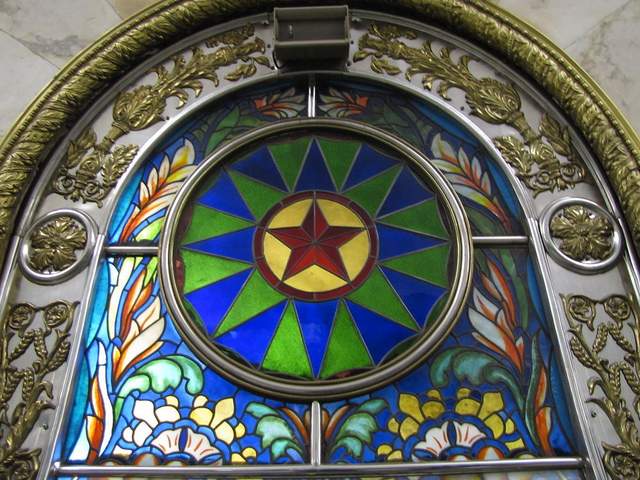
Novoslobodskaya is the Metro’s unique stained glass station. Each column has its own distinctive panels of colorful glass, most of them with a floral theme, some of them capturing the odd sailor, musician, artist, gardener, or stenographer in action. The glass is framed in Art Deco metalwork, and there is the lovely aspect of discovering panels in the less frequented haunches of the hall (on the trackside, between the incoming staircases). Novosblod is, I’ve been told, the favorite amongst out-of-town visitors.
Komsomolskaya Station
Komsomolskaya Station is one of palatial grandeur. It seems both magnificent and obligatory, like the presidential palace of a colonial city. The yellow ceiling has leafy, white concrete garland and a series of golden military mosaics accenting the tile mosaics of glorified Russian life. Switching lines here, the hallway has an Alice-in-Wonderland feel, impossibly long with decorative tile walls, culminating in a very old station left in a remarkable state of disrepair, offering a really tangible glimpse behind the palace walls.
Dostoevskaya Station
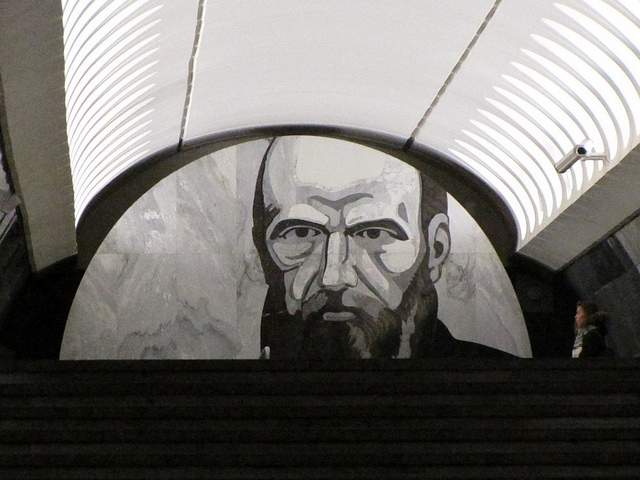
Dostoevskaya is a tribute to the late, great hero of Russian literature . The station at first glance seems bare and unimpressive, a stark marble platform without a whiff of reassembled chips of tile. However, two columns have eerie stone inlay collages of scenes from Dostoevsky’s work, including The Idiot , The Brothers Karamazov , and Crime and Punishment. Then, standing at the center of the platform, the marble creates a kaleidoscope of reflections. At the entrance, there is a large, inlay portrait of the author.
Chkalovskaya Station
Chkalovskaya does space Art Deco style (yet again). Chrome borders all. Passageways with curvy overhangs create the illusion of walking through the belly of a chic, new-age spacecraft. There are two (kos)mosaics, one at each end, with planetary subjects. Transferring here brings you above ground, where some rather elaborate metalwork is on display. By name similarity only, I’d expected Komsolskaya Station to deliver some kosmonaut décor; instead, it was Chkalovskaya that took us up to the space station.
Elektrozavodskaya Station
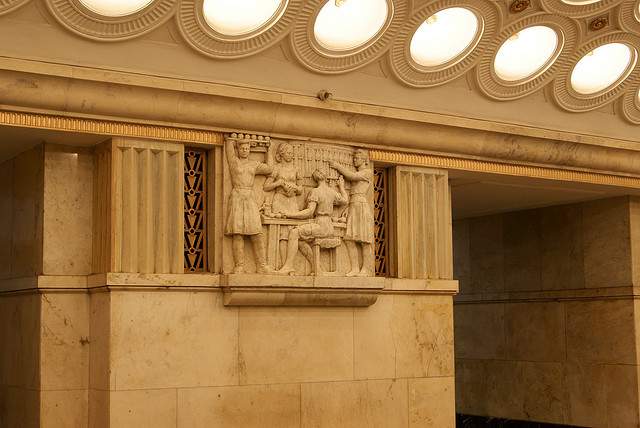
Elektrozavodskaya is full of marble reliefs of workers, men and women, laboring through the different stages of industry. The superhuman figures are round with muscles, Hollywood fit, and seemingly undeterred by each Herculean task they respectively perform. The station is chocked with brass, from hammer and sickle light fixtures to beautiful, angular framework up the innards of the columns. The station’s art pieces are less clever or extravagant than others, but identifying the different stages of industry is entertaining.
Baumanskaya Statio
Baumanskaya Station is the only stop that wasn’t suggested by the students. Pulling in, the network of statues was just too enticing: Out of half-circle depressions in the platform’s columns, the USSR’s proud and powerful labor force again flaunts its success. Pilots, blacksmiths, politicians, and artists have all congregated, posing amongst more Art Deco framing. At the far end, a massive Soviet flag dons the face of Lenin and banners for ’05, ’17, and ‘45. Standing in front of the flag, you can play with the echoing roof.
Ploshchad Revolutsii Station
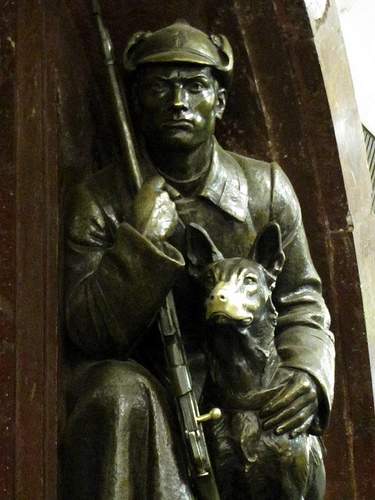
Novokuznetskaya Station
Novokuznetskaya Station finishes off this tour, more or less, where it started: beautiful mosaics. This station recalls the skyward-facing pieces from Mayakovskaya (Station #2), only with a little larger pictures in a more cramped, very trafficked area. Due to a line of street lamps in the center of the platform, it has the atmosphere of a bustling market. The more inventive sky scenes include a man on a ladder, women picking fruit, and a tank-dozer being craned in. The station’s also has a handsome black-and-white stone mural.
Here is a map and a brief description of our route:
Start at (1)Kievskaya on the “ring line” (look for the squares at the bottom of the platform signs to help you navigate—the ring line is #5, brown line) and go north to Belorusskaya, make a quick switch to the Dark Green/#2 line, and go south one stop to (2)Mayakovskaya. Backtrack to the ring line—Brown/#5—and continue north, getting off at (3)Novosblodskaya and (4)Komsolskaya. At Komsolskaya Station, transfer to the Red/#1 line, go south for two stops to Chistye Prudy, and get on the Light Green/#10 line going north. Take a look at (5)Dostoevskaya Station on the northern segment of Light Green/#10 line then change directions and head south to (6)Chkalovskaya, which offers a transfer to the Dark Blue/#3 line, going west, away from the city center. Have a look (7)Elektroskaya Station before backtracking into the center of Moscow, stopping off at (8)Baumskaya, getting off the Dark Blue/#3 line at (9)Ploschad Revolyutsii. Change to the Dark Green/#2 line and go south one stop to see (10)Novokuznetskaya Station.
Check out our new Moscow Indie Travel Guide , book a flight to Moscow and read 10 Bars with Views Worth Blowing the Budget For
Jonathon Engels, formerly a patron saint of misadventure, has been stumbling his way across cultural borders since 2005 and is currently volunteering in the mountains outside of Antigua, Guatemala. For more of his work, visit his website and blog .

Photo credits: SergeyRod , all others courtesy of the author and may not be used without permission
View prices for your travel dates
- Excellent 6
- Very Good 11
- All languages ( 25 )
- Russian ( 25 )
- English ( 0 )
Own or manage this property? Claim your listing for free to respond to reviews, update your profile and much more.
ELEKTROSTAL HOTEL
Firebird Travel
RUSSIA TRAVEL HOME
Thank you for your enquiry.
RUSSIA TRAVEL PACKAGES A selection of Russian tours to take as they are or adjust to your needs.
THE GOLDEN RING Visit the heart of ancient Russia. What is the Golden Ring?
MOSCOW TOURS What you can see in Moscow.
MOSCOW DAY TRIPS Get out of Moscow and take a relaxing trip to some of these places
ST. PETERSBURG Some of the sights to see in Petersburg
LAKE BAIKAL TOURS Hiking and trekking around the world's deepest lake in the heart of Siberia
RUSSIAN DIGS Come and work in the field on a Russian Archaeological dig. Full training given on site.
TRAVEL TIPS & SERVICES Getting around in Russia
If you do not receive a confirmation email shortly then you have probably incorrectly entered your email.
Number of travelers ">
Special Interests or requests. "> ">
If you experience difficulties please use this link to send Regular Email . All information is treated as confidential
Claudia Looi
Touring the Top 10 Moscow Metro Stations
By Claudia Looi 2 Comments
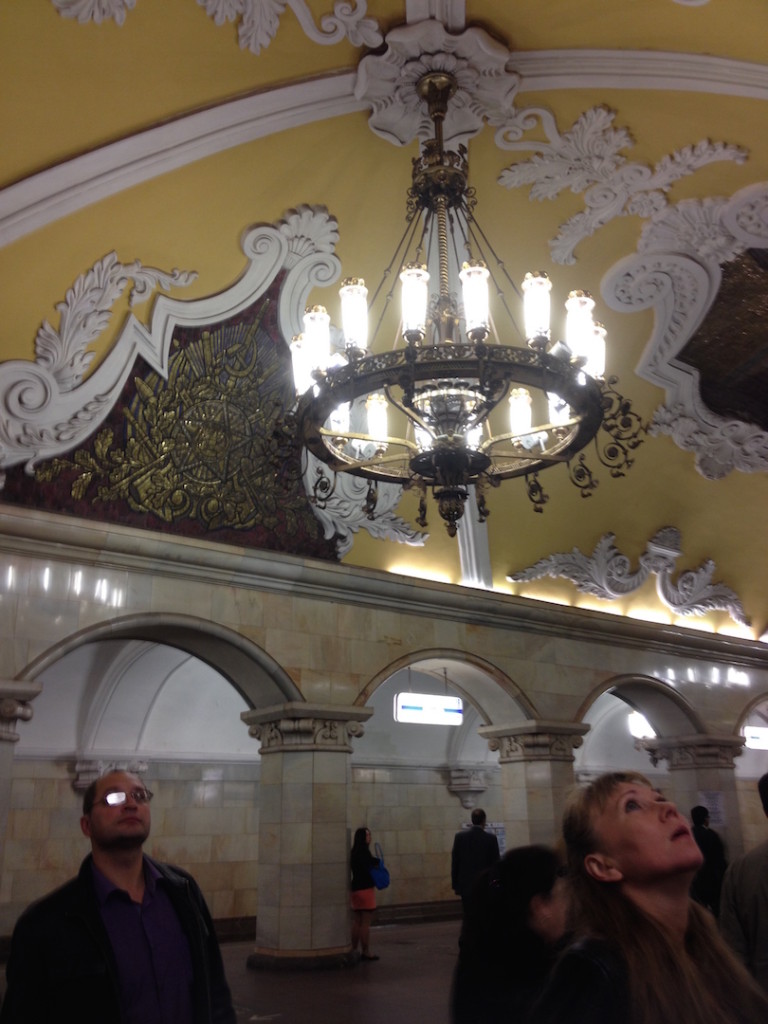
Komsomolskaya metro station looks like a museum. It has vaulted ceilings and baroque decor.
Hidden underground, in the heart of Moscow, are historical and architectural treasures of Russia. These are Soviet-era creations – the metro stations of Moscow.
Our guide Maria introduced these elaborate metro stations as “the palaces for the people.” Built between 1937 and 1955, each station holds its own history and stories. Stalin had the idea of building beautiful underground spaces that the masses could enjoy. They would look like museums, art centers, concert halls, palaces and churches. Each would have a different theme. None would be alike.
The two-hour private tour was with a former Intourist tour guide named Maria. Maria lived in Moscow all her life and through the communist era of 60s to 90s. She has been a tour guide for more than 30 years. Being in her 60s, she moved rather quickly for her age. We traveled and crammed with Maria and other Muscovites on the metro to visit 10 different metro stations.
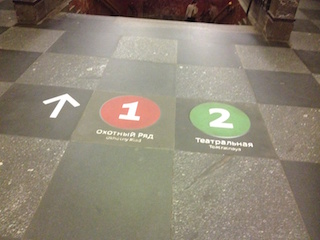
Arrow showing the direction of metro line 1 and 2
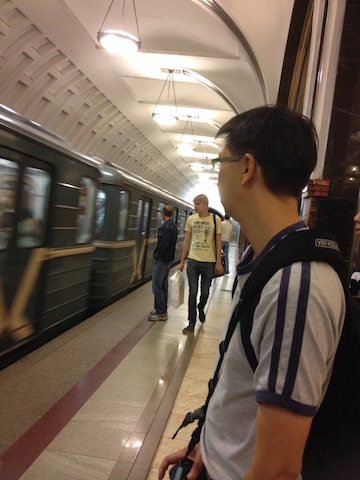
Moscow subways are very clean
To Maria, every street, metro and building told a story. I couldn’t keep up with her stories. I don’t remember most of what she said because I was just thrilled being in Moscow. Added to that, she spilled out so many Russian words and names, which to one who can’t read Cyrillic, sounded so foreign and could be easily forgotten.
The metro tour was the first part of our all day tour of Moscow with Maria. Here are the stations we visited:
1. Komsomolskaya Metro Station is the most beautiful of them all. Painted yellow and decorated with chandeliers, gold leaves and semi precious stones, the station looks like a stately museum. And possibly decorated like a palace. I saw Komsomolskaya first, before the rest of the stations upon arrival in Moscow by train from St. Petersburg.
2. Revolution Square Metro Station (Ploshchad Revolyutsii) has marble arches and 72 bronze sculptures designed by Alexey Dushkin. The marble arches are flanked by the bronze sculptures. If you look closely you will see passersby touching the bronze dog's nose. Legend has it that good luck comes to those who touch the dog's nose.
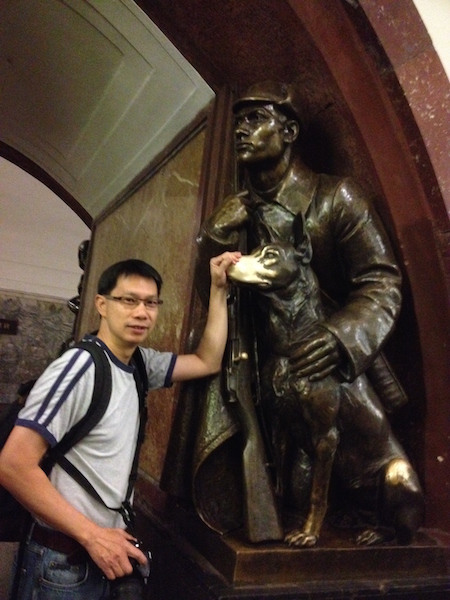
Touch the dog's nose for good luck. At the Revolution Square station
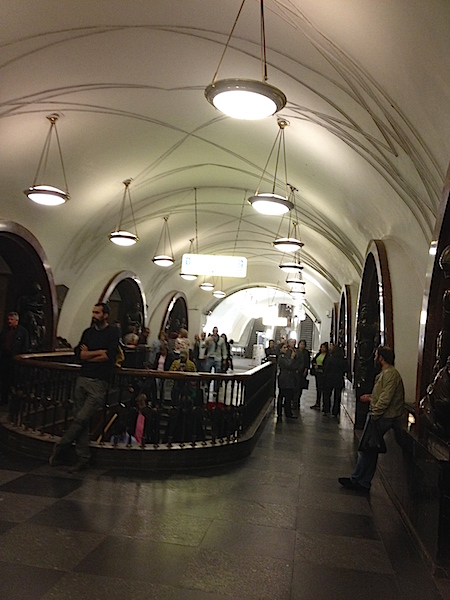
Revolution Square Metro Station
3. Arbatskaya Metro Station served as a shelter during the Soviet-era. It is one of the largest and the deepest metro stations in Moscow.
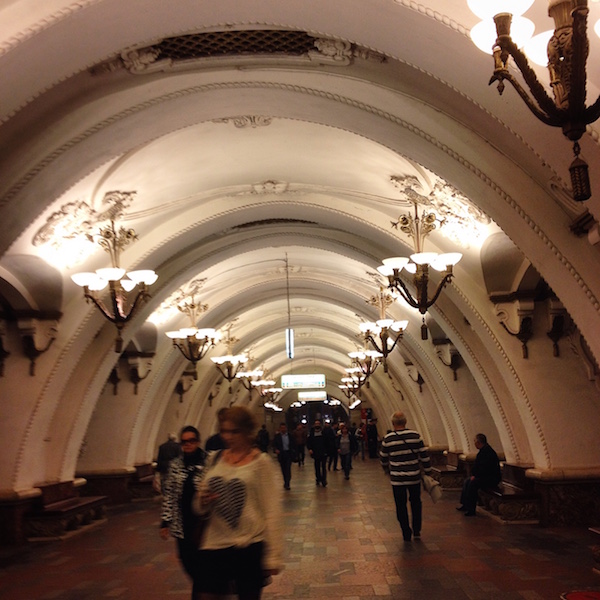
Arbatskaya Metro Station
4. Biblioteka Imeni Lenina Metro Station was built in 1935 and named after the Russian State Library. It is located near the library and has a big mosaic portrait of Lenin and yellow ceramic tiles on the track walls.
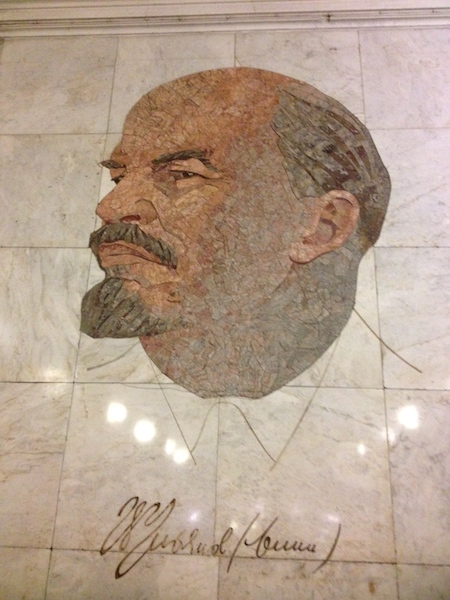
Lenin's portrait at the Biblioteka Imeni Lenina Metro Station
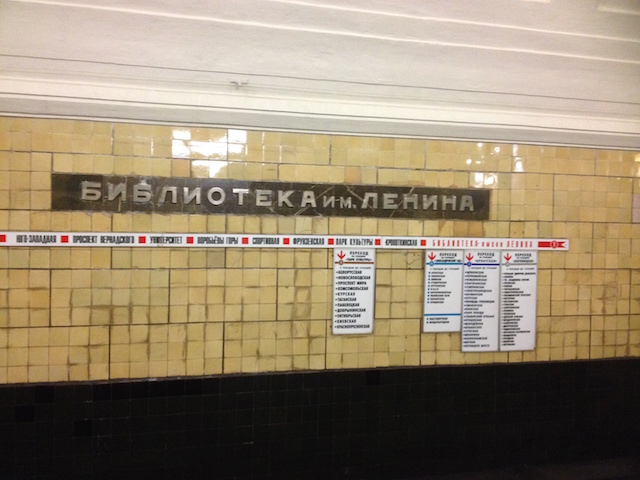
5. Kievskaya Metro Station was one of the first to be completed in Moscow. Named after the capital city of Ukraine by Kiev-born, Nikita Khruschev, Stalin's successor.
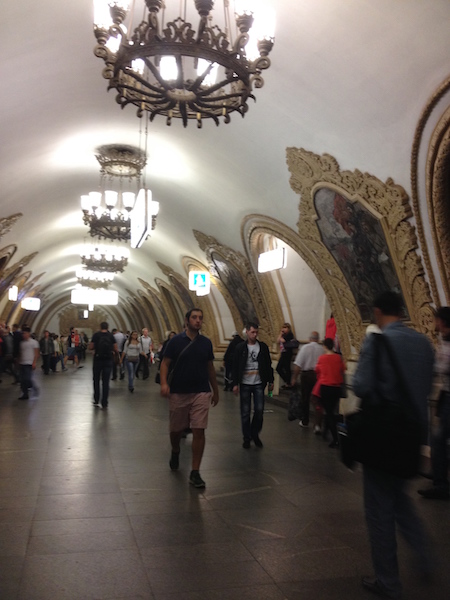
Kievskaya Metro Station
6. Novoslobodskaya Metro Station was built in 1952. It has 32 stained glass murals with brass borders.
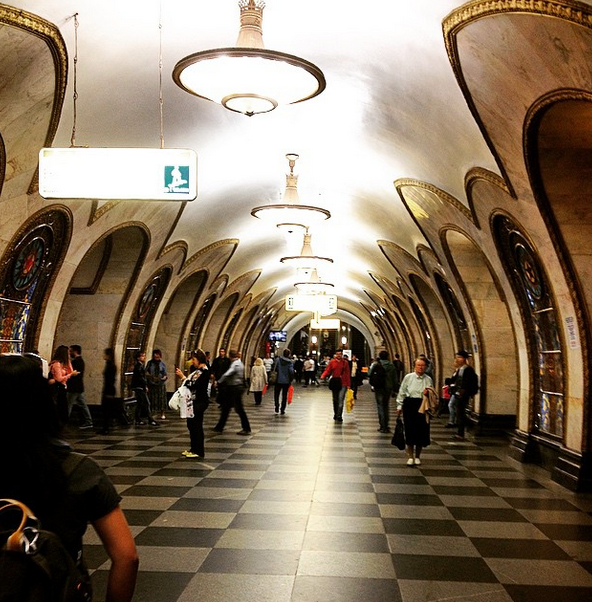
Novoslobodskaya metro station
7. Kurskaya Metro Station was one of the first few to be built in Moscow in 1938. It has ceiling panels and artwork showing Soviet leadership, Soviet lifestyle and political power. It has a dome with patriotic slogans decorated with red stars representing the Soviet's World War II Hall of Fame. Kurskaya Metro Station is a must-visit station in Moscow.

Ceiling panel and artworks at Kurskaya Metro Station
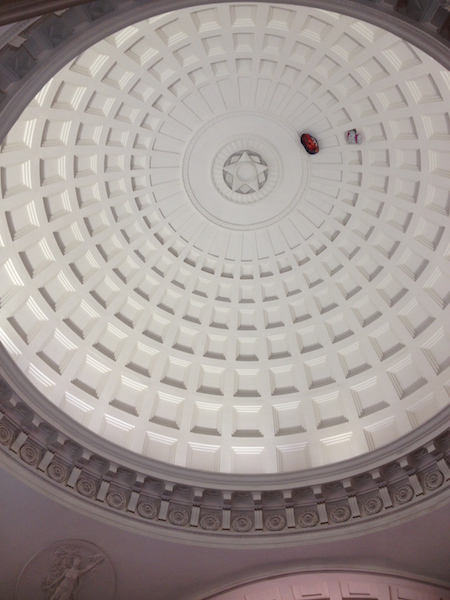
8. Mayakovskaya Metro Station built in 1938. It was named after Russian poet Vladmir Mayakovsky. This is one of the most beautiful metro stations in the world with 34 mosaics painted by Alexander Deyneka.
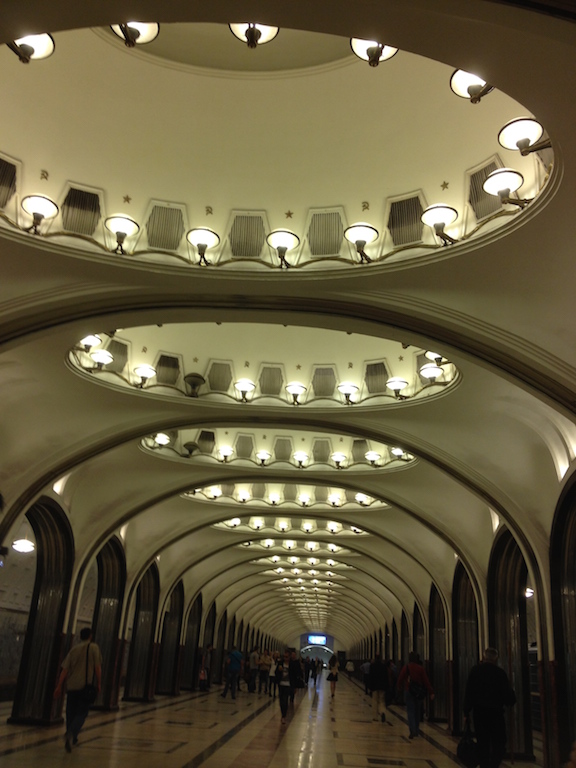
Mayakovskaya station
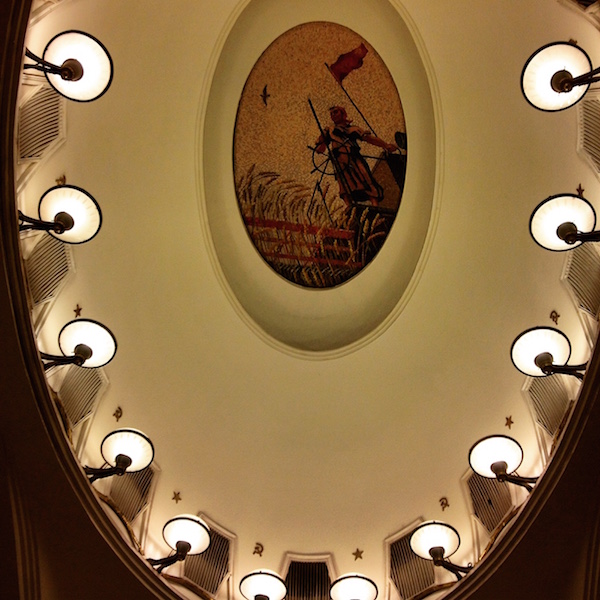
One of the over 30 ceiling mosaics in Mayakovskaya metro station
9. Belorusskaya Metro Station is named after the people of Belarus. In the picture below, there are statues of 3 members of the Partisan Resistance in Belarus during World War II. The statues were sculpted by Sergei Orlov, S. Rabinovich and I. Slonim.
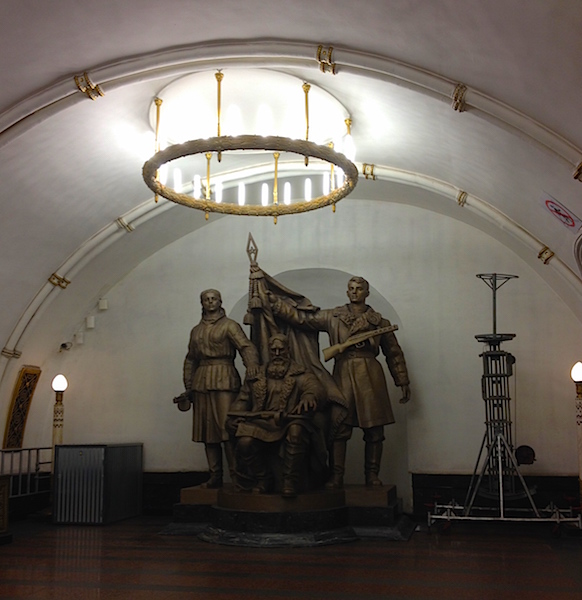
10. Teatralnaya Metro Station (Theatre Metro Station) is located near the Bolshoi Theatre.

Teatralnaya Metro Station decorated with porcelain figures .
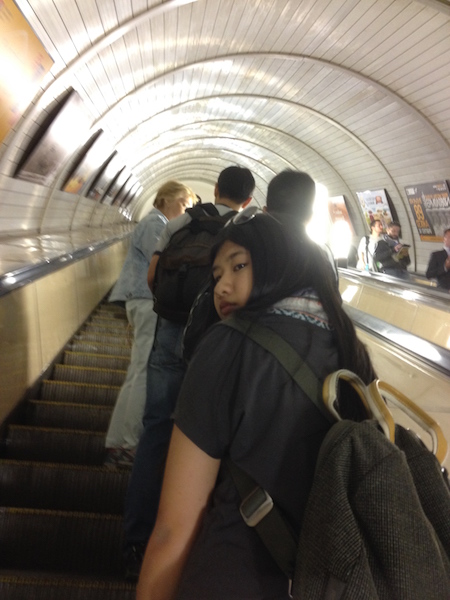
Taking the metro's escalator at the end of the tour with Maria the tour guide.
Have you visited the Moscow Metro? Leave your comment below.
January 15, 2017 at 8:17 am
An excellent read! Thanks for much for sharing the Russian metro system with us. We're heading to Moscow in April and exploring the metro stations were on our list and after reading your post, I'm even more excited to go visit them. Thanks again 🙂
December 6, 2017 at 10:45 pm
Hi, do you remember which tour company you contacted for this tour?
Leave a Reply Cancel reply
You must be logged in to post a comment.
Please go to the Instagram Feed settings page to create a feed.
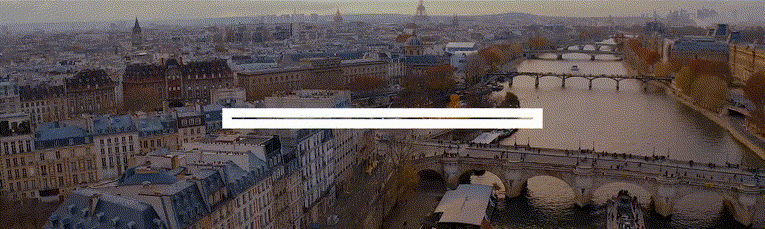
IMAGES
COMMENTS
Temperatures on Mars can range from -284 degrees F to 86 degrees F. The atmosphere on Mars is 96% carbon dioxide. One day on Mars lasts about 37 minutes longer than an Earth day. A year on Mars is almost twice as long as a year on Earth. Gravity on Mars is about one-third of the gravity on Earth.
Valles Marineris. NASA. Mars not only hosts the largest volcano of the solar system, but also the largest canyon. Valles Marineris is roughly 1850 miles (3000 km) long, according to NASA. That's ...
The prospect of Mars tourism tantalizes with its blend of extreme adventure and unprecedented exploration, forming the next potential leap for the travel industry. Once the stuff of science fiction, the idea is inching closer to reality as advancements in spacecraft technology improve and plans for human settlement on the Red Planet take shape.
LANDING ON MARS. Starship will enter Mars' atmosphere at 7.5 kilometers per second and decelerate aerodynamically. The vehicle's heat shield is designed to withstand multiple entries, but given that the vehicle is coming into Mars' atmosphere so hot, we still expect to see some ablation of the heat shield (similar to wear and tear on a brake pad).
Oct 08, 2015. RELEASE 15-206. NASA is leading our nation and the world on a journey to Mars, and Thursday the agency released a detailed outline of that plan in its report, "NASA's Journey to Mars: Pioneering Next Steps in Space Exploration.". "NASA is closer to sending American astronauts to Mars than at any point in our history ...
Jason Lyon. By Debra Kamin. May 7, 2022. Ilida Alvarez has dreamed of traveling to space since she was a child. But Ms. Alvarez, a legal-mediation firm owner, is afraid of flying, and she isn't ...
The Mars program will help with space tourism by improving engineering expertise with space docking, launches, and reentry and providing additional experience about the impact of space travel on ...
2021 Saw Progress on Mars, Rise in Space Tourists. December 26, 2021. NASA's Perseverance Mars rover took a 'selfie' with the Ingenuity helicopter, seen here about 13 feet (3.9 meters) from the ...
Based out of Florida's Kennedy Space Center, Space Perspective just notched $7 million in seed financing to get its Spaceship Neptune project ( ahem) off the ground. Founder and Co-CEO Taber ...
By Michael Greshko. June 16, 2016. • 3 min read. NASA has taken a nostalgic approach to promoting its Journey to Mars initiative: stunning travel posters that highlight Mars and its moons in all ...
The future of spaceflight—from orbital vacations to humans on Mars. NASA aims to travel to the moon again—and beyond. Here's a look at the 21st-century race to send humans into space.
Neukum Crater. Nearing the end of our tour is one of the oldest regions of Mars that dates back to at least 3.9 billion years ago, Neukum Crater. Scientists think this crater formed from a ...
Take remote learning to a new level with free virtual and augmented reality experiences. Use Access Mars to take a field trip to the Red Planet. Visit the Mars Curiosity rover and see important landmarks on the planet's surface. Users need a cellphone or computer and an internet connection for this interactive, narrated experience.
Mars - JPL Travel Poster. NASA's Mars Exploration Program seeks to understand whether Mars was, is, or can be a habitable world. Missions like Mars Pathfinder, Mars Exploration Rovers, Mars Science Laboratory and Mars Reconnaissance Orbiter, among many others, have provided important information in understanding of the habitability of Mars.
Catch the Earth rising above the Moon's horizon, against the backdrop of black, empty space. A view of the Earth's continents, seas, and clouds - all in one glimpse - is breathtaking. Seeing the Earth from the Moon could shift our perspective and change the way we think about life and everything in the Universe.
Space tourism is human space travel for recreational purposes. ... The Inspiration Mars Foundation is an American nonprofit organization founded by Dennis Tito that proposed to launch a crewed mission to flyby Mars in January 2018, or 2021 if they missed the first deadline. Their ...
An all-inclusive tour to Mars might consist of an extended space travel (back and forth), a temporary integration in the Martian population, optionally with hard work experience and likely include a surface exploration tour. A very high class working holiday, in a sense. Later Mars tourism. As travel cost go down, the number of tourists should ...
NASA's plans for space exploration include a return to the Moon to stay—boots back on the lunar surface with an orbital outpost. This station will be a launch point for voyages to destinations ...
Venturing to Mars is a challenging and lengthy endeavour, requiring sophisticated technology, meticulous planning, and a deep understanding of the physics involved in long-distance space travel. On this journey spanning roughly 225 million kilometres, a spacecraft endures a multitude of factors, from the gravitational pulls of celestial bodies ...
Send Your Name to Mars is not currently open for reservations. We will reopen reservations closer to a future launch date. If you have already signed up to send your name to Mars on NASA's next flight to the Red Planet, your spot is reserved. Stay up to date by signing up for one of these […]
NASA's Mars Curiosity rover took this selfie on June 13, 2018. ... With a background in travel and design journalism, as well as a Bachelor of Arts degree from New York University, she specializes ...
The snacking favorite further solidifies its spot as the top snack for air travel ... Mars Snacking. "With international tourism expected to grow 15% in 2024 and over 6 million people flying every day, this campaign captures those increasingly and absurdly relatable instances we've all encountered traveling and reminds hungry travelers to ...
Have a look (7)Elektroskaya Station before backtracking into the center of Moscow, stopping off at (8)Baumskaya, getting off the Dark Blue/#3 line at (9)Ploschad Revolyutsii. Change to the Dark Green/#2 line and go south one stop to see (10)Novokuznetskaya Station. Check out our new Moscow Indie Travel Guide, book a flight to Moscow and read 10 ...
"With international tourism expected to grow 15 percent in 2024 and over six million people flying every day, this campaign captures those increasingly and absurdly relatable instances we've all encountered traveling and reminds hungry travelers to grab a SNICKERS, to help keep them on track as they navigate the turbulent skies of travel," BBDO Senior Vice President Kristin Clark said.
Similarly, Craft Travel has organized a busy, 15-day itinerary for a family of four, including two teenagers, from New York. Among the exclusive experiences is a private concert at the Palais ...
Elektrostal Hotel, Elektrostal: See 25 traveler reviews, 44 candid photos, and great deals for Elektrostal Hotel, ranked #1 of 2 B&Bs / inns in Elektrostal and rated 4 of 5 at Tripadvisor.
RUSSIA TRAVEL PACKAGES A selection of Russian tours to take as they are or adjust to your needs. THE GOLDEN RING Visit the heart of ancient Russia. What is the Golden Ring? MOSCOW TOURS What you can see in Moscow. MOSCOW DAY TRIPS Get out of Moscow and take a relaxing trip to some of these places.
6. Novoslobodskaya Metro Station was built in 1952. It has 32 stained glass murals with brass borders. Novoslobodskaya metro station. 7. Kurskaya Metro Station was one of the first few to be built in Moscow in 1938. It has ceiling panels and artwork showing Soviet leadership, Soviet lifestyle and political power.