Gamma rays: Everything you need to know about these powerful packets of energy
Gamma rays can only be detected by sensors made of dense metals and takes over six feet (1.8 meters) of concrete to block.
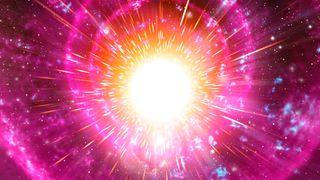
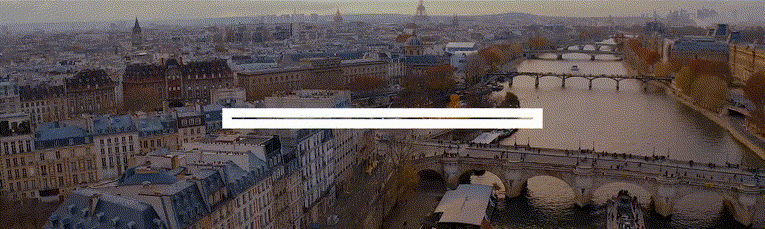
How were gamma rays discovered?
How to stop gamma rays, are gamma rays dangerous, gamma-ray astronomy, gamma-ray bursts, additional resources.
Gamma rays are high-energy photons produced by some of the most violent events in the universe.
Photons of light are massless particles that are essentially packets of energy. Because of a quantum-mechanical phenomenon known as wave-particle duality , particles can behave like waves, and photons are no different. Photons have wavelengths, and the amplitude of their wavelength determines where they sit on the electromagnetic spectrum . Radio and microwave photons sit at the lower energy, longer wavelength end of the spectrum, while in the shorter wavelength, higher-energy regime are photons of ultraviolet, X-rays and the most energetic of them all with the shortest wavelengths: gamma rays.
Gamma rays have wavelengths shorter than 10^-11 meters and frequencies above 30 x 10^18 hertz. The European Space Agency describes how gamma-ray photons have energies in excess of 100,000 electronvolts (eV). We can compare this to X-rays, which NASA describes as having energies between 100 eV and 100,000 eV , and optical photons that we can see with our eyes, which are about 1 eV.
Related: What is the cosmic microwave background?
On Earth, gamma rays are produced by radioactive decay, nuclear weapons and lightning, while in space they are produced by violent, high-energy sources such as solar flares , quasars , black holes tearing stars apart , black-hole accretion disks, exploding stars and the strong gravitational environments of neutron stars .
At the turn of the twentieth century, two forms of radiation emitted by decaying atoms were known, namely alpha particles (which are helium nuclei) and beta particles (which are electrons and positrons).
However, when the French chemist Paul Villard began experimenting with the radioactive element radium, which had been discovered two years prior by Marie and Pierre Curie , he noticed that the ionizing radiation produced by the decay of radium packed a harder punch than either alpha or beta particles.
This radiation received its name — gamma-rays — simply because gamma is the third letter in the Greek alphabet after alpha and beta. Unbeknownst to Villard and his cohorts in the early 1900s, the key difference between gamma rays and alpha/beta particles is that gamma rays are a form of light, whereas alpha and beta particles are made of matter.
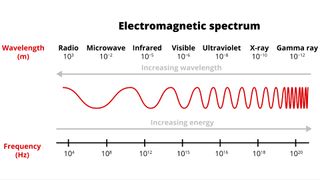
To block gamma rays requires a dense material, and the thickness of that material depends on the substance. To reduce the strength of incoming gamma rays by a billion, you need 13.8 feet (4.2 meters) of water, 6.6 feet (2 m) of concrete or 1.3 feet (0.39 m) of lead, according to the radiation protection solution website StemRad .
This poses a problem for gamma-ray telescopes, such as NASA's Fermi Space Telescope . Ordinary telescopes like the Hubble Space Telescope use mirrors and lenses to collect and focus light, but gamma rays will simply pass straight through an ordinary telescope. Instead, gamma-ray telescopes have to employ other means.
On the Fermi Space Telescope, a gamma-ray photon will pass through a device called the Anti-coincidence Detector, which blocks cosmic rays that might give a false signal, according to NASA . The gamma-ray is then absorbed by one of 16 sheets of tungsten, a material that is dense enough to stop gamma rays.
By interacting with the tungsten, the gamma-ray is converted into an electron and a positron (the antimatter or antiparticle counterpart of an electron), the paths of which are read by a tracker, which is a module of silicon strips interweaved by tungsten foil that can determine the direction that the gamma-ray came from in space, based on the trajectory of the electron and the positron.
Finally, the electron and then positron have their energies measured by a calorimeter — a device that measures the energy of a particle by absorbing it — made from cesium iodide, and therefore the energy of the gamma-ray can be determined.
Because of their high energy, gamma rays are ionizing, meaning they can dislodge electrons from atoms , ultimately damaging living cells and causing a hazard to health. However, as with all radiation, it depends upon the dose that you receive.
In small doses, very carefully targeted to limit exposure, they can be used safely as a medical diagnostic tool, or even to kill cancerous cells (ironic since exposure to radiation including gamma rays can cause cancer). In particular, one tool used by doctors is the ' Gamma Knife ', which is an ultra-precise form of surgery in which a beam of gamma rays cuts away diseased brain cells and can even penetrate deep into the brain without damaging the exterior lobes.
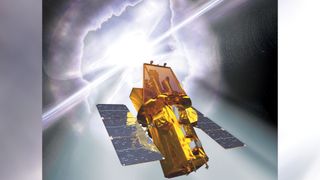
Given their ionizing power, it's fortunate that Earth's atmosphere is able to block gamma rays from space. For astronomers, however, that's unfortunate, because it means that to conduct gamma-ray astronomy observatories have to either be built on mountaintops where the atmosphere is thinner or sent into space.
The first gamma-ray space telescope was launched in 1961 on the NASA Explorer 11 satellite, but things didn't really begin to kick off until the late 1960s and early 1970s with a major finding, and it wasn't even an astronomical telescope that made the discovery.
Over the years there have been many observatories, both on the ground and in space, that have been designed to observe cosmic gamma-ray radiations. In 1990, NASA launched the Compton Gamma-Ray Observatory as the gamma-ray counterpart to the Hubble Space Telescope. The Compton Gamma-Ray Observatory explored the cosmos from 1991 until 2000. The aforementioned BeppoSAX was a joint Italian–Dutch mission that operated between 1996 and 2003, while NASA launched HETE-2 (the High-Energy Transient Explorer; HETE-1 had previously failed in orbit) that tracked down many GRBs between 2000 and 2008.
Currently, as of the end of 2022, several satellites , observatories and telescopes continue to conduct gamma-ray astronomy both on Earth and in space. NASA's Swift satellite , launched in 2004, combines both X-ray and gamma-ray observations, as does Italy's AGILE satellite launched in 2007. In 2002, the European Space Agency launched INTEGRAL , the International Gamma-Ray Astrophysics Laboratory.. The current most sophisticated gamma-ray space telescope is Fermi, which NASA launched in 2008.
Meanwhile, on the ground, there are several gamma-ray observatories including VERITAS (Very Energetic Radiation Imaging Telescope Array System) at the Fred Lawrence Whipple Observatory in Arizona and HESS (High Energy Stereoscopic System) in Namibia.
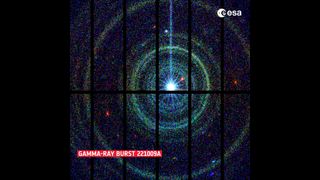
In 1963, the Soviet Union, the United Kingdom and the United States signed a nuclear test ban treaty that prohibited the world's superpowers from testing any nuclear devices in the atmosphere or in space. However, the U.S. was suspicious that the Soviet Union wouldn't adhere to the treaty, so they launched the Vela series of satellites to watch for any pulses of gamma-ray radiation that could be coming from secretive nuclear detonations. As it happened, gamma rays were detected, but from space: random blasts of powerful gamma-ray energy that seemed to be coming from all around the Earth. But how far away were these gamma-ray bursts ?
Related: Most powerful gamma-ray burst ever seen could help reveal how black holes are born
If these gamma-ray bursts, which are abbreviated to GRBs for short, were coming from our galaxy, then astronomers would detect them mostly in the plane of the Milky Way . Instead, they were spread all over the sky, it could mean only one of two things. Either they were very close, within our solar system , or they were very far away, beyond our galaxy. A special debate was even convened in 1995, echoing a similar ' Great Debate ' in 1920 between Harlow Shapley and Heber D. Curtis that discussed the size of our galaxy based on the distribution of globular clusters .
In the 1995 debate , chaired by Martin Rees, astronomer Bohdan Paczynski of Princeton University argued that GRBs came from very far away, while Donald Lamb of the University of Chicago reasoned that GRBs must be from close by because the energy required for them to be billions of light-years away would contravene the laws of physics.
Just two years later astronomers had their answer when the BeppoSAX satellite detected a gamma-ray burst that the William Herschel Telescope in the Canary Islands was able to quickly follow up on, in the process detecting the faint afterglow of whatever explosion had created the GRB. Measuring the redshift of the afterglow's light revealed it to have come from six billion light-years away. Bohdan Paczynski was right!
There are two main types of GRB. One type is called short GRBs which last just fractions of a second, while the other kind is known as the long GRBs, and can last many seconds up to an hour. Short GRBS are emitted during the merger of two neutron stars , while long GRBs are the death cries of rare, massive stars .
Physicists Andrew MacFadyen and Stan Woosley of the University of California, Santa Cruz, developed a model to explain how stars could explode and produce long GRBs without breaking the laws of physics. When a massive star with 50–100 times the mass of the sun reaches the end of its life, the star begins to collapse in on its core, and if the star is rotating fast enough, the energy within the collapsing layers rebounds off the core and is blasted out in two jets that move at almost the speed of light and blow the star apart. Charged particles within these jets spiral around powerful magnetic fields and produce something called synchrotron radiation, in the form of the gamma rays that we observe. Because the gamma rays are only released in the direction of the jets, and not in all directions at once, the total energy released does not contravene the laws of physics.
Learn more about ionizing radiation with the United States Environment Protection Agency (EPA) , and the American Cancer Society . Explore gamma rays in more detail in a tour of the electromagnetic spectrum with NASA Science.
Follow Keith Cooper on Twitter @21stCenturySETI. Follow us on Twitter @Spacedotcom and on Facebook .
Bibliography
Flash! The Hunt for the Biggest Explosions in the Universe by Govert Schilling (Cambridge University Press, 2002)
The Biggest Bangs: The Mystery of Gamma-Ray Bursts, The Most Violent Explosions in the Universe by Jonathan Katz (Oxford University Press, 2002)
Join our Space Forums to keep talking space on the latest missions, night sky and more! And if you have a news tip, correction or comment, let us know at: [email protected].
Get the Space.com Newsletter
Breaking space news, the latest updates on rocket launches, skywatching events and more!
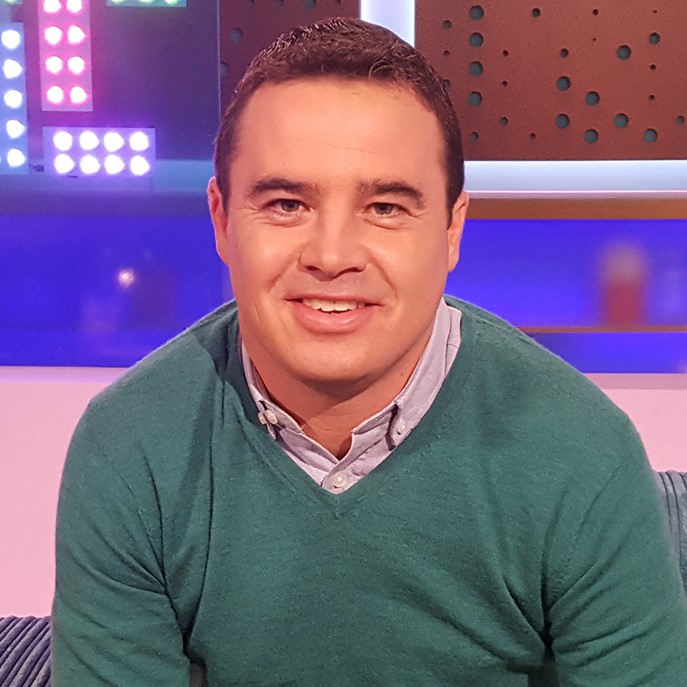
Keith Cooper is a freelance science journalist and editor in the United Kingdom, and has a degree in physics and astrophysics from the University of Manchester. He's the author of "The Contact Paradox: Challenging Our Assumptions in the Search for Extraterrestrial Intelligence" (Bloomsbury Sigma, 2020) and has written articles on astronomy, space, physics and astrobiology for a multitude of magazines and websites.
A 'snowball fight' may help scientists find life on Jupiter's moon Europa
Arrokoth the 'space snowman' and other Kuiper Belt objects may be packed with ancient ice
The total solar eclipse 2024 is happening today! Here's what you need to know
- larbud Interesting; no mention of 1859. Reply
- billslugg The 1859 Carrington event was caused by a stream of charged particles from a solar flare. Any gamma rays emitted would have been blocked by the Earth's atmosphere. Reply
- View All 2 Comments
Most Popular
By Tantse Walter February 06, 2024
By Fran Ruiz January 29, 2024
By Fran Ruiz January 26, 2024
By Conor Feehly January 05, 2024
By Keith Cooper December 22, 2023
By Fran Ruiz December 20, 2023
By Fran Ruiz December 19, 2023
By Fran Ruiz December 18, 2023
By Tantse Walter December 18, 2023
By Robert Lea December 05, 2023
By Robert Lea December 04, 2023
- 2 Maya nobility performed bloodletting sacrifices to strengthen a 'dying' sun god during solar eclipses
- 3 Coding the cosmos: Building an app for the total solar eclipse 2024 (op-ed)
- 4 My dogs and I watched the 2017 total solar eclipse, but we won't travel for this one
- 5 SpaceX rocket launches 11 satellites, including one for South Korea, on Bandwagon-1 rideshare flight (photos)
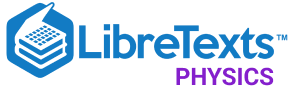
- school Campus Bookshelves
- menu_book Bookshelves
- perm_media Learning Objects
- login Login
- how_to_reg Request Instructor Account
- hub Instructor Commons
- Download Page (PDF)
- Download Full Book (PDF)
- Periodic Table
- Physics Constants
- Scientific Calculator
- Reference & Cite
- Tools expand_more
- Readability
selected template will load here
This action is not available.
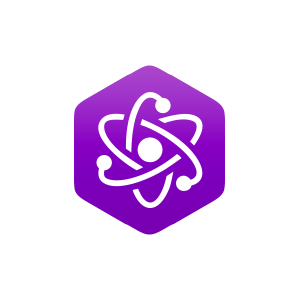
2.3: The Electromagnetic Spectrum
- Last updated
- Save as PDF
- Page ID 30463

- Kim Coble, Kevin McLin, & Lynn Cominsky
- San Francisco State University, Chico State University, & Sonoma State University
Learning Objectives
- You will be able to rank the different bandpasses in the EM Spectrum by energy, frequency, and wavelength
What Do You Think: Radio Waves
On their way to a concert, three students decide to listen to the radio.
- Angela: “I wonder if radio telescopes work the same way as the radio we’re listening to. You know, by picking up sound waves that we can hear.”
- Brianna: “I don’t think so, there’s no air in space for the sound waves to travel through, so you wouldn’t be able to get any signals.”
- Callie: “Right, radio waves are light waves. The radio is really detecting light waves in the radio portion of the spectrum and converting them into sound waves that we can hear.”
Do you agree with any of these students, and if so, whom?
While much can be learned from observing objects in visible light—the kind of light we see with our eyes—there is a tremendous amount of additional information carried only by other kinds of light. Over the past half-century or so, astronomers have learned to observe the Universe not only in visible light, but in all bands of the electromagnetic (EM) spectrum, from low-energy radio waves all the way up to high-energy gamma-rays. Figure 2.2 shows the (EM) spectrum.
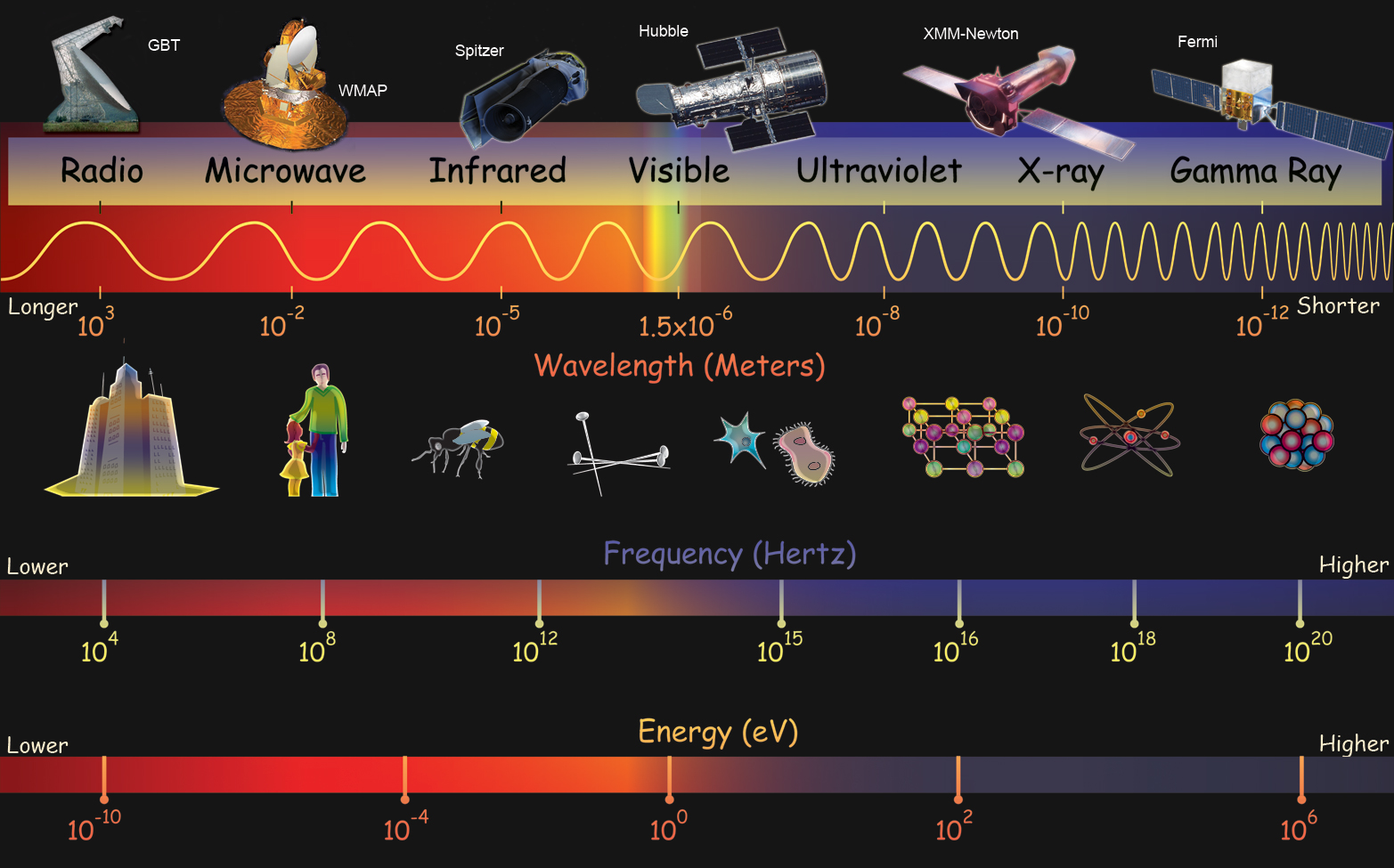
The EM spectrum, of which visible light is a tiny part, is divided into somewhat arbitrary regions, or bands, that are based on how the light in that region is measured. These different methods of detecting or measuring the light result from the different wavelengths or frequencies of the light in each band. To give you an overview, and to put some useful labels on what we are discussing, we will briefly describe the different bands of the EM spectrum. It turns out that most portions of the EM spectrum are not visible to our eyes.
- Radio —At the longest wavelength end of the EM spectrum are the radio waves. This is the kind of light used to carry radio signals to a stereo in your car and to your television (if you receive broadcast TV and not cable). Are you surprised to find that radio is a kind of light, and not a kind of sound? Many people are surprised by this fact when they first learn about it. Since astronomical radio waves have such long wavelengths, they are usually detected using telescopes that look like big dishes. There is no limit to the length of radio waves (on the long end), but the shortest radio waves have lengths of about a meter.
- Microwaves —At the high-frequency end of the radio spectrum are microwaves. They are the types of waves used in microwave ovens, where they interact with the water molecules in your food to heat it up. They also carry the signals used by cell phones. Although the prefix “micro” usually means “one-millionth,” microwaves have typical wavelengths of centimeters (one-hundredth of a meter) to millimeters (one-thousandth of a meter).
- Infrared —At slightly higher energies than microwave is infrared light. Infrared (IR) light is between microwaves and the red end of the visible light spectrum (infra is a prefix that means “below” — IR has a frequency below that of visible light). IR is sometimes called heat radiation, but this is a misnomer. As we will learn, all kinds of EM radiation are emitted by warm objects. But IR is emitted predominantly by objects we consider warm. Such objects include hot coals and warm-blooded animals (including people). Long-wavelength IR is also emitted by telescopes themselves. IR light has enough energy that it is not usually measured as a wave, but rather as a particle. IR photons have wavelengths in the range of hundreds to 1 or 2 microns (millionths of a meter).
- Visible Light —Next is visible light, also known as optical light. We perceive many different colors of visible light, which correspond to different frequencies of the light. At the low-frequency end is red, followed by orange, yellow, green, blue, and violet. One common mnemonic used to remember these colors in the proper order is ROY G. BIV (where indigo has been inserted to make the phrase easier). Check it out the next time you see a rainbow. Optical astronomy has been done using telescopes made of mirrors and lenses since the early 1600s, when Galileo Galilei first used a telescope to study the night sky. Visible light spans the wavelength range 400–700 nanometers (billionths of a meter), or if you prefer, it has wavelengths clustered around half a micron.
- Ultraviolet —Ultraviolet (UV) light is just beyond the blue end of the visible spectrum and it has somewhat higher frequencies than visible light. UV is what gives you a suntan (or sunburn with too much exposure). For the most part, exposure to light from radio through visible is generally without risk (but there are exceptions). At UV, this risk changes; too much exposure to light at UV or higher frequencies can be harmful or even fatal because these particles have enough energy to dislodge electrons from the atoms in your body (in a manner similar to the photoelectric effect ). Although some low-energy UV rays penetrate Earth’s atmosphere, most higher-energy UV does not. Hence, most UV astronomy is done from detectors launched into space. UV photons have wavelengths typically measured in tens to hundreds of nanometers.
- X-rays —X-rays have energies higher than UV. You probably know from visits to the doctor or dentist that x-rays can be used to create images of our insides. These images are not like normal photographs, however. They are really shadows. X-rays can penetrate many materials, but their penetrability depends on the density of the material. Thus, they pass easily through skin and muscles, but less easily through bones and teeth. As a result, x-ray images really just show contrast in the shadows caused by different parts of the body. They can also be used the same way to look into other materials, like machines and machine parts. X-ray images of astronomical objects are very different because the objects are emitting x-ray photons and the telescope is just catching them. Since Earth’s atmosphere absorbs x-rays from space, x-ray astronomy is always done from instruments in space. X-rays have so much energy that we tend to describe them using the energy of individual photons, rather than specifying their wavelengths or frequencies. X-ray energies start at 1 keV (kilo or thousands of electron volts), which are a more convenient measurement than using the SI unit joules. (One eV is equal to 1.6 × 10 -19 J.)
- Gamma-rays —The highest energy light is called gamma-rays. At low energies, gamma-rays merge into x-rays. In principle, there is no upper limit to gamma-ray energies; their energies could go to higher and higher values. In practice, we will see there are practical limits to how high the energy of a gamma-ray can go. Gamma-rays are the least familiar kind of light because they are almost completely absent on the Earth’s surface. This is a good thing. Gamma-rays interact so strongly with matter (including the matter comprising the proteins and DNA in our bodies) that a small exposure to them can be harmful, or even fatal. Gamma-ray energies start around 1 MeV (mega or million electron volts). The highest gamma-ray energies that have been directly measured from astronomical objects are in the TeV (tera or trillion electron volt ) range, though indirectly detected gamma rays have been seen with energies more than a million times higher.
Now that you have learned about the different bands of the electromagnetic spectrum, you will do some activities exploring wavelength, frequency, energy, and speed.
RANKING THE EM SPECTRUM BANDS
A. Wavelength.
Rank the different bands of the EM spectrum in order from shorter wavelength (on the left) to longer wavelength (on the right).
Play Activity
Rank the different bands of the EM spectrum in order from lower energy (on the left) to higher energy (on the right).
C. Frequency.
Rank the different bands of the EM spectrum in order from lower frequency (on the left) to higher frequency (on the right).
D. Relationships.
1. How did the three rankings you completed compare with one another?
PHOTON RACE REPRISE
Ladies and gentlemen! Place your bets! Which colors of light do you think travel fastest through the vacuum of empty space?
1. Rank the wavebands: gamma-ray, x-ray, optical, and radio, according to their speeds.
Now that you have made your predictions, press the “start” button to enjoy the race.
2. Describe how the outcome of the race compares with your initial predictions.
3. Explain how the equation that describes the relationship between a wave’s wavelength and frequency (λ = c / f ) relates to the outcome of the race.
UNITS OF ENERGY
In this activity, you will have a chance to practice converting the units of energy for photons using the conversion factor 1 eV = 1.6 × 10 -19 J. Sometimes astronomers express energy in keV (10 3 eV) or MeV (10 6 eV).
Worked Example:
1. An x-ray photon has an energy of 1 keV. What is its energy in joules?
- Given: \(E\) = 1 keV = 1E3 eV
- Find: Energy in joules
- Concept(s): 1 eV = 1.6E-19 J
- Solution: \(E\) = 1 keV = 1E3eV × (1.6E-19 J / 1 eV) = 1.6E-16 J
1. A gamma-ray photon has an energy of 6.63 x 10 -13 J. What is its energy in MeV?
Show your work:
SPECTRUM PUZZLE
Above the table are several tiles, each with a specific energy, frequency, or wavelength. Drag and drop each tile into the appropriate row and column on the chart of the EM spectrum until all tiles have been placed. If the tile is placed correctly, a green check mark appears.
If you are having difficulty, you may want to refer to previous activities where you ranked the energies, frequencies, and wavelengths of the various bands of the EM spectrum.
Challenge round: Compute the values.
Once you complete part A, blank tiles will automatically appear in the rest of the chart.
Complete the chart by computing the energies, frequencies, and wavelengths for the remaining tiles and entering the values on the tiles:
- Click the “edit” button, which is the vertical bar on the right-hand side of the tile
- Enter the coefficient into the first small box
- Enter the exponent into the second small box
- Select the appropriate units
- Click the “edit” button again to check your answers
Try at least one example of each type to make sure you understand the calculations.
Show your work here:
Dietary Fiber Consumption Reduces Blood Pressure, Prevents Cardiovascular Disease, Review Says
Astronomers detect unusual radio pulses from nearby magnetar, astronomers detect rainbow-like ‘glory’ in atmosphere of wasp-76b.
- Paleontologists Discover New Species of Elasmosaur
- Scientists Create Largest and Most Detailed Bird Family Tree to Date
- Astronomers Discover Faintest Known Milky Way Satellite
- Event Horizon Telescope Observes Twisted Magnetic Field around Milky Way’s Central Black Hole

Do Gamma-Ray Jets Really Achieve Superluminal Speeds?
High-speed particle jets from gamma-ray bursts may exceed the speed of light in surrounding gas clouds, but do so without violating Einstein’s theory of relativity, says a team of astrophysicists from the Michigan Technological University and the College of Charleston.

An artist’s impression of a particle jet emanating from a black hole at the center of a blazar. Image credit: DESY / Science Communication Lab.
Gamma-ray bursts are the most luminous explosions in the Universe.
Most occur when some types of massive stars run out of fuel and collapse to create new black holes. Others happen when two neutron stars, superdense remnants of stellar explosions, merge.
Both kinds of cataclysmic events create jets of particles that move near the speed of light.
Michigan Technological University’s Professor Robert Nemiroff and Professor Jon Hakkila from the College of Charleston propose that these jets could reach superluminal speeds and create the time-reversibility seen in light curves of the gamma-ray bursts.
These proposed jets, however, do not violate the Einstein’s relativity because they only move faster than light does through the jet medium, not faster than light through vacuum.
“A good way to visualize this superluminal motion is to imagine someone on one side of a pond skipping a stone across the water in your direction,” Professor Hakkila said.
“The frequently-hopping stone moves through the air between hops faster than the waves it generates move through water.”
“You would see waves created by each skip of the approaching stone in reverse order, with waves from the most recent skip arriving first and those from the initial skip arriving last.”
“This superluminal blast explanation retains many characteristics of accepted gamma-ray burst jet models.”
“However, our scenario involves Cherenkov radiation , a type of light created by superluminal motion that was not previously thought to be important in generating the light curves of gamma-ray bursts,” Professor Nemiroff added.
“Standard gamma-ray burst models have neglected time-reversible light curve properties,” Professor Hakkila said.
“Superluminal jet motion accounts for these properties while retaining a great many standard model features.”
The team’s paper was published in the Astrophysical Journal .
Jon Hakkila & Robert Nemiroff. 2019. Time-reversed Gamma-Ray Burst Light-curve Characteristics as Transitions between Subluminal and Superluminal Motion. ApJ 883, 70; doi: 10.3847/1538-4357/ab3bdf

Scientists Discuss Physics and Mathematics behind ‘3 Body Problem’

VST Captures Beautiful Emission Nebula: Gum 41

DESI Creates Largest 3D Map of Our Expanding Universe Ever

Hubble Space Telescope Looks at Obscured Spiral Galaxy


- Gamma-rays: what are they?
- " onclick="window.open(this.href,'win2','status=no,toolbar=no,scrollbars=yes,titlebar=no,menubar=no,resizable=yes,width=640,height=480,directories=no,location=no'); return false;" rel="nofollow"> Print
Almost all of our information about the universe beyond our planet comes from the study of electromagnetic radiation. Gamma radiation is part of the electromagnetic spectrum that includes the familiar visible light and radio waves.
However, there are also many differences between gamma-rays and other forms of energy. For example, a gamma-ray photon has one million to one trillion (one million million) times the energy of a photon of visible light. And, unlike photons of radio and visible light, gamma-ray photons cannot penetrate the earth's atmosphere, for they are absorbed by interactions with air molecules ten miles above the Earth's surface. Also, gamma-rays cannot be reflected by mirrors. Thus, normal telescope optics cannot be used to enhance or increase the collection area of a gamma-ray detector.
Because gamma-rays can traverse great distances in space without absorption by intergalactic dust and gas, they can serve as powerful probes of distant regions of the cosmos as well as otherwise obscured regions of our own Milky Way .
Gamma-rays are produced on Earth from the radioactive decay of naturally occurring elements, and oncologists may use such gamma-rays to treat cancer tumors. Particle physicists can produce gamma-rays at accelerators such as Fermilab in Illinois or the Stanford Linear Accelerator in California, where they are used to study the properties of elementary matter. But very-high-energy gamma-rays like those emitted by astronomical bodies do not occur naturally on Earth.
- You are here:

- Atmospheric Cherenkov Technique and VERITAS Technologies
- VERITAS Specifications
- VERITAS Outstanding Contribution Awards
- VERITAS Governance
- VERITAS Code of Conduct
- History & Timeline
- Introduction to VHE Gamma-Ray Astrophysics
- VERITAS Results
- VERITAS Blazar Spectral Information & Light Curves
- VERITAS Blazar Long-term Monitoring
- Mrk 421 Long-term Light Curve
- Theses & dissertations
- Directions & Maps
- FLWO Visitor's Information
- Information for Mountain Users
Current Weather
- All-sky Camera
- How to Collaborate
- Current Associate Members
- Fermi Collaborative Agreement
- Outreach & Education

- April 8, 2024 | Neutrinos Whisper Quantum Gravity Secrets From the South Pole
- April 8, 2024 | Teenage E-Cigarette Use Linked to Frequent Headaches
- April 8, 2024 | The Moon’s Great Inversion: How It Turned Itself Inside Out
- April 8, 2024 | What Came First the Chicken or the Egg? Discovery of Ancient Eggshells Rewrites Chicken History
- April 8, 2024 | Revolutionizing AI With the Power of Energy Efficiency
Beyond Visible Light: Unraveling the Secrets of Gamma-Ray Bursts
By National Research University Higher School of Economics August 1, 2023
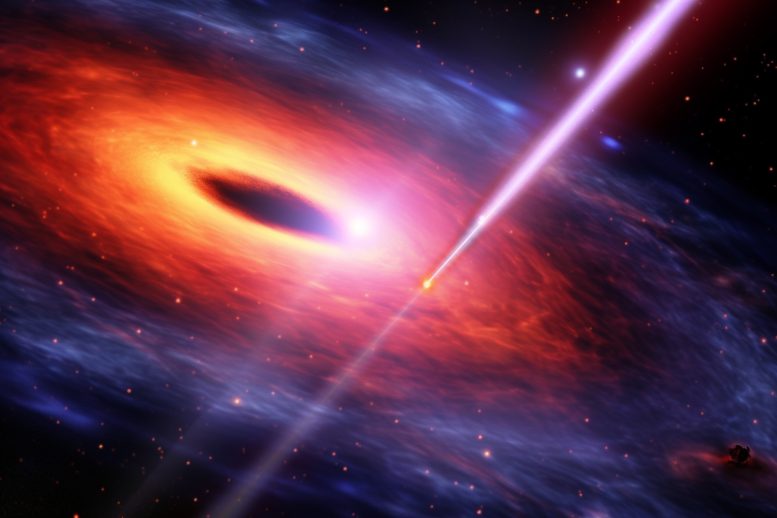
Stars exceeding ten times the mass of the Sun undergo cataclysmic explosions, transforming into black holes, accompanied by brief and unpredictable gamma-ray bursts detectable via space telescopes. Detailed study of these bursts and their associated optical radiation, as seen in 2021’s GRB 210619B, has provided invaluable data about the workings of these star explosions and the conditions they create.
They also discovered a bright optical emission.
Joseph Shklovsky, one of the pioneers of modern astrophysics, posited that a star’s existence is an eternal struggle between two conflicting forces: gravity, which endeavors to shrink the star, and the opposing gas pressure that seeks to disperse it. When the thermonuclear reactions that fuel the star’s core cease, the star loses its capacity to maintain balance and begins to collapse into a singular point.
This scenario, when it occurs to a star whose mass surpasses ten times the mass of our Sun, results in the core’s contraction and the explosive disruption of the outer shell. This leads to an extraordinarily powerful explosion on a galactic scale. This is how the most massive stars transform into black holes.
These explosions are accompanied by an intense burst of gamma radiation — a stream of photons carrying energy millions of times greater than the quanta of visible light we are familiar with.
A gamma-ray burst is an extremely brief — with a duration ranging from a fraction of a second to several hundred seconds — and unpredictable event. There is no way to anticipate the precise location within the skies and the exact timing of a gamma-ray burst. Furthermore, since the Earth’s atmosphere blocks gamma radiation, gamma-ray bursts can only be detected by using space telescopes.
Gamma-ray bursts have been recorded since the late 1960s. For many years, scientists only recorded gamma radiation invisible to the human eye. However, there were suggestions that these bursts might be accompanied by optical radiation that is observable from Earth. Indeed, it was observed for the first time on January 23rd, 1999.
To enable the rapid detection of optical radiation, scientists have developed robotic telescopes which have the capability to gather real-time data directly from the location of the burst. On June 20th, 2021, GRB 210619B, one of the most powerful gamma-ray bursts documented to date was observed using telescopes located in the Czech Republic and Spain, as well as the Russian Mini-MegaTORTORA system owned by Kazan Federal University and located in the North Caucasus. The telescopes began recording the luminous afterglow 28 seconds after the gamma-ray flash. The data acquired simultaneously from three telescopes made it possible to reconstruct the overall shape of the light curve, the slope of the optical spectrum at different times, and the early multiband evolution of the optical radiation.
“We were lucky. First, we observed a fairly bright afterglow. Second, we observed it with a high temporal resolution by capturing frames at a frequent rate. Third, we obtained information about the spectrum of optical radiation. In the Mini-MegaTORTORA system, we were able to conduct observations using a set of optical filters, including blue and visible (yellow-green), simultaneously. In other words, we measured not only the overall brightness but also the brightness displayed in specific individual colors. This is a rare, virtually unique case,” says Anton Biryukov, co-author of the study, Associate Professor of the HSE Faculty of Physics.
The availability of detailed data on radiation across various wavebands, including the optical range, made it possible to determine the physical parameters of the medium associated with the gamma-ray burst in the region where the optical radiation originated. “The extensive dataset obtained by the group enabled us to investigate the inner workings of the gamma-ray burst phenomenon. It was akin to surgically dissecting a gamma-ray burst and peering into its inner mechanism: examining the particles in motion, their energy levels, the density of the surrounding medium, and the characteristics of the magnetic fields involved,” the scientist explained.
The study authors concluded that the luminous phenomenon observed during a gamma-ray burst arises from the motion of high-energy charged particles, which exhibit velocities almost indistinguishable from the speed of light, within a rarefied medium characterized by a powerful magnetic field.
“Gamma-ray bursts are like beacons from the early universe. We record these phenomena at distances spanning several billion light-years. These rare sources provide us with an opportunity to learn about the workings of stars billions of years ago and how their existence ended, to explore the interstellar environment that enveloped them, such as the composition and quantity of interstellar gas, and how it interacted with the stellar ejecta,” explains Biryukov.
But studying gamma-ray bursts does more than expand our knowledge about the most massive distant stars. From a fundamental physics perspective, gamma-ray bursts serve as natural physics laboratories that manifest the most extreme conditions imaginable, encompassing ultra-high energies, velocities, densities, and gravitational forces. It is within these states that scientists can test the physical theories available to humankind.
“Physicists are well aware that the existing fundamental theories describing the world, such as the theory of relativity and quantum mechanics, have their inherent limits of applicability. These limits can only be experimentally determined, and a gamma-ray burst serves as a natural experiment. However, detecting these limits is not an easy task. Continuing observations and striving to comprehensively describe as many similar events as possible is crucial for accumulating an adequate amount of information. But this pursuit aligns well with the natural progression of scientific knowledge,” claims Andrey Biryukov.
Reference: “Exceptionally bright optical emission from a rare and distant gamma-ray burst” by Gor Oganesyan, Sergey Karpov, Om Sharan Salafia, Martin Jelínek, Gregory Beskin, Samuele Ronchini, Biswajit Banerjee, Marica Branchesi, Jan Štrobl, Cyril Polášek, René Hudec, Eugeny Ivanov, Elena Katkova, Alexey Perkov, Anton Biryukov, Nadezhda Lyapsina, Vyacheslav Sasyuk, Martin Mašek, Petr Janeček, Jan Ebr, Jakub Juryšek, Ronan Cunniffe and Michael Prouza, 11 May 2023, Nature Astronomy . DOI: 10.1038/s41550-023-01972-4
More on SciTechDaily
Best view yet of exceptional cosmic explosion – challenges established theory of gamma-ray bursts in the universe, surprising phenomena observed by nasa’s nustar in brightest cosmic explosion ever detected.
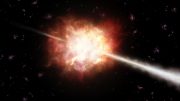
Caught in Afterglow of a Dying Star: Most Powerful Light in the Universe
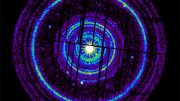
Solar System Hit by Pulse of Intense Radiation – Incredible 1-in-10,000-Year Gamma-Ray Burst
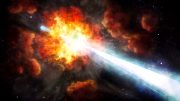
The Brightest Explosion Ever Seen: 1,000x More Energy Than Our Sun Has Emitted Throughout Its 4.5 Billion Year Life
The boat event: brightest gamma-ray burst in history puzzles astronomers worldwide.
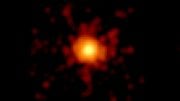
Astronomers View Cosmic Blast GRB 130427A in Unique Detail
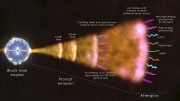
New Era in Gamma-Ray Science with NASA Fermi, Swift Missions
Be the first to comment on "beyond visible light: unraveling the secrets of gamma-ray bursts", leave a comment cancel reply.
Email address is optional. If provided, your email will not be published or shared.
Save my name, email, and website in this browser for the next time I comment.
National Aeronautics and Space Administration
Goddard space flight center, imagine the universe.
- Astronomer's Toolbox
- Cosmic Objects
- Big Questions
- Featured Science
- Observatories
- Scientist Profiles
- You Be the Astrophysicist
- The Cosmic Distance Scale
- Lesson Plans
- Ask an Astrophysicist
- Other Resources
- News #include virtual="/news/newsNav.html"
Educators' Corner
How far how powerful.
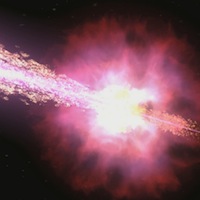
Suggested grade levels : 11-12 Estimated class time : 2 class periods
Lesson plan by Laura Whitlock, Kara Granger, and Padi Boyd
These activities invite you to journey with your students to gamma-ray bursts distant explosions that briefly outshine the rest of the gamma-ray Universe! Your students will follow the same procedures used by today's astronomers to determine how far away and how powerful gamma-ray bursts truly are.
- Students will reinforce their knowledge of basic physics concepts (light, the electromagnetic spectrum) and chemistry concepts (spectral lines, spectroscopy)
- Students will learn about Hubble's Law and how this allows astronomers to measure the distance to faraway objects.
National Education Standards
National science education standards.
This lesson plan addresses the following National Science Education Standards:
- Abilities necessary to do scientific inquiry
- Understandings about scientific inquiry
NS.9-12.2 Physical Science As a result of their activities in grades 9-12, all students should develop an understanding of:
- Structure of atoms
- Conservation of energy and increase in disorder
- Interactions of energy and matter
NS.9-12.4 Earth and Space Science As a result of activities in grades 5-8, all students should develop an understanding of:
- Origin and evolution of the universe
National Council of Teachers of Mathematics
This lesson plan addresses the following educational standards from the National Council of Teachers of Mathematics:
- build new mathematical knowledge through problem solving
- solve problems that arise in mathematics and in other contexts
- recognize and apply mathematics in contexts outside of mathematics
- develop a deeper understanding of very large and very small numbers and of various representations of them
- understand the meaning of equivalent forms of expressions, equations, inequalities, and relations
- use symbolic algebra to represent and explain mathematical relationships
- identify essential quantitative relationships in a situation and determine the class or classes of functions that might model the relationships
- use symbolic expressions, including iterative and recursive forms, to represent relationships arising from various contexts
- make decisions about units and scales that are appropriate for problem situations involving measurement
- use unit analysis to check measurement computations
Prerequisites
- Students should be proficient in Algebra.
- Students should be proficient with the metric system of measurement and units conversions.
- Students should have had an introduction to the electromagnetic spectrum, the concepts of wavelength and frequency.
- tape measure
- single-hole hole punch
- dark orange or dark red paper (at least 7 cm x 7 cm)
- flashlight with a well focused emission of light and light end no more than 5cm in diameter
- graphing calculator or computer software that can perform a power regression on a set of data
- Fall-off of light procedure worksheet (one for each lab group) ( html , Word , PDF )
- Keck Spectrum worksheets (one for each student or each lab group) ( html , Word , PDF )
Introduction
Gamma ray bursts are fast, intense bursts of gamma rays and are the most energetic events in the Universe. They travel clear across the universe from distant galaxies to our detectors in low Earth orbit. But how do we know how far away these objects are?
The activities presented in this lesson plan allow your students to use the same methods as today's astronomers to discover for themselves the distance to a gamma-ray burst. To do this, they will need to review some basic physics concepts about light, learn about redshifted spectra and Hubble's Law, and put all of this information together.
This lesson plan proceeds through the following set of activities:
- Introduce the students to the big idea: what we know from stars and galaxies is all based on what we measure using detectors either on Earth or in orbit. How do we go from this information to an estimate of how far away the objects are, and how much power they are putting out? Let students brainstorm possible paths to these answers.
- Students measure how light falls off over distance. Your class might break up into several groups, whose results could be averaged together. How close did your class come to the actual behavior of one over r-squared?
- Present the information found in Introduction to Hubble's Law and the Doppler Shift. The central, most important concept to get across is that Hubble discovered that the further a galaxy is from Earth, the greater its redshift i.e. the faster it is receding from us. We can turn this idea around: if we measure the redshift of an object we can calculate its distance from Earth.
- Finally, bring all of these concepts together by having your class analyze a Keck Observatory spectrum of a real gamma-ray burst. Students will use real astronomical data to measure the redshift of the gamma ray burst, and then use this information to estimate its distance from Earth. The final step of this activity applies the 1/r 2 fall-off of light, the distance to the object, and the power measured at our detector, to estimate the total power emitted by the gamma ray burst.
Guided Practice: Fall-off of light
Ask students to imagine that they saw a light on a dark night. How would they know how far away that light was? What would they need to know? How would they know the difference between a flashlight that was a few hundred meters away or a headlight a couple kilometers away? They would need to know how bright the original source was.
Astronomers often use an object's "apparent magnitude," or how bright the object appears , coupled with a measure of the object's distance to determine its "absolute magnitude", or how bright the object really is.
Tell your students that they they are astronomers who have collected light from a faraway source. Their detector is in orbit around Earth and has measured a certain amount of energy from this source. Their job is to determine how much energy the source actually emitted. They can assume the source emits energy equally in all directions.
If a light source emits light equally in all directions, what does this mean about the amount of light we will measure in any given square cm as we move further and further away from the light source?
A light source, at the center of this image, emits light equally in all directions. Moving away from the source, that light encompasses a larger and larger surface. This is the basis of the 1-over-r-squared law (or 1/r 2 law).
Think of light as a wave traveling away from the source. At a distance r 1 from the source, all the energy from the light is found on a spherical surface with radius r 1 . The area of such a sphere would be:

As the wave continues to travel away from the source to a distance of r 2 , the light is then all found on a spherical surface whose area is:

Keep in mind that the total amount of light emitted by the source, let's call it L, is constant. What is changing as we move away from the source is the area over which it is spread.
Let us then compare the amount of light per unit area at a distance of r 1 to that at r 2 . At r 1 , the light per unit area, L 1 , is equal to:
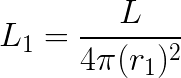
And at r 2 , the light per unit area, L 2 , is equal to:
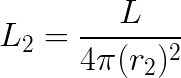
Solving each equation for L gives:
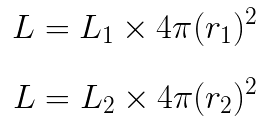
So the amount of light per unit area at L 1 relative to the amount of light per unit area at r 2 is then:
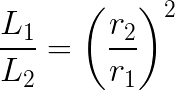
Think about what this means. If r 1 is 5 cm and r 2 is 10 cm, then there is 1/4 as much light per square cm at r 2 as at r 1 . So the distance changed by a factor of 2, but the amount of light per square cm changed by a factor of 4. What if r 1 was 5 and r 2 was 50? How much less light per square cm do you have there?
We say that the intensity, or amount of light per square cm, changes as 1/distance squared (i.e., 1/r 2 ) away from the source. This is just one step for how astronomers determine the distance and power of faraway objects. If we measure X amount of energy per square cm in our detector, then we know that the source must have emitted energy equal to 4 π r 2 times X!
Measuring Light Fall-off
Students will now prove that light really does fall off as 1/r 2 . Below we describe a quick and easy way to do this. If you would rather have your students performa a more rigorous measurement, try the Just How Far is that Star? lesson plan from our Cosmic Times materials.
The steps below are also enumerated on the Fall-off of light procedure worksheet . Have students break up into lab groups. and complete the procedure below.
- dark orange or dark red paper (at least 7 by 7cm)
- flashlight with a well focused emission of light and light end no more than 5 cm in diameter
- graphing calculator or computer with software to perform a power regression on your data
- Find a blank, flat wall to shine a light onto and a table or chair that can stabilize the flashlight for this lab. If you have a nice blank wall near the end of a lab table, you can just put the light on top of the table. You will need to be able to move the light (whether along the table or by moving the chair) at least 1 meter in distance closer to or away from the wall.
- Use the hole punch to punch a hole in the center of the dark orange or dark red paper.
- Tape the paper to the light end of the flashlight, making sure the hole is roughly centered over the light.
- Place the flashlight on the chair or table at least 10 cm from the wall. You can adjust it with papers or books so that it is level (i.e., the light beam shines perpendicular on the wall). Measure the distance from the wall to the hole on the front of the flashlight. Record this distance in your data table.
- Turn the flashlight on. You should see a distinct white circle projected on the wall. The rest of the light emitted outside of this light should be a shade of the colored paper taped to the flashlight.
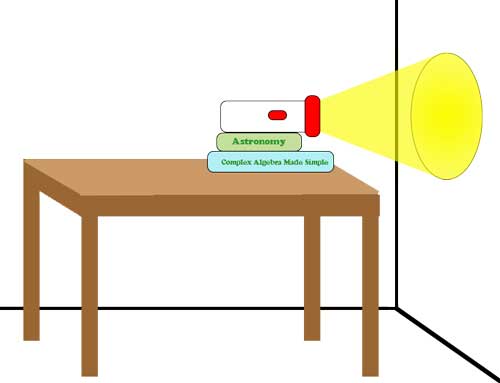
Illustration of the lab set-up. (Credit: NASA/Imagine the Universe)
- Take 3 different measurements of the diameter of the circle of white light on the wall. HINT: Remember, the diameter of a circle is the longest measured chord from one edge of the circle to the other. Therefore, you know you've got the diameter when you hold one end of the tape measure on one side (edge) of the circle and get the largest measured value by moving the other end of the tape measure along the other side.
- Record these values in the data chart. Calculate the mean and record it as well.
- Move the chair with the flashlight (or move along the table) about 20 cm further away from the wall. Measure the distance from the wall to the hole on the front of the flashlight. Record this distance on your data table.
- Repeat steps 6-8 at least two more times.
- Calculate the radius from each mean diameter. Record each calculation in the data table.
- Calculate the area of the circle formed from each calculated radius. Record each calculation in the data table. HINT: Area of a circle equals π r 2 .
- In your graphics calculator, enter the distance from the hole to the wall as one list and the area of each calculated circle as another list.
- Plot the data in the lists as a scatter plot. What kind of relationship do you see?
- Calculate a Power Regression equation on the two lists. What is the equation?
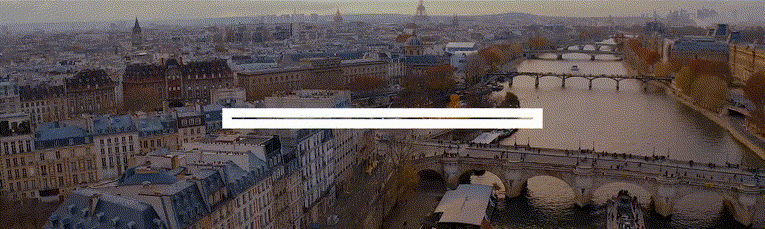
Guided Practice: Hubble's Law and Doppler Shift
During the 1920s and 30s, Edwin Hubble discovered that the Universe is expanding, with galaxies moving away from each other at a velocity given by an expression:

where v represents the galaxy's recessional velocity, d is its distance away from Earth, and H o is a constant of proportionality called Hubble's Constant.
This equation is known as Hubble's Law. The exact value of the Hubble Constant is still somewhat uncertain. Hubble's first determination of it was a value of 550 kilometers per second for every megaparsec in distance (km/sec/Mpc), where a megaparsec is equal to 3 \times 10 6 light-years. Today, it is generally believed to be around 70 km/sec/Mpc. This means that a galaxy 1 Mpc away will be moving away from us at a speed of around 70 km/sec, while another galaxy 100 Mpc away will be receding at 100 times this speed. In essence, the Hubble Constant reflects the rate at which the Universe is expanding.
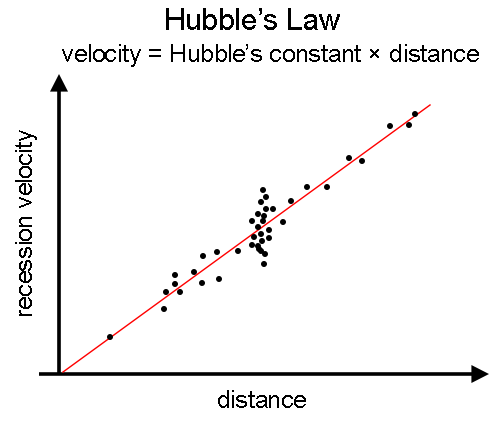
Plot of recession velocity versus distance for galaxies, showing the basis of Hubble's law. The further a galaxy is away, the faster it is receding from us. Therefore, if we know how fast a galaxy is moving, we can determine its distance using this plot.
While there is certainly some scatter of measured observations off the line of best fit for Hubble's Law, it is nevertheless a good way to get a rough idea of how far away a very distant object is from Earth. All we need to do is to be able to determine the object's velocity.
The velocity is relatively easy for us to measure using the Doppler effect, or Doppler shift. An object in motion will have its radiation (i.e. light) shifted in wavelength according to the following formula:
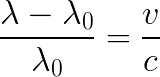
where λ is the measured wavelength, λ 0 is the original (rest) wavelength, v is the speed of the object and c is the speed of light. The quantity on the left side of the equation above is usually called the redshift, and is denoted by the letter "Z".
Wavelengths can be measured in any units angstroms (10 -10 meters), nm, mm, or km. As long as λ - λ 0 and λ are in the same units, they will cancel. The speed of light has a constant value of 300,000 km/sec. So, to determine the velocity of a galaxy (in km/sec) from its spectrum, we simply measure the wavelength shift of a known absorption line and solve for v.
Example : A certain absorption line that is found at 5000 angstroms in the lab is found at 5050 angstroms when analyzing the spectrum of a particular galaxy. We then conclude that this galaxy is moving with a velocity v = (50/5000) * c = 3000 km/sec away from us.
Putting it all together now, if the object is moving away from us at 3000 km/sec, its distance from us (according to the Hubble Law) is:
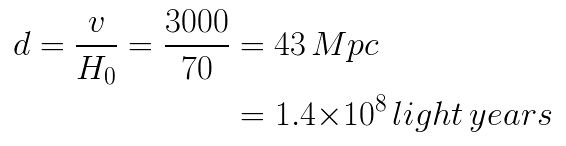
Independent Practice: Putting it all Together
Using optical data of a gamma ray burst afterglow, students will put together all of the information in this lesson to determine the power of a gamma-ray burst. First, students will measure the velocity of the object from the redshifts of its spectral lines. Then they will use Hubble's Law to determine the distance to this object. Finally, they will estimate the power of the gamma ray burst from its measured flux at Earth and its distance from us.
Hand out the Keck Spectrum worksheet to your students and have them work through the problem on the worksheet to determine the power of GRB980703.
Formative assessment and observation should be evident throughout the lesson. The worksheet, final questions during closure or a future quiz may serve as summative assessment.
Direct students to write for five minutes in their journals summarizing the lab and all procedures in this lesson. Encourage students to then share their findings and what they might have written in their journals.
- This experiment was adapted from "Spectroscopy Lab", Chemistry Laboratory Manual, Prentice Hall, 1996, pp. 61-66.
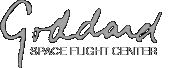
- Project Leader: Dr. Barbara Mattson
- Web Curator: J.D. Myers
- Responsible NASA Official : Dr. Andy Ptak
- Privacy Policy & Important Notices
- Page Last Updated: 18-Oct-2021
Faster-Than-Light Travel Could Explain Mysterious Signals Beaming Through the Cosmos
But don't worry, no laws of physics are being violated.
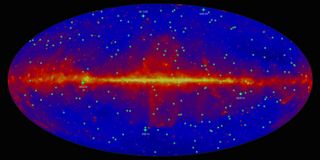
In a distant corner of the universe, something is traveling faster than light.
No, the laws of physics aren't being violated: It's still true that nothing can travel faster than light in the vacuum of empty space. But when light travels through matter , like interstellar gas or a soup of charged particles, it slow downs, meaning other matter might overtake it. And that may explain the weird symmetry in pulses of some of the most energetic light in the universe, called gamma-ray bursts.
Related: 8 Ways You Can See Einstein’s Theory of Relativity in Real Life
These cryptic bursts — bright flashes of gamma-ray light that come from faraway galaxies — form when massive stars collapse or when ultradense neutron stars collide. These cataclysms send speeding jets of hot, charged plasma zooming through space.
But these signals have an odd symmetry , and the reason they do is still a mystery.
A gamma-ray burst doesn't brighten and dim in one steady peak, but instead in a flickering pattern, said Jon Hakkila, an astrophysicist at the College of Charleston in South Carolina.
Hakkila has worked on this puzzle for years. Now, he and a collaborator have a solution: plasma traveling both slower and faster than the speed of light could explain this flickering pattern, as they report in a paper published Sept. 23 in The Astrophysical Journal . If they're right, it may help us understand what's actually producing these gamma-rays.
Sign up for the Live Science daily newsletter now
Get the world’s most fascinating discoveries delivered straight to your inbox.
"I find it a great step forward," that connects the small scale phenomena in the plasma to our large-scale observations, said Dieter Hartmann, an astrophysicist at Clemson University who was not involved in the study.
In the last few years, Hakkila has found that gamma-ray bursts have small fluctuations in brightness on top of their overall brightening and dimming. If you subtract the overarching brightening and dimming, you're left with a series of smaller peaks — one primary peak with smaller peaks in brightness before and after. And this pattern is strangely symmetric. If you "fold" the pattern over at the main peak and stretch one side, the two sides match remarkably well. In other words, the light pattern of a gamma-ray burst's pulse hints at a set of mirrored events.
"Whatever happened on the front side happened on the back side," Hakkila said. "And the events knew to happen in reverse order."
Though astronomers don't know what causes gamma-ray burst emission at the particle scale, they are fairly sure that it happens when jets of plasma traveling near the speed of light interact with surrounding gases. Hakkila had been trying to come up with explanations for how these situations might make symmetric light pulses when he heard from Robert Nemiroff, an astrophysicist at Michigan Technological University.
Nemiroff was studying what happens when an object travels through a surrounding medium faster than the light it emits, called superluminal motion. In previous research, Nemiroff had found that when such an object goes from traveling slower than light to faster than light, or vice versa, this transition can trigger a phenomenon called relativistic image doubling. Nemiroff wondered whether this could account for the symmetric patterns Hakkila found in gamma-ray burst pulses.
So what exactly is "relativistic image doubling?" Imagine a boat creating ripples as it moves across a lake toward the shore. If the boat travels more slowly than the waves it creates, a person standing on the shore will see the boat's ripples hit the shore in the order that the boat created them. But if the boat travels faster than the waves it creates, the boat will overtake the first wave it creates only to create a new ripple in front of that one and so on. In that way, the new ripples created by the boat will reach the shore sooner than the first waves it created. A person standing on the shore will see the ripples hit the shore in a time-reversed order.
The same idea applies to gamma-ray bursts. If the cause of a gamma-ray burst is traveling faster than the light it emits through the gas and matter surrounding it, we would see the emission pattern in reverse chronological order.
Hakkila and Nemiroff reasoned that this could account for half of a gamma-ray burst's symmetric pulse.
But what if the material was first traveling slower than the speed of light, but then accelerated? What if it started fast and then slowed down? In either case, we might see the emission both in chronological order and reverse chronological order right after one another, making a symmetric pulse pattern like the symmetric peaks observed in gamma-ray bursts.
There are still missing pieces to this puzzle. For one, researchers still don't know what's causing these bursts at the microscopic scale. But this proposed model gives researchers one small clue in the hunt to find the ultimate cause of gamma-ray bursts, Hartmann said.
Originally published on Live Science .
- The 7 Silliest Time Travel Concepts in Science Fiction
- The 12 Strangest Objects in the Universe
- The Biggest Unsolved Mysteries in Physics
'You could feel the energy and wonder': Despite clouds, totality wows crowds during solar eclipse in Syracuse
What should you do with your used solar eclipse glasses?
Pet fox with 'deep relationship with the hunter-gatherer society' buried 1,500 years ago in Argentina
Most Popular
By Nicoletta Lanese April 09, 2024
By Stephanie Pappas April 09, 2024
By Melissa Hobson April 09, 2024
By Jamie Carter April 08, 2024
By Ben Turner April 08, 2024
By Emily Cooke April 08, 2024
By Sharmila Kuthunur April 08, 2024
By Brandon Specktor April 08, 2024
By Sascha Pare April 08, 2024
By Andre Luiz Saraiva De Oliveira, Andrew Dzurak April 08, 2024
- 2 Where to get solar eclipse glasses last minute
- 3 How and where to watch the April 8 solar eclipse online for free
- 4 NASA engineers discover why Voyager 1 is sending a stream of gibberish from outside our solar system
- 5 Giant coyote killed in southern Michigan turns out to be a gray wolf — despite the species vanishing from region 100 years ago
- 2 Giant 'toe biter' water bugs discovered in Cyprus for the 1st time
- 3 When is the next total solar eclipse after 2024 in North America?
- 4 Watch live! The total solar eclipse has begun over North America.
- 5 8,200-year-old campsite of 'Paleo-Archaic' peoples discovered on US Air Force base in New Mexico
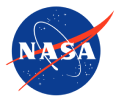
NASA’s Fermi Mission Nets 300 Gamma-Ray Pulsars … and Counting
A new catalog produced by a French-led international team of astronomers shows that NASA’s Fermi Gamma-ray Space Telescope has discovered 294 gamma-ray-emitting pulsars, while another 34 suspects await confirmation. This is 27 times the number known before the mission launched in 2008.
“Pulsars touch on a wide range of astrophysics research, from cosmic rays and stellar evolution to the search for gravitational waves and dark matter,” said study coordinator David Smith, research director at the Bordeaux Astrophysics Laboratory in Gironde, France, which is part of CNRS (the French National Center for Scientific Research). “This new catalog compiles full information on all known gamma-ray pulsars in an effort to promote new avenues of exploration.”
The catalog was published on Monday, Nov. 27, in The Astrophysical Journal Supplement.
Pulsars are a type of neutron star, the city-sized leftover of a massive sun that has exploded as a supernova. Neutron stars, containing more mass than our Sun in a ball less than 17 miles wide, represent the densest matter astronomers can study directly . They possess strong magnetic fields, produce streams of energetic particles, and spin quickly – 716 times a second for the fastest known. Pulsars, in addition, emit narrow beams of energy that swing lighthouse-like through space as the objects rotate. When one of these beams sweeps past Earth, astronomers detect a pulse of emission.
The new catalog represents the work of 170 scientists across the globe. A dozen radio telescopes carry out regular monitoring of thousands of pulsars, and radio astronomers search for new pulsars within gamma-ray sources discovered by Fermi. Other researchers have teased out gamma-ray pulsars that have no radio counterparts through millions of hours of computer calculation, a process called a blind search.
More than 15 years after its launch, Fermi remains an incredible discovery machine, and pulsars and their neutron star kin are leading the way.
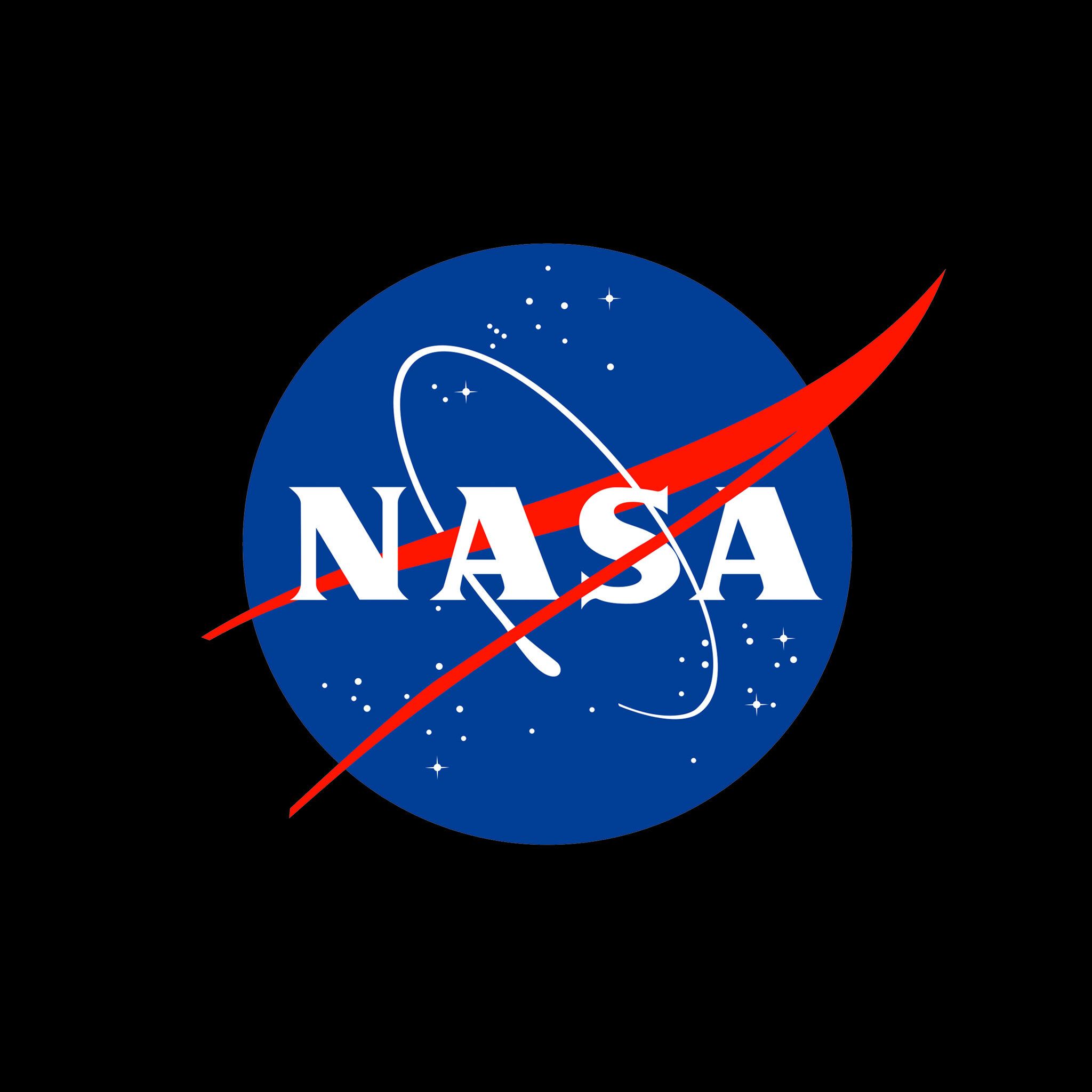
Elizabeth Hays
Fermi Project Scientist
Of the 3,400 pulsars known, most of them observed via radio waves and located within our Milky Way galaxy, only about 10% also pulse in gamma rays, the highest-energy form of light. Visible light has energies between 2 and 3 electron volts. Fermi’s Large Area Telescope can detect gamma rays with billions of times this energy, and other facilities have observed emission thousands of times greater still from the nearby Vela pulsar , the brightest persistent source in the sky for Fermi.
The Vela pulsar and its famous sibling in the Crab Nebula are young, solitary objects, formed about 11,000 and 970 years ago, respectively. Their emissions arise as their magnetic fields spin through space, but this also gradually slows their rotation. The younger Crab pulsar spins nearly 30 times a second, while Vela clocks in about a third as fast.
The Old and the Restless
Paradoxically, though, pulsars that are thousands of times older spin much faster. One example of these so-called millisecond pulsars (MSPs) is J1824-2452A. It whirls around 328 times a second and, with an age of about 30 million years, ranks among the youngest MSPs known.
Thanks to a great combination of gamma-ray brightness and smooth spin slowdown, the MSP J1231-1411 is an ideal “timer” for use in gravitational wave searches. By monitoring a collection of stable MSPs, astronomers hope to link timing changes to passing low-frequency gravitational waves – ripples in space-time – that cannot be detected by current gravitational observatories. It was discovered in one of the first radio searches targeting Fermi gamma-ray sources not associated with any known counterpart at other wavelengths, a technique that turned out to be exceptionally successful.
"Before Fermi, we didn't know if MSPs would be visible at high energies, but it turns out they mostly radiate in gamma rays and now make up fully half of our catalog," said co-author Lucas Guillemot, an associate astronomer at the Laboratory of Physics and Chemistry of the Environment and Space and the University of Orleans, France.
Along Come the Spiders
The presence of MSPs in binary systems offers a clue to understanding the age-spin paradox. Left to itself, a pulsar’s emissions slow it down, and with slower spin its emissions dim. But if closely paired with a normal star, the pulsar can pull a stream of matter from its companion that, over time, can spin up the pulsar.
“Spider” systems offer a glimpse of what happens next. They’re classified as redbacks or black widows – named for spiders known for consuming their mates. Black widows have light companions (less than about 5% of the Sun's mass), while redbacks have heavier partners. As the pulsar spins up, its emissions and particle outflows become so invigorated that – through processes still poorly understood – it heats and slowly evaporates its companion. The most energetic spiders may fully evaporate their partners, leaving only an isolated MSP behind.
J1555-2908 is a black widow with a surprise – its gravitational web may have ensnared a passing planet . An analysis of 12 years of Fermi data reveals long-term spin variations much larger than those seen in other MSPs. “We think a model incorporating the planet as a third body in a wide orbit around the pulsar and its companion describes the changes a little better than other explanations, but we need a few more years of Fermi observations to confirm it,” said co-author Colin Clark, a research group leader at the Max Planck Institute for Gravitational Physics in Hannover, Germany.
Other curious binaries include the so-called transitional pulsars, such as J1023+0038, the first identified. An erratic stream of gas flowing from the companion to the neutron star may surge, suddenly forming a disk around the pulsar that can persist for years. The disk shines brightly in optical light, X-rays, and gamma rays, but pulses become undetectable. When the disk again vanishes, so does the high-energy light and the pulses return.
Some pulsars don’t require a partner to switch things up. J2021+4026, a young, isolated pulsar located about 4,900 light-years away, underwent a puzzling “mode change” in 2011, dimming its gamma rays over about a week and then, years later, slowly returning to its original brightness. Similar behavior had been seen in some radio pulsars, but this was a first in gamma rays. Astronomers suspect the event may have been triggered by crustal cracks that temporarily changed the pulsar‘s magnetic field.
Farther afield, Fermi discovered the first gamma-ray pulsar in another galaxy , the neighboring Large Magellanic Cloud, in 2015. And in 2021, astronomers announced the discovery of a giant gamma-ray flare from a different type of neutron star (called a magnetar) located in the Sculptor galaxy, about 11.4 million light-years away.
“More than 15 years after its launch, Fermi remains an incredible discovery machine, and pulsars and their neutron star kin are leading the way,” said Elizabeth Hays, the mission’s project scientist at NASA’s Goddard Space Flight Center in Greenbelt, Maryland.
Explore the Fermi gamma-ray pulsar catalog on WorldWide Telescope
Max Planck Institute release
U.S. Naval Research Laboratory release
French Atomic Energy Commission release
By Francis Reddy NASA’s Goddard Space Flight Center , Greenbelt, Md.
Media contact: Claire Andreoli [email protected] NASA’s Goddard Space Flight Center, Greenbelt, Md. (301) 286-1940
Related Terms
- Astrophysics
- Binary Stars
- Fermi Gamma-Ray Space Telescope
- Goddard Space Flight Center
- Neutron Stars
- The Universe
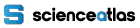
- Planet Earth
- Strange News
Faster-Than-Light Particles Emit Superbright Gamma Sun rays that Circle Pulsars
Table of Contents:
China’s enormous Radio Telescope is already picking up mysterious signals – The mysterious Fast Radio Bursts (FRBs) are energetic radio signals that are much louder than pulsars despite being much further away.
The Five-hundred-meter Aperture Spherical Radio Telescope (FAST), a project that the Chinese government first began planning in 2008, is now fully operational. By the end of September 2019, the $171 million USD (1. 2 billion CNY) FAST project will undergo a last review process, which the Chinese government anticipates will give the go signal to begin studying the skies.
Video advice: NASA
A pulsar is a neutron star that emits beams of radiation that sweep through Earth’s line of sight. Like a black hole, it is an endpoint to stellar evolution. The \”pulses\” of high-energy radiation we see from a pulsar are due to a misalignment of the neutron star’s rotation axis and its magnetic axis. Pulsars seem to pulse from our perspective because the rotation of the neutron star causes the beam of radiation generated within the magnetic field to sweep in and out of our line of sight with a regular period, somewhat like the beam of light from a lighthouse. The stream of light is, in reality, continuous, but to a distant observer, it seems to wink on and off at regular intervals.

Particles travel faster than light in the warped vacuum surrounding pulsars.
Yasemin is a staff writer at Live Science, covering health, neuroscience and biology. Her work has appeared in Scientific American, Science and the San Jose Mercury News. She has a bachelor’s degree in biomedical engineering from the University of Connecticut and a graduate certificate in science communication from the University of California, Santa Cruz.
Charged particles travel faster than light through the quantum vacuum of space that surrounds pulsars. As these electrons and protons fly by pulsars, they create the ultrabright gamma-ray flashes emitted by the rapidly twirling neutron stars, new research reveals. These gamma-rays, called Cherenkov emissions, are also found in powerful particle accelerators on Earth, such as the Large Hadron Collider near Geneva, Switzerland. The rays are also the source of the bluish-white glow in the waters of a nuclear reactor. (Wacky Physics: The Coolest Little Particles in Nature)But until now, no one thought that pulsar emissions consisted of Cherenkov radiation. That’s in part because of Albert Einstein’s famous theory of relativity, which holds that nothing can travel faster than light in a vacuum. Because of those propositions, scientists previously thought that Cherenkov emissions couldn’t happen in the quantum vacuum of space surrounding pulsars. That area is mostly devoid of matter but home to ghostly quantum particles that flicker in and out of existence.
Posted in r/EcoInternet by u/EcoInternetNewsfeed • 1 point and 0 comments.
limit my search to r/EcoInternetuse the following search parameters to narrow your results:subreddit:subredditfind submissions in “subreddit”author:usernamefind submissions by “username”site:example. comfind submissions from “example. com”url:textsearch for “text” in urlselftext:textsearch for “text” in self post contentsself:yes (or self:no)include (or exclude) self postsnsfw:yes (or nsfw:no)include (or exclude) results marked as NSFWe. g. subreddit:aww site:imgur. com dogsee the search faq for details. advanced search: by author, subreddit. . .
Flipboard – renaissancegeek – space.com/quantum-vacuum-gamma-rays.html.
space. com/quantum-vacuum-gamma-rays. html51 likes•115 sharesrenaissancegeek • 1001dRead more on flipboard. comMagazinerenaissancegeek flipped this story into Things that make you say hmmm. . . 🤔•1001dJoin the flipboard communityDiscover, collect, and share stories for all your interests.
astro101 fifnal Flashcards
Start studying astro101 fifnal. Learn vocabulary, terms, and more with flashcards, games, and other study tools.
- Terms in this set (61)
- Astronomy Cosmology Quiz, Astronomy Black Holes Qu…
- Physics Test on Tuesday
- Chapter 18 : Astronomy Reading Quiz
- Finals Notes (Ch 18 – 23)
- PTCB 2 – Angina, Anxiety, Asthma, Bacterial Infect…
- Chemistry Unit 6- Atom, Atomic Theory, Nuclear Che…
- HP SEC4: Electromagnetic Waves
- Physiology Lab Midterm
Upgrade to remove adsOnly RUB 2,325/yearSTUDYFlashcardsLearnWriteSpellTestPLAYMatchGravityTerms in this set (61)magnetars vs neutron star differencesmuch more magnetism, slower rotation. . . bursts of xrays/gamma because of magnetic field decay. active for a very short time, 10k years. apparently considered apulsarneutron star emitting beam(s) of electromagnetic radiation. radiation can only be viewed when pointed at earth. similar to lighthouse only being visible when light is pointed at you. novae that repeat are. . . the least brilliant/violentexplosions of xrays on magnetars are caused byruptures of magnetism on the surface. explosions come from cracks on the surface. the instability strip is where. . . stars that vary in brightness sit. RR Lyrae where it intersects the horizontal strip, Classic Cepheids where it intersects the supergiantsChandrasekhar Limit is. . . The critical mass limit for a white dwarf, 1. 4 solar masses. After this point, the star begins to supernova. kilonovawhen two neutron stars combine, exceeding mass max limit and thus forming a black hole.
Video advice: Tachyon: A faster than light particle
A tachyon or tachyonic particle is a hypothetical particle that always travels faster than light. Most physicists believe that faster-than-light particles cannot exist because they are not consistent with the known laws of physics.

Which form of radiation is the fastest?
Photons travel in harmonic waves at the fastest speed possible in the universe: 186,282 miles per second (299,792,458 meters per second) in a vacuum, also known as the speed of light.
Beta particles have a mass which is half of one thousandth of the mass of a proton and carry either a single negative (electron) or positive (positron) charge. As they have a small mass and can be released with high energy, they can reach relativistic speeds (close to the speed of light).
Which form of radiation is the fastest? What is the speed of a gamma ray? What is the speed of beta rays? Which Ray travels the farthest? Which is faster Alpha or beta? What is the speed of alpha particles? How fast do alpha particles travel? Are gamma rays the fastest? What is the speed of Alpha? How fast do beta particles travel? Is alpha radiation the slowest? Are gamma rays faster than beta? What is the speed of Alpha Beta Beta and gamma particles? What are the properties of alpha beta and gamma rays? What is the mass of a Beta Ray? What are alpha rays?
Does Gamma Travel Faster Than Alpha
Gamma particles travel at the speed of light, they are simply E.M radiation. Beta and alpha particles van both travel at any speed. What is faster gamma or alpha? Alpha.
Microwave is a color of light, too low-energy for you to see, lower-energy even than infrared, but higher-energy than radio. Microwave ovens produce large amounts of this light. Because of the size of the light waves (micrometers, hence the term microwave), they are capable of spinning water molecules as they go past.
What is faster gamma or alpha? Does gamma travel the fastest? Which radiation travels the fastest? Which Ray is fastest? How fast do gamma particles travel? What is the speed of alpha particles? Do gamma waves travel faster than radio waves? Can gamma rays travel faster than light? Which travels faster gamma or radio? Which travels faster through a vacuum infrared or gamma? Which wave travels at the greatest speed? Which among the electromagnetic waves travels fastest slowest? Is alpha radiation the slowest? What is the speed of gamma ray? Does radiation travel faster than the speed of light? What exceeds the speed of light? What can stop gamma particles? Why do alpha particles travel short distances? Which radiation travels at the speed of light? Are jets faster than light? Do all waves travel at the same speed? Are there waves smaller than gamma rays? Are microwaves light?
Particles Moving Faster than Light: Create Super Bright Gamma Rays Circling Pulsars
A new research revealed charged particles travel faster than light through the quantum vacuum of space that surrounds these pulsars. As these electrons and protons fly by pulsars, they create the ultra-bright gamma-ray flashes emitted by the rapidly twirling neutron stars. So, are they the long sought particles that violate Einstein’s law that says nothing can move faster than light? According to co-author Dino Jaroszynski, a professor of physics at the University of Strathclyde in Scotland they do not. Pulsars create crushingly strong electromagnetic fields in the quantum vacuum surrounding the stars. These fields warp, or polarize, the vacuum, essentially creating speed bumps that slow down light particles, Jaroszynski told Live Science. Meanwhile, charged particles such as protons and electrons zoom through these fields, racing past light.
The Hunt for High-Energy Photons Takes Place From a Mountaintop in Mexico
A new telescope built from water tanks might help answer some of the biggest questions in astronomy.
Completed in March 2015, the observatory just recently released its first year of data —a map of the sky revealing about 40 super-bright sources, many from within our Milky Way galaxy. “These are not run-of-the-mill stars,” said physicist Brenda Dingus of Los Alamos National Laboratory, spokesperson for HAWC.
The air over your head is alive with invisible showers. Powerful particles from space are constantly hitting the atmosphere above you, generating a subatomic cascade that shoots downward at near light speed. Figuring out where these downpours originate and what they tell us about the universe is the job of the High-Altitude Water Cherenkov (HAWC) observatory a telescope consisting of 300 giant tanks of purified water placed near the summit of the Sierra Negra volcano in Mexico.
Video advice: 3 Things ‘Faster Than Light’
These 3 things go \”faster\” than the speed of light. How’s that even possible?

Are gamma rays faster than light?
Light of any wavelength, from picometer-wavelength gamma-rays to radio waves more than a trillion times longer , all move at the speed of light in a vacuum.
Do gamma ray bursts travel faster than light?
Cosmic ray bursts produce the most-powerful blasts witnessed since the big bang. New research shows jets formed by these events could travel faster than their local speed of light.
Which particle emits gamma rays?
Gamma rays (γ) are weightless packets of energy called photons . Unlike alpha and beta particles, which have both energy and mass, gamma rays are pure energy. Gamma rays are similar to visible light, but have much higher energy. Gamma rays are often emitted along with alpha or beta particles during radioactive decay.
Does light emit gamma rays?
Gamma-rays are the most energetic form of light and are produced by the hottest regions of the universe.
What is the speed of gamma?
The Gamma rays ( γ- Rays) are also part of the vast EM radiation, so they also travels with the speed of Light c (3 x 10^8 m/s) .
Related Articles:
- Faster-Than-Light Particles? Not Too Fast, New Test States
- Response to Shocking ‘Faster-Than-Light’ Particles Expected Soon
- What exactly are gamma sun rays?
- Astronomers Solve the Mystery of Where Gamma Sun rays Originate
- VERITAS Detects Gamma Sun rays from Distant Universe PKS 1441+25
- A Volcano-Covered Exomoon May Circle a Planet 500 Light-Years Away
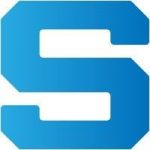
Science Journalist
Science atlas, our goal is to spark the curiosity that exists in all of us. We invite readers to visit us daily, explore topics of interest, and gain new perspectives along the way.
You may also like
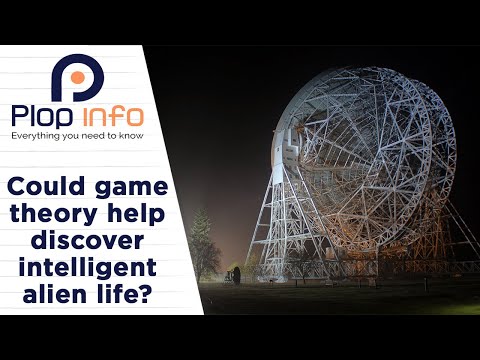
Using Game Theory to assist Uncover Intelligent Alien Existence
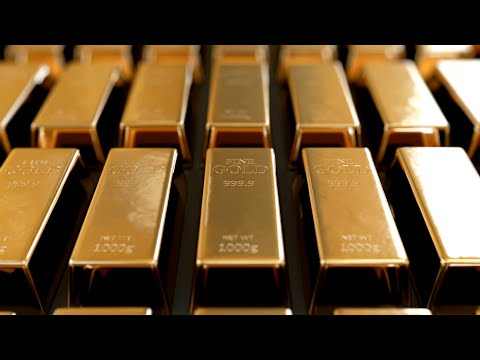
There’s an excessive amount of gold within the world. Nobody knows where it originated from
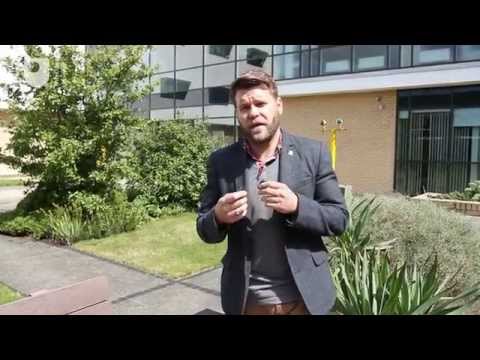
Chemistry of Existence: Key Foundation for Organic Molecules Discovered in Meteorites
Add comment, cancel reply.
Your email address will not be published. Required fields are marked *
Save my name, email, and website in this browser for the next time I comment.
Recent discoveries

Why Did My Commission Robotics App Quit Working
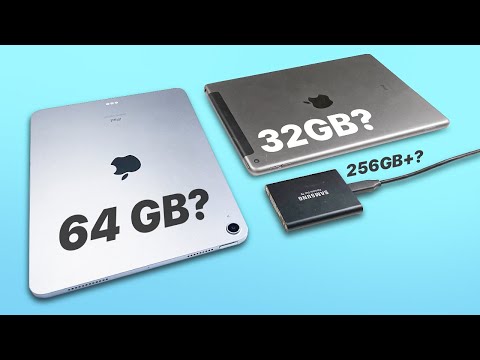
How Much Space Does Ipados Take

What Middle Earth Race Are You
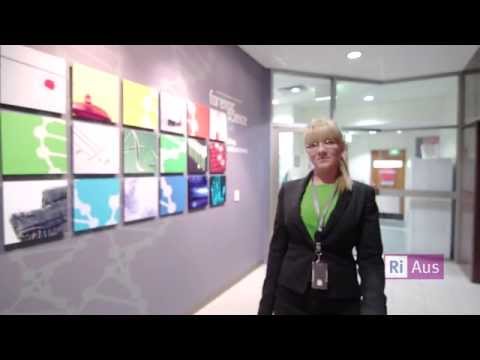
How To Become A Forensic Scientist With A Biology Degree
- Animals 3041
- Astronomy 8
- Biology 2281
- Chemistry 482
- Culture 1333
- Health 8466
- History 2152
- Physics 913
- Planet Earth 3239
- Science 2158
- Strange News 1230
- Technology 3625
Random fact
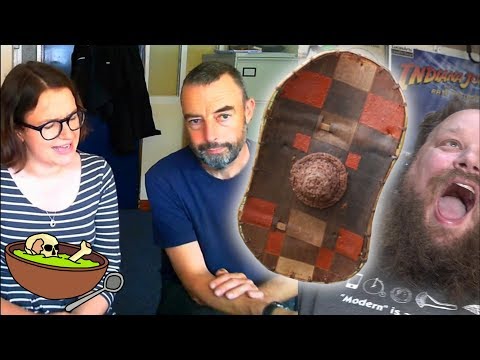
New Iron-Age Shield Made from Bark Discovered in Leicester
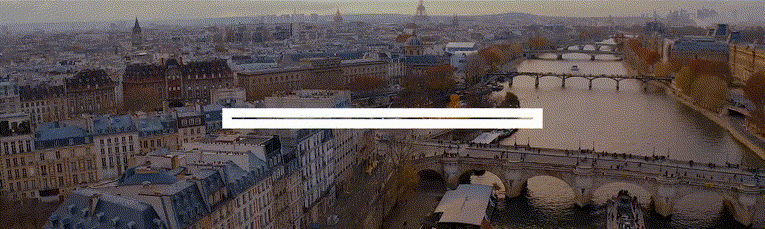
IMAGES
VIDEO
COMMENTS
But last year, researchers found events in some gamma-ray burst pulses that seemed to repeat themselves as though they were going backwards in time. Now, new research suggests a potential answer for what might be causing this time reversibility effect. If waves within the relativistic jets that produce gamma-ray bursts travel faster than light ...
Gamma rays have wavelengths shorter than 10^-11 meters and frequencies above 30 x 10^18 hertz. The European Space Agency describes how gamma-ray photons have energies in excess of 100,000 ...
Light of any wavelength, from picometer-wavelength gamma-rays to radio waves more than a trillion times longer, all move at the speed of light in a vacuum. The frequency of any photon is equal to ...
Astrophysicists propose that gamma-ray bursts may exceed the speed of light. The superluminal jets may also be responsible for time-reversibility. The finding doesn't go against Einstein's theory ...
Gamma-rays—The highest energy light is called gamma-rays. At low energies, gamma-rays merge into x-rays. In principle, there is no upper limit to gamma-ray energies; their energies could go to higher and higher values. ... Place your bets! Which colors of light do you think travel fastest through the vacuum of empty space? 1. Rank the ...
Gamma rays have the smallest wavelengths and the most energy of any wave in the electromagnetic spectrum. They are produced by the hottest and most energetic objects in the universe, such as neutron stars and pulsars, supernova explosions, and regions around black holes. On Earth, gamma waves are generated by nuclear explosions, lightning, and ...
The Crab Nebula, a source of gamma rays. (NASA/ESA/J. Hester/A. Loll/Arizona State University) Physicists have conducted the most high-energy test of the speed of light yet, and found that it is still constant, everywhere in the Universe, even in gamma rays spewed out of sources such as exploding stars. This means that, even at the highest ...
Light of any wavelength, from picometer-wavelength gamma-rays to radio waves more than a trillion times longer, all move at the speed of light in a vacuum. The frequency of any photon is equal to ...
Gamma rays are a form of light. All light travels in waves and is classified according to its wavelength, the distance between its waves. The universe produces a broad range of light, only a fraction of which is visible to our eyes. Other types of nonvisible light include x-rays, ultraviolet light, infrared radiation, and radio waves.
Michigan Technological University's Professor Robert Nemiroff and Professor Jon Hakkila from the College of Charleston propose that these jets could reach superluminal speeds and create the time-reversibility seen in light curves of the gamma-ray bursts. These proposed jets, however, do not violate the Einstein's relativity because they ...
Does a radio wave or gamma radiation have a faster speed? I know that all light travels at $\pu{3E8 m s-1}$, but does that include these forms of electromagnetic radiation? Or do radio waves travel at $700~\mathrm{nm}$ and gamma rays $400~\mathrm{nm}$?
These events, called terrestrial gamma-ray flashes, last less than a millisecond and produce gamma rays with tens of millions of times the energy of visible light. Thunderstorms form when warm, damp air near the ground starts to rise and encounters colder air. As the warm air rises, moisture condenses into water droplets.
Gamma-rays coming from space are mostly absorbed by the Earth's atmosphere.So gamma-ray astronomy could not develop until it was possible to get our detectors above all or most of the atmosphere, using balloons or spacecraft. The first gamma-ray telescope carried into orbit, on the Explorer 11 satellite in 1961, picked up fewer than 100 cosmic gamma-ray photons.
Gamma-ray photons have many properties similar to photons of visible light and radio waves: they travel in straight lines; they move at the speed of light; and they are not affected by magnetic or electric fields in space. However, there are also many differences between gamma-rays and other forms of energy. For example, a gamma-ray photon has ...
There is no way to anticipate the precise location within the skies and the exact timing of a gamma-ray burst. Furthermore, since the Earth's atmosphere blocks gamma radiation, gamma-ray bursts can only be detected by using space telescopes. Gamma-ray bursts have been recorded since the late 1960s.
Now, recent research suggests a potential answer for what might be causing this time reversibility effect. If waves within the relativistic jets that produce gamma-ray bursts travel faster than light - at 'superluminal' speeds - one of the effects could be time reversibility. Such speeding waves could actually be possible.
Gamma ray bursts are fast, intense bursts of gamma rays and are the most energetic events in the Universe. They travel clear across the universe from distant galaxies to our detectors in low Earth orbit. But how do we know how far away these objects are?
The same idea applies to gamma-ray bursts. If the cause of a gamma-ray burst is traveling faster than the light it emits through the gas and matter surrounding it, we would see the emission ...
Travel distance depends on the type of radiation, as does the ability to penetrate other materials. Alpha and beta particles do not travel far at all, and they are easily blocked. By contrast, gamma rays , x-rays , and neutrons travel a significant distance and are much more difficult to block (particularly for large radioactive sources).
How fast do gamma rays travel? Gamma rays travel at the speed of light, which is approximately 299,792,458 meters per second in a vacuum. 2. Can anything travel faster than gamma rays? According to the theory of relativity, nothing can travel faster than the speed of light, including gamma rays. This is considered a fundamental physical limitation.
So, this is really not something worth worrying about. Gamma Rays - Most energetic end of the electromagnetic spectrum, therefore it travels like every other light particle at 300.000 km/s. It's traveling at the speed of light, so we wouldn't see it coming. oh you know, ray as in a ray of light, and gamma as in gamma radiation - light.
Details. A new catalog produced by a French-led international team of astronomers shows that NASA's Fermi Gamma-ray Space Telescope has discovered 294 gamma-ray-emitting pulsars, while another 34 suspects await confirmation. This is 27 times the number known before the mission launched in 2008.
Which form of radiation is the fastest? Photons travel in harmonic waves at the fastest speed possible in the universe: 186,282 miles per second (299,792,458 meters per second) in a vacuum, also known as the speed of light.