
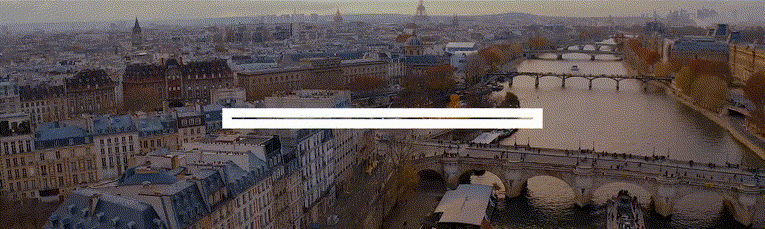
Is Time Travel Possible?
We all travel in time! We travel one year in time between birthdays, for example. And we are all traveling in time at approximately the same speed: 1 second per second.
We typically experience time at one second per second. Credit: NASA/JPL-Caltech
NASA's space telescopes also give us a way to look back in time. Telescopes help us see stars and galaxies that are very far away . It takes a long time for the light from faraway galaxies to reach us. So, when we look into the sky with a telescope, we are seeing what those stars and galaxies looked like a very long time ago.
However, when we think of the phrase "time travel," we are usually thinking of traveling faster than 1 second per second. That kind of time travel sounds like something you'd only see in movies or science fiction books. Could it be real? Science says yes!
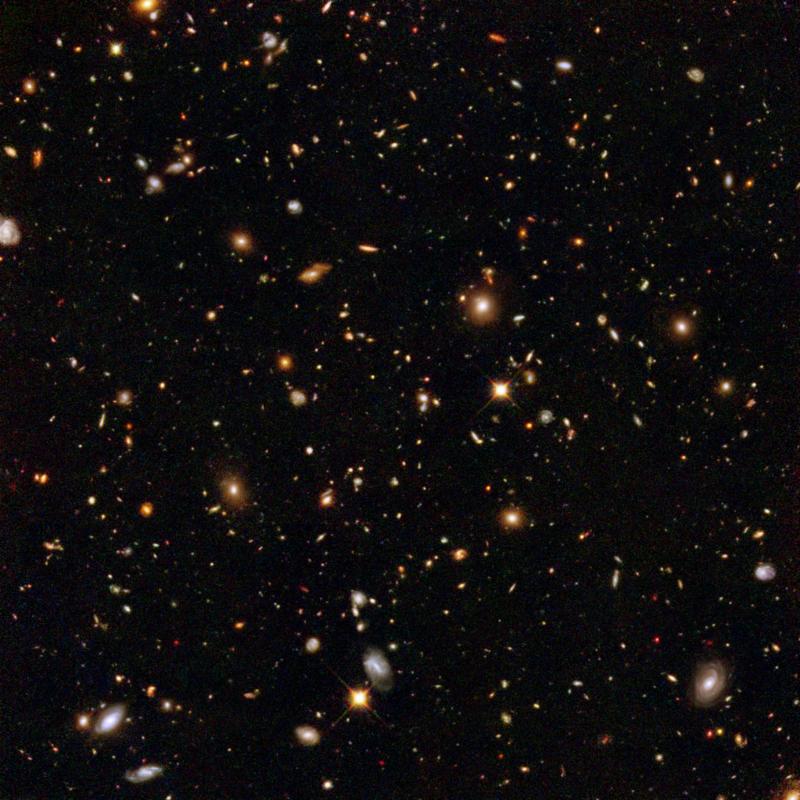
This image from the Hubble Space Telescope shows galaxies that are very far away as they existed a very long time ago. Credit: NASA, ESA and R. Thompson (Univ. Arizona)
How do we know that time travel is possible?
More than 100 years ago, a famous scientist named Albert Einstein came up with an idea about how time works. He called it relativity. This theory says that time and space are linked together. Einstein also said our universe has a speed limit: nothing can travel faster than the speed of light (186,000 miles per second).
Einstein's theory of relativity says that space and time are linked together. Credit: NASA/JPL-Caltech
What does this mean for time travel? Well, according to this theory, the faster you travel, the slower you experience time. Scientists have done some experiments to show that this is true.
For example, there was an experiment that used two clocks set to the exact same time. One clock stayed on Earth, while the other flew in an airplane (going in the same direction Earth rotates).
After the airplane flew around the world, scientists compared the two clocks. The clock on the fast-moving airplane was slightly behind the clock on the ground. So, the clock on the airplane was traveling slightly slower in time than 1 second per second.
Credit: NASA/JPL-Caltech
Can we use time travel in everyday life?
We can't use a time machine to travel hundreds of years into the past or future. That kind of time travel only happens in books and movies. But the math of time travel does affect the things we use every day.
For example, we use GPS satellites to help us figure out how to get to new places. (Check out our video about how GPS satellites work .) NASA scientists also use a high-accuracy version of GPS to keep track of where satellites are in space. But did you know that GPS relies on time-travel calculations to help you get around town?
GPS satellites orbit around Earth very quickly at about 8,700 miles (14,000 kilometers) per hour. This slows down GPS satellite clocks by a small fraction of a second (similar to the airplane example above).
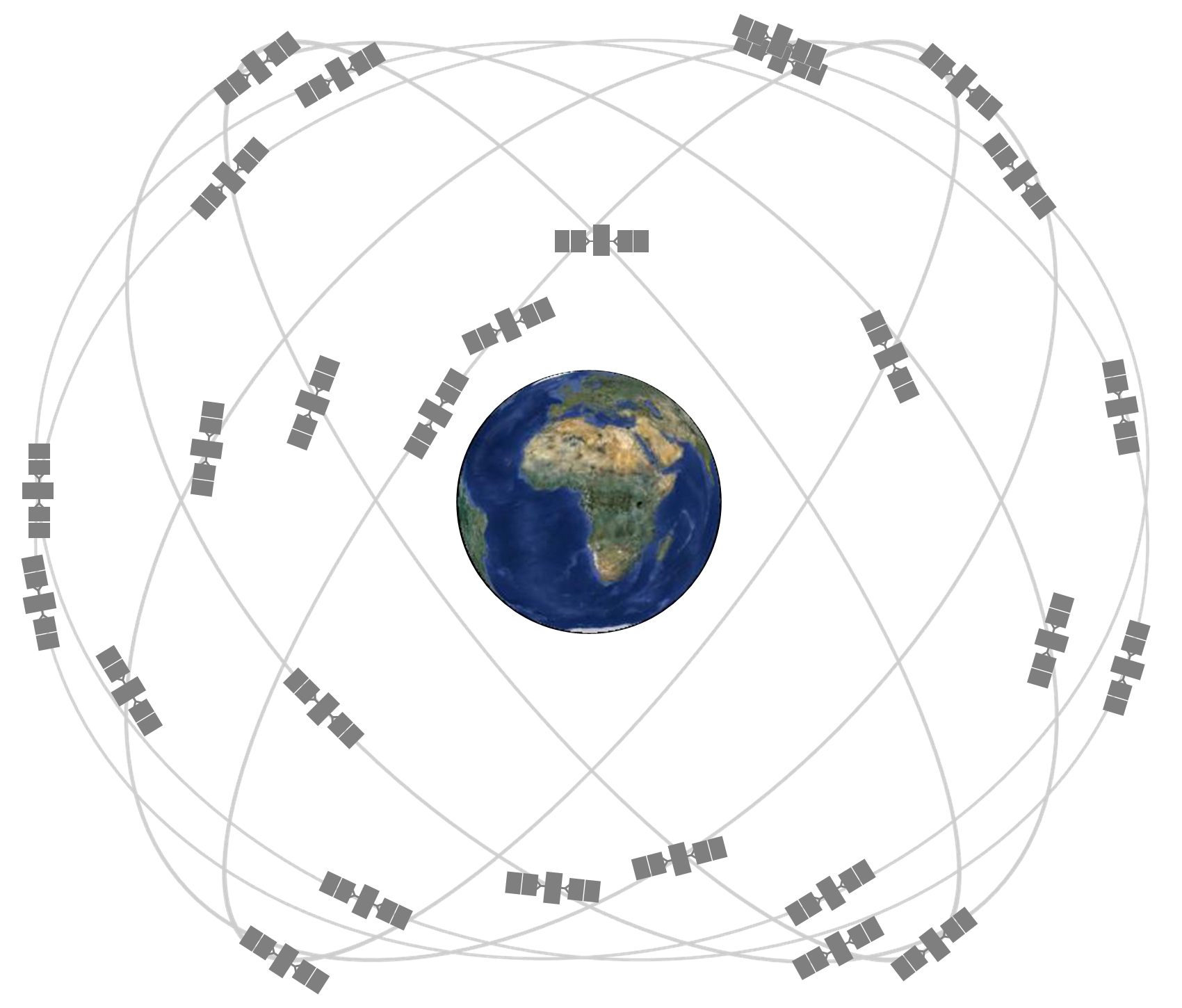
GPS satellites orbit around Earth at about 8,700 miles (14,000 kilometers) per hour. Credit: GPS.gov
However, the satellites are also orbiting Earth about 12,550 miles (20,200 km) above the surface. This actually speeds up GPS satellite clocks by a slighter larger fraction of a second.
Here's how: Einstein's theory also says that gravity curves space and time, causing the passage of time to slow down. High up where the satellites orbit, Earth's gravity is much weaker. This causes the clocks on GPS satellites to run faster than clocks on the ground.
The combined result is that the clocks on GPS satellites experience time at a rate slightly faster than 1 second per second. Luckily, scientists can use math to correct these differences in time.
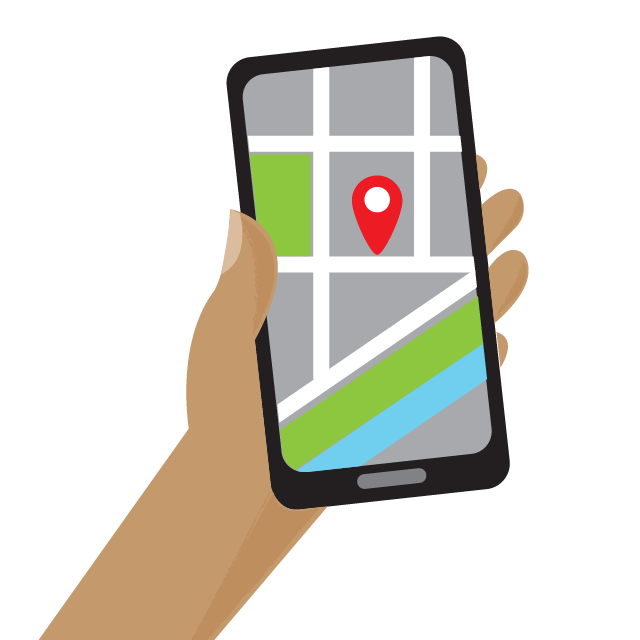
If scientists didn't correct the GPS clocks, there would be big problems. GPS satellites wouldn't be able to correctly calculate their position or yours. The errors would add up to a few miles each day, which is a big deal. GPS maps might think your home is nowhere near where it actually is!
In Summary:
Yes, time travel is indeed a real thing. But it's not quite what you've probably seen in the movies. Under certain conditions, it is possible to experience time passing at a different rate than 1 second per second. And there are important reasons why we need to understand this real-world form of time travel.
If you liked this, you may like:

A beginner's guide to time travel
Learn exactly how Einstein's theory of relativity works, and discover how there's nothing in science that says time travel is impossible.
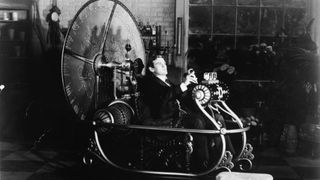
Everyone can travel in time . You do it whether you want to or not, at a steady rate of one second per second. You may think there's no similarity to traveling in one of the three spatial dimensions at, say, one foot per second. But according to Einstein 's theory of relativity , we live in a four-dimensional continuum — space-time — in which space and time are interchangeable.
Einstein found that the faster you move through space, the slower you move through time — you age more slowly, in other words. One of the key ideas in relativity is that nothing can travel faster than the speed of light — about 186,000 miles per second (300,000 kilometers per second), or one light-year per year). But you can get very close to it. If a spaceship were to fly at 99% of the speed of light, you'd see it travel a light-year of distance in just over a year of time.
That's obvious enough, but now comes the weird part. For astronauts onboard that spaceship, the journey would take a mere seven weeks. It's a consequence of relativity called time dilation , and in effect, it means the astronauts have jumped about 10 months into the future.
Traveling at high speed isn't the only way to produce time dilation. Einstein showed that gravitational fields produce a similar effect — even the relatively weak field here on the surface of Earth . We don't notice it, because we spend all our lives here, but more than 12,400 miles (20,000 kilometers) higher up gravity is measurably weaker— and time passes more quickly, by about 45 microseconds per day. That's more significant than you might think, because it's the altitude at which GPS satellites orbit Earth, and their clocks need to be precisely synchronized with ground-based ones for the system to work properly.
The satellites have to compensate for time dilation effects due both to their higher altitude and their faster speed. So whenever you use the GPS feature on your smartphone or your car's satnav, there's a tiny element of time travel involved. You and the satellites are traveling into the future at very slightly different rates.
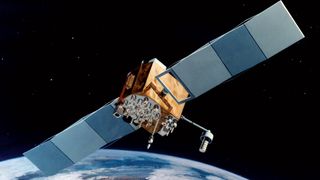
But for more dramatic effects, we need to look at much stronger gravitational fields, such as those around black holes , which can distort space-time so much that it folds back on itself. The result is a so-called wormhole, a concept that's familiar from sci-fi movies, but actually originates in Einstein's theory of relativity. In effect, a wormhole is a shortcut from one point in space-time to another. You enter one black hole, and emerge from another one somewhere else. Unfortunately, it's not as practical a means of transport as Hollywood makes it look. That's because the black hole's gravity would tear you to pieces as you approached it, but it really is possible in theory. And because we're talking about space-time, not just space, the wormhole's exit could be at an earlier time than its entrance; that means you would end up in the past rather than the future.
Trajectories in space-time that loop back into the past are given the technical name "closed timelike curves." If you search through serious academic journals, you'll find plenty of references to them — far more than you'll find to "time travel." But in effect, that's exactly what closed timelike curves are all about — time travel
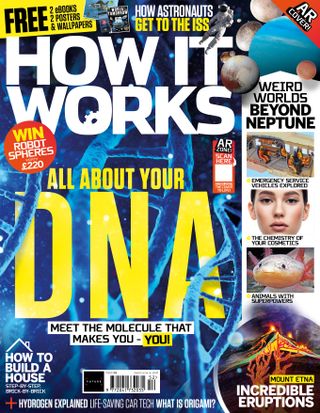
This article is brought to you by How It Works .
How It Works is the action-packed magazine that's bursting with exciting information about the latest advances in science and technology, featuring everything you need to know about how the world around you — and the universe — works.
There's another way to produce a closed timelike curve that doesn't involve anything quite so exotic as a black hole or wormhole: You just need a simple rotating cylinder made of super-dense material. This so-called Tipler cylinder is the closest that real-world physics can get to an actual, genuine time machine. But it will likely never be built in the real world, so like a wormhole, it's more of an academic curiosity than a viable engineering design.
Yet as far-fetched as these things are in practical terms, there's no fundamental scientific reason — that we currently know of — that says they are impossible. That's a thought-provoking situation, because as the physicist Michio Kaku is fond of saying, "Everything not forbidden is compulsory" (borrowed from T.H. White's novel, "The Once And Future King"). He doesn't mean time travel has to happen everywhere all the time, but Kaku is suggesting that the universe is so vast it ought to happen somewhere at least occasionally. Maybe some super-advanced civilization in another galaxy knows how to build a working time machine, or perhaps closed timelike curves can even occur naturally under certain rare conditions.
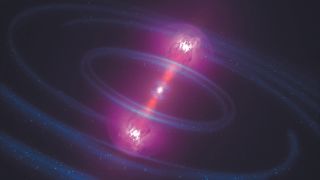
This raises problems of a different kind — not in science or engineering, but in basic logic. If time travel is allowed by the laws of physics, then it's possible to envision a whole range of paradoxical scenarios . Some of these appear so illogical that it's difficult to imagine that they could ever occur. But if they can't, what's stopping them?
Thoughts like these prompted Stephen Hawking , who was always skeptical about the idea of time travel into the past, to come up with his "chronology protection conjecture" — the notion that some as-yet-unknown law of physics prevents closed timelike curves from happening. But that conjecture is only an educated guess, and until it is supported by hard evidence, we can come to only one conclusion: Time travel is possible.
A party for time travelers
Hawking was skeptical about the feasibility of time travel into the past, not because he had disproved it, but because he was bothered by the logical paradoxes it created. In his chronology protection conjecture, he surmised that physicists would eventually discover a flaw in the theory of closed timelike curves that made them impossible.
In 2009, he came up with an amusing way to test this conjecture. Hawking held a champagne party (shown in his Discovery Channel program), but he only advertised it after it had happened. His reasoning was that, if time machines eventually become practical, someone in the future might read about the party and travel back to attend it. But no one did — Hawking sat through the whole evening on his own. This doesn't prove time travel is impossible, but it does suggest that it never becomes a commonplace occurrence here on Earth.
The arrow of time
One of the distinctive things about time is that it has a direction — from past to future. A cup of hot coffee left at room temperature always cools down; it never heats up. Your cellphone loses battery charge when you use it; it never gains charge. These are examples of entropy , essentially a measure of the amount of "useless" as opposed to "useful" energy. The entropy of a closed system always increases, and it's the key factor determining the arrow of time.
It turns out that entropy is the only thing that makes a distinction between past and future. In other branches of physics, like relativity or quantum theory, time doesn't have a preferred direction. No one knows where time's arrow comes from. It may be that it only applies to large, complex systems, in which case subatomic particles may not experience the arrow of time.
Time travel paradox
If it's possible to travel back into the past — even theoretically — it raises a number of brain-twisting paradoxes — such as the grandfather paradox — that even scientists and philosophers find extremely perplexing.
Killing Hitler
A time traveler might decide to go back and kill him in his infancy. If they succeeded, future history books wouldn't even mention Hitler — so what motivation would the time traveler have for going back in time and killing him?
Killing your grandfather
Instead of killing a young Hitler, you might, by accident, kill one of your own ancestors when they were very young. But then you would never be born, so you couldn't travel back in time to kill them, so you would be born after all, and so on …
A closed loop
Suppose the plans for a time machine suddenly appear from thin air on your desk. You spend a few days building it, then use it to send the plans back to your earlier self. But where did those plans originate? Nowhere — they are just looping round and round in time.
Sign up for the Live Science daily newsletter now
Get the world’s most fascinating discoveries delivered straight to your inbox.
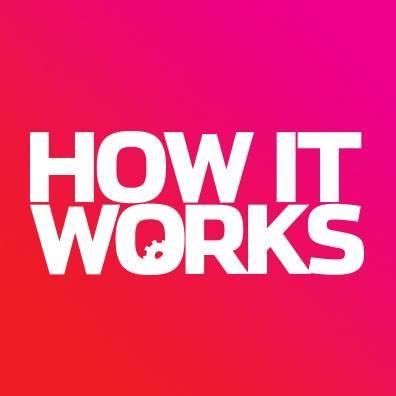
How It Works has a special formula for making learning fun by answering questions on science, space, history, technology, transport and the environment with engaging articles, in-depth special features, global science news, and topical interviews. With impressive cutaway illustrations that show how things function, and mindblowing photography of the planet’s most inspiring spectacles, How It Works represents the pinnacle of engaging, factual fun for a mainstream audience keen to keep up with the latest tech and the most impressive phenomena on the planet and beyond. Written and presented in a style that makes even the most complex subjects interesting and easy to understand, How It Works is enjoyed by readers of all ages.
Get fantastic offers by subscribing to the digital and/or print edition now. Subscribers get 13 issues per year!
Hundreds of black 'spiders' spotted in mysterious 'Inca City' on Mars in new satellite photos
Scientists find one of the oldest stars in the universe in a galaxy right next to ours
World's thinnest gold leaf, dubbed 'goldene,' is just 1 atom thick
Most Popular
- 2 Giant, 82-foot lizard fish discovered on UK beach could be largest marine reptile ever found
- 3 Global 'time signals' subtly shifted as the total solar eclipse reshaped Earth's upper atmosphere, new data shows
- 4 Rare 'porcelain gallbladder' found in 100-year-old unmarked grave at Mississippi mental asylum cemetery
- 5 'I nearly fell out of my chair': 1,800-year-old mini portrait of Alexander the Great found in a field in Denmark
- 2 Hundreds of black 'spiders' spotted in mysterious 'Inca City' on Mars in new satellite photos
- 3 Plato's burial place finally revealed after AI deciphers ancient scroll carbonized in Mount Vesuvius eruption
- 4 China green-lights mass production of autonomous flying taxis — with commercial flights set for 2025
- 5 'We were in disbelief': Antarctica is behaving in a way we've never seen before. Can it recover?
Time travel: Is it possible?
Science says time travel is possible, but probably not in the way you're thinking.
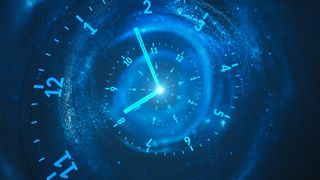
Albert Einstein's theory
- General relativity and GPS
- Wormhole travel
- Alternate theories
Science fiction
Is time travel possible? Short answer: Yes, and you're doing it right now — hurtling into the future at the impressive rate of one second per second.
You're pretty much always moving through time at the same speed, whether you're watching paint dry or wishing you had more hours to visit with a friend from out of town.
But this isn't the kind of time travel that's captivated countless science fiction writers, or spurred a genre so extensive that Wikipedia lists over 400 titles in the category "Movies about Time Travel." In franchises like " Doctor Who ," " Star Trek ," and "Back to the Future" characters climb into some wild vehicle to blast into the past or spin into the future. Once the characters have traveled through time, they grapple with what happens if you change the past or present based on information from the future (which is where time travel stories intersect with the idea of parallel universes or alternate timelines).
Related: The best sci-fi time machines ever
Although many people are fascinated by the idea of changing the past or seeing the future before it's due, no person has ever demonstrated the kind of back-and-forth time travel seen in science fiction or proposed a method of sending a person through significant periods of time that wouldn't destroy them on the way. And, as physicist Stephen Hawking pointed out in his book " Black Holes and Baby Universes" (Bantam, 1994), "The best evidence we have that time travel is not possible, and never will be, is that we have not been invaded by hordes of tourists from the future."
Science does support some amount of time-bending, though. For example, physicist Albert Einstein 's theory of special relativity proposes that time is an illusion that moves relative to an observer. An observer traveling near the speed of light will experience time, with all its aftereffects (boredom, aging, etc.) much more slowly than an observer at rest. That's why astronaut Scott Kelly aged ever so slightly less over the course of a year in orbit than his twin brother who stayed here on Earth.
Related: Controversially, physicist argues that time is real
There are other scientific theories about time travel, including some weird physics that arise around wormholes , black holes and string theory . For the most part, though, time travel remains the domain of an ever-growing array of science fiction books, movies, television shows, comics, video games and more.
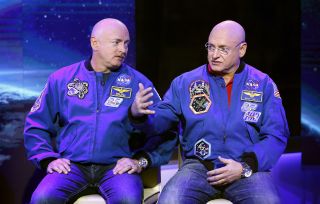
Einstein developed his theory of special relativity in 1905. Along with his later expansion, the theory of general relativity , it has become one of the foundational tenets of modern physics. Special relativity describes the relationship between space and time for objects moving at constant speeds in a straight line.
The short version of the theory is deceptively simple. First, all things are measured in relation to something else — that is to say, there is no "absolute" frame of reference. Second, the speed of light is constant. It stays the same no matter what, and no matter where it's measured from. And third, nothing can go faster than the speed of light.
From those simple tenets unfolds actual, real-life time travel. An observer traveling at high velocity will experience time at a slower rate than an observer who isn't speeding through space.
While we don't accelerate humans to near-light-speed, we do send them swinging around the planet at 17,500 mph (28,160 km/h) aboard the International Space Station . Astronaut Scott Kelly was born after his twin brother, and fellow astronaut, Mark Kelly . Scott Kelly spent 520 days in orbit, while Mark logged 54 days in space. The difference in the speed at which they experienced time over the course of their lifetimes has actually widened the age gap between the two men.
"So, where[as] I used to be just 6 minutes older, now I am 6 minutes and 5 milliseconds older," Mark Kelly said in a panel discussion on July 12, 2020, Space.com previously reported . "Now I've got that over his head."
General relativity and GPS time travel
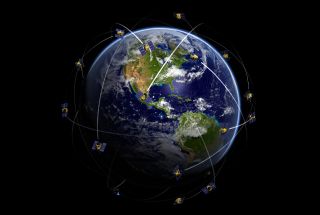
The difference that low earth orbit makes in an astronaut's life span may be negligible — better suited for jokes among siblings than actual life extension or visiting the distant future — but the dilation in time between people on Earth and GPS satellites flying through space does make a difference.
Read more: Can we stop time?
The Global Positioning System , or GPS, helps us know exactly where we are by communicating with a network of a few dozen satellites positioned in a high Earth orbit. The satellites circle the planet from 12,500 miles (20,100 kilometers) away, moving at 8,700 mph (14,000 km/h).
According to special relativity, the faster an object moves relative to another object, the slower that first object experiences time. For GPS satellites with atomic clocks, this effect cuts 7 microseconds, or 7 millionths of a second, off each day, according to the American Physical Society publication Physics Central .
Read more: Could Star Trek's faster-than-light warp drive actually work?
Then, according to general relativity, clocks closer to the center of a large gravitational mass like Earth tick more slowly than those farther away. So, because the GPS satellites are much farther from the center of Earth compared to clocks on the surface, Physics Central added, that adds another 45 microseconds onto the GPS satellite clocks each day. Combined with the negative 7 microseconds from the special relativity calculation, the net result is an added 38 microseconds.
This means that in order to maintain the accuracy needed to pinpoint your car or phone — or, since the system is run by the U.S. Department of Defense, a military drone — engineers must account for an extra 38 microseconds in each satellite's day. The atomic clocks onboard don’t tick over to the next day until they have run 38 microseconds longer than comparable clocks on Earth.
Given those numbers, it would take more than seven years for the atomic clock in a GPS satellite to un-sync itself from an Earth clock by more than a blink of an eye. (We did the math: If you estimate a blink to last at least 100,000 microseconds, as the Harvard Database of Useful Biological Numbers does, it would take thousands of days for those 38 microsecond shifts to add up.)
This kind of time travel may seem as negligible as the Kelly brothers' age gap, but given the hyper-accuracy of modern GPS technology, it actually does matter. If it can communicate with the satellites whizzing overhead, your phone can nail down your location in space and time with incredible accuracy.
Can wormholes take us back in time?
General relativity might also provide scenarios that could allow travelers to go back in time, according to NASA . But the physical reality of those time-travel methods is no piece of cake.
Wormholes are theoretical "tunnels" through the fabric of space-time that could connect different moments or locations in reality to others. Also known as Einstein-Rosen bridges or white holes, as opposed to black holes, speculation about wormholes abounds. But despite taking up a lot of space (or space-time) in science fiction, no wormholes of any kind have been identified in real life.
Related: Best time travel movies
"The whole thing is very hypothetical at this point," Stephen Hsu, a professor of theoretical physics at the University of Oregon, told Space.com sister site Live Science . "No one thinks we're going to find a wormhole anytime soon."
Primordial wormholes are predicted to be just 10^-34 inches (10^-33 centimeters) at the tunnel's "mouth". Previously, they were expected to be too unstable for anything to be able to travel through them. However, a study claims that this is not the case, Live Science reported .
The theory, which suggests that wormholes could work as viable space-time shortcuts, was described by physicist Pascal Koiran. As part of the study, Koiran used the Eddington-Finkelstein metric, as opposed to the Schwarzschild metric which has been used in the majority of previous analyses.
In the past, the path of a particle could not be traced through a hypothetical wormhole. However, using the Eddington-Finkelstein metric, the physicist was able to achieve just that.
Koiran's paper was described in October 2021, in the preprint database arXiv , before being published in the Journal of Modern Physics D.
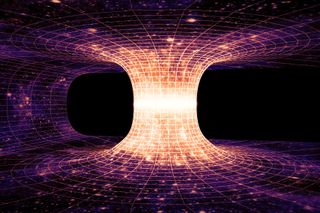
Alternate time travel theories
While Einstein's theories appear to make time travel difficult, some researchers have proposed other solutions that could allow jumps back and forth in time. These alternate theories share one major flaw: As far as scientists can tell, there's no way a person could survive the kind of gravitational pulling and pushing that each solution requires.
Infinite cylinder theory
Astronomer Frank Tipler proposed a mechanism (sometimes known as a Tipler Cylinder ) where one could take matter that is 10 times the sun's mass, then roll it into a very long, but very dense cylinder. The Anderson Institute , a time travel research organization, described the cylinder as "a black hole that has passed through a spaghetti factory."
After spinning this black hole spaghetti a few billion revolutions per minute, a spaceship nearby — following a very precise spiral around the cylinder — could travel backward in time on a "closed, time-like curve," according to the Anderson Institute.
The major problem is that in order for the Tipler Cylinder to become reality, the cylinder would need to be infinitely long or be made of some unknown kind of matter. At least for the foreseeable future, endless interstellar pasta is beyond our reach.
Time donuts
Theoretical physicist Amos Ori at the Technion-Israel Institute of Technology in Haifa, Israel, proposed a model for a time machine made out of curved space-time — a donut-shaped vacuum surrounded by a sphere of normal matter.
"The machine is space-time itself," Ori told Live Science . "If we were to create an area with a warp like this in space that would enable time lines to close on themselves, it might enable future generations to return to visit our time."
Amos Ori is a theoretical physicist at the Technion-Israel Institute of Technology in Haifa, Israel. His research interests and publications span the fields of general relativity, black holes, gravitational waves and closed time lines.
There are a few caveats to Ori's time machine. First, visitors to the past wouldn't be able to travel to times earlier than the invention and construction of the time donut. Second, and more importantly, the invention and construction of this machine would depend on our ability to manipulate gravitational fields at will — a feat that may be theoretically possible but is certainly beyond our immediate reach.
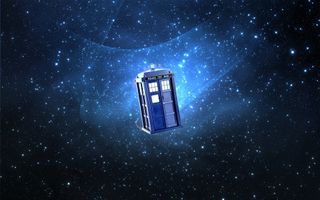
Time travel has long occupied a significant place in fiction. Since as early as the "Mahabharata," an ancient Sanskrit epic poem compiled around 400 B.C., humans have dreamed of warping time, Lisa Yaszek, a professor of science fiction studies at the Georgia Institute of Technology in Atlanta, told Live Science .
Every work of time-travel fiction creates its own version of space-time, glossing over one or more scientific hurdles and paradoxes to achieve its plot requirements.
Some make a nod to research and physics, like " Interstellar ," a 2014 film directed by Christopher Nolan. In the movie, a character played by Matthew McConaughey spends a few hours on a planet orbiting a supermassive black hole, but because of time dilation, observers on Earth experience those hours as a matter of decades.
Others take a more whimsical approach, like the "Doctor Who" television series. The series features the Doctor, an extraterrestrial "Time Lord" who travels in a spaceship resembling a blue British police box. "People assume," the Doctor explained in the show, "that time is a strict progression from cause to effect, but actually from a non-linear, non-subjective viewpoint, it's more like a big ball of wibbly-wobbly, timey-wimey stuff."
Long-standing franchises like the "Star Trek" movies and television series, as well as comic universes like DC and Marvel Comics, revisit the idea of time travel over and over.
Related: Marvel movies in order: chronological & release order
Here is an incomplete (and deeply subjective) list of some influential or notable works of time travel fiction:
Books about time travel:
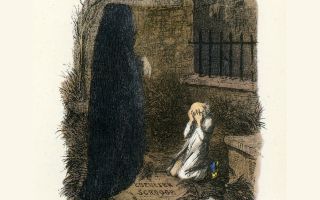
- Rip Van Winkle (Cornelius S. Van Winkle, 1819) by Washington Irving
- A Christmas Carol (Chapman & Hall, 1843) by Charles Dickens
- The Time Machine (William Heinemann, 1895) by H. G. Wells
- A Connecticut Yankee in King Arthur's Court (Charles L. Webster and Co., 1889) by Mark Twain
- The Restaurant at the End of the Universe (Pan Books, 1980) by Douglas Adams
- A Tale of Time City (Methuen, 1987) by Diana Wynn Jones
- The Outlander series (Delacorte Press, 1991-present) by Diana Gabaldon
- Harry Potter and the Prisoner of Azkaban (Bloomsbury/Scholastic, 1999) by J. K. Rowling
- Thief of Time (Doubleday, 2001) by Terry Pratchett
- The Time Traveler's Wife (MacAdam/Cage, 2003) by Audrey Niffenegger
- All You Need is Kill (Shueisha, 2004) by Hiroshi Sakurazaka
Movies about time travel:
- Planet of the Apes (1968)
- Superman (1978)
- Time Bandits (1981)
- The Terminator (1984)
- Back to the Future series (1985, 1989, 1990)
- Star Trek IV: The Voyage Home (1986)
- Bill & Ted's Excellent Adventure (1989)
- Groundhog Day (1993)
- Galaxy Quest (1999)
- The Butterfly Effect (2004)
- 13 Going on 30 (2004)
- The Lake House (2006)
- Meet the Robinsons (2007)
- Hot Tub Time Machine (2010)
- Midnight in Paris (2011)
- Looper (2012)
- X-Men: Days of Future Past (2014)
- Edge of Tomorrow (2014)
- Interstellar (2014)
- Doctor Strange (2016)
- A Wrinkle in Time (2018)
- The Last Sharknado: It's About Time (2018)
- Avengers: Endgame (2019)
- Tenet (2020)
- Palm Springs (2020)
- Zach Snyder's Justice League (2021)
- The Tomorrow War (2021)
Television about time travel:
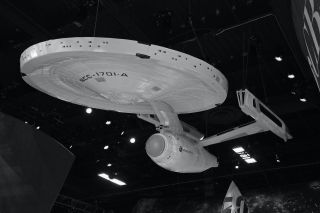
- Doctor Who (1963-present)
- The Twilight Zone (1959-1964) (multiple episodes)
- Star Trek (multiple series, multiple episodes)
- Samurai Jack (2001-2004)
- Lost (2004-2010)
- Phil of the Future (2004-2006)
- Steins;Gate (2011)
- Outlander (2014-2023)
- Loki (2021-present)
Games about time travel:
- Chrono Trigger (1995)
- TimeSplitters (2000-2005)
- Kingdom Hearts (2002-2019)
- Prince of Persia: Sands of Time (2003)
- God of War II (2007)
- Ratchet and Clank Future: A Crack In Time (2009)
- Sly Cooper: Thieves in Time (2013)
- Dishonored 2 (2016)
- Titanfall 2 (2016)
- Outer Wilds (2019)
Additional resources
Explore physicist Peter Millington's thoughts about Stephen Hawking's time travel theories at The Conversation . Check out a kid-friendly explanation of real-world time travel from NASA's Space Place . For an overview of time travel in fiction and the collective consciousness, read " Time Travel: A History " (Pantheon, 2016) by James Gleik.
Join our Space Forums to keep talking space on the latest missions, night sky and more! And if you have a news tip, correction or comment, let us know at: [email protected].
Get the Space.com Newsletter
Breaking space news, the latest updates on rocket launches, skywatching events and more!
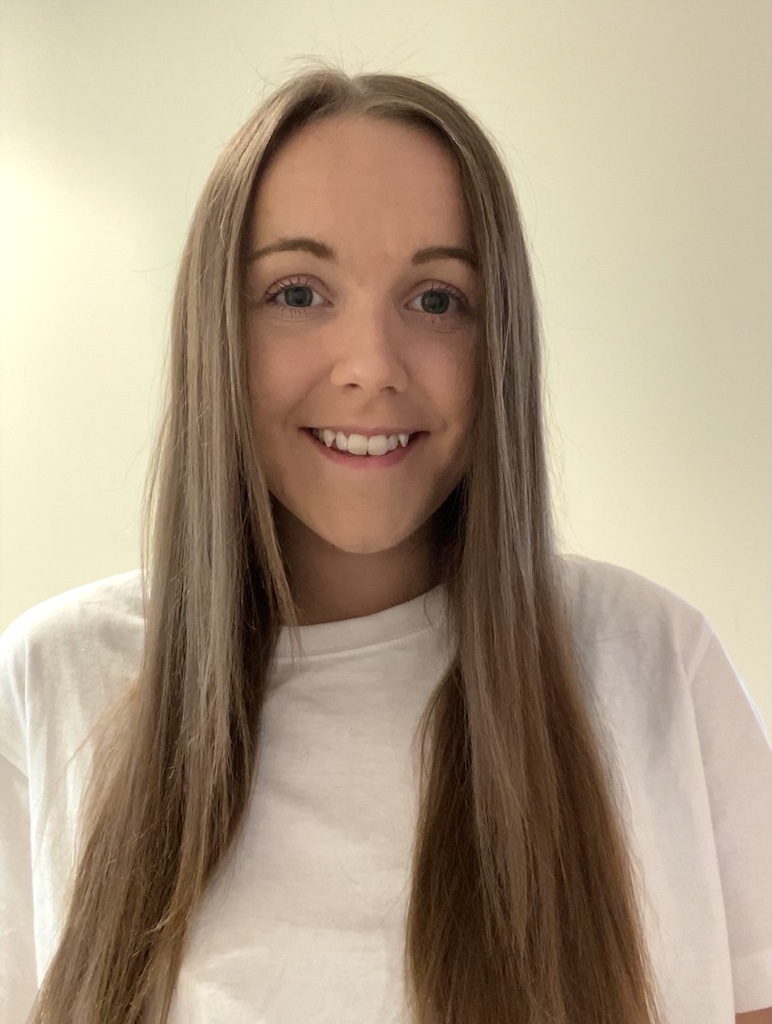
Ailsa is a staff writer for How It Works magazine, where she writes science, technology, space, history and environment features. Based in the U.K., she graduated from the University of Stirling with a BA (Hons) journalism degree. Previously, Ailsa has written for Cardiff Times magazine, Psychology Now and numerous science bookazines.
Satellites watch as 4th global coral bleaching event unfolds (image)
Happy Earth Day 2024! NASA picks 6 new airborne missions to study our changing planet
Boeing's Starliner spacecraft will not fly private missions yet, officials say
Most Popular
- 2 SpaceX launching Falcon 9 rocket on record-tying 20th mission today
- 3 Watch 2 gorgeous supernova remnants evolve over 20 years (timelapse video)
- 4 Boeing Starliner 1st astronaut flight: Live updates
- 5 US Space Force picks Rocket Lab for 2025 Victus Haze space domain awareness mission

- April 24, 2024 | Quantum Computing Meets Genomics: The Dawn of Hyper-Fast DNA Analysis
- April 24, 2024 | Scientists Turn to Venus in the Search for Alien Life
- April 24, 2024 | NASA Astronauts Enter Quarantine As Boeing Starliner Test Flight Approaches
- April 23, 2024 | Peeking Inside Protons: Supercomputers Reveal Quark Secrets
- April 23, 2024 | A Cheaper and More Sustainable Lithium Battery: How LiDFOB Could Change Everything
Exploring the Reality of Time Travel: Science Fact vs. Science Fiction
By Adi Foord, University of Maryland, Baltimore County November 16, 2023
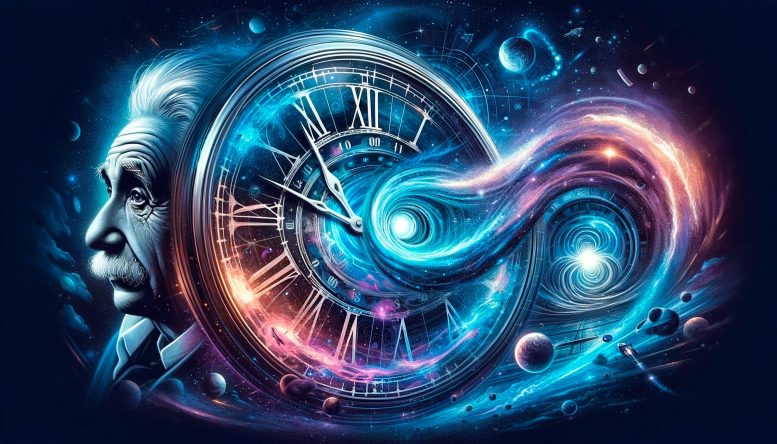
Time travel, a longstanding fascination in science fiction, remains a complex and unresolved concept in science. The second law of thermodynamics suggests time can only move forward, while Einstein’s theory of relativity shows time’s relativity to speed. Theoretical ideas like wormholes offer potential methods, but practical challenges and paradoxes, such as the “grandfather paradox,” complicate the feasibility of actual time travel.
Will it ever be possible for time travel to occur?
Have you ever dreamed of traveling through time, like characters do in science fiction movies? For centuries, the concept of time travel has captivated people’s imaginations. Time travel is the concept of moving between different points in time, just like you move between different places. In movies, you might have seen characters using special machines, magical devices or even hopping into a futuristic car to travel backward or forward in time.
But is this just a fun idea for movies, or could it really happen?
The Science Behind Time Travel
The question of whether time is reversible remains one of the biggest unresolved questions in science. If the universe follows the laws of thermodynamics , it may not be possible. The second law of thermodynamics states that things in the universe can either remain the same or become more disordered over time.
It’s a bit like saying you can’t unscramble eggs once they’ve been cooked. According to this law, the universe can never go back exactly to how it was before. Time can only go forward, like a one-way street.
Time Is Relative
However, physicist Albert Einstein’s theory of special relativity suggests that time passes at different rates for different people. Someone speeding along on a spaceship moving close to the speed of light – 671 million miles per hour! – will experience time slower than a person on Earth.
People have yet to build spaceships that can move at speeds anywhere near as fast as light, but astronauts who visit the International Space Station orbit around the Earth at speeds close to 17,500 mph. Astronaut Scott Kelly has spent 520 days at the International Space Station, and as a result has aged a little more slowly than his twin brother – and fellow astronaut – Mark Kelly. Scott used to be 6 minutes younger than his twin brother. Now, because Scott was traveling so much faster than Mark and for so many days, he is 6 minutes and 5 milliseconds younger .
Theoretical Possibilities and Challenges
Some scientists are exploring other ideas that could theoretically allow time travel. One concept involves wormholes, or hypothetical tunnels in space that could create shortcuts for journeys across the universe. If someone could build a wormhole and then figure out a way to move one end at close to the speed of light – like the hypothetical spaceship mentioned above – the moving end would age more slowly than the stationary end. Someone who entered the moving end and exited the wormhole through the stationary end would come out in their past.
However, wormholes remain theoretical: Scientists have yet to spot one. It also looks like it would be incredibly challenging to send humans through a wormhole space tunnel.
Paradoxes and Failed Dinner Parties
There are also paradoxes associated with time travel. The famous “ grandfather paradox ” is a hypothetical problem that could arise if someone traveled back in time and accidentally prevented their grandparents from meeting. This would create a paradox where you were never born, which raises the question: How could you have traveled back in time in the first place? It’s a mind-boggling puzzle that adds to the mystery of time travel.
Famously, physicist Stephen Hawking tested the possibility of time travel by throwing a dinner party where invitations noting the date, time, and coordinates were not sent out until after it had happened. His hope was that his invitation would be read by someone living in the future, who had capabilities to travel back in time. But no one showed up.
As he pointed out : “The best evidence we have that time travel is not possible, and never will be, is that we have not been invaded by hordes of tourists from the future.”
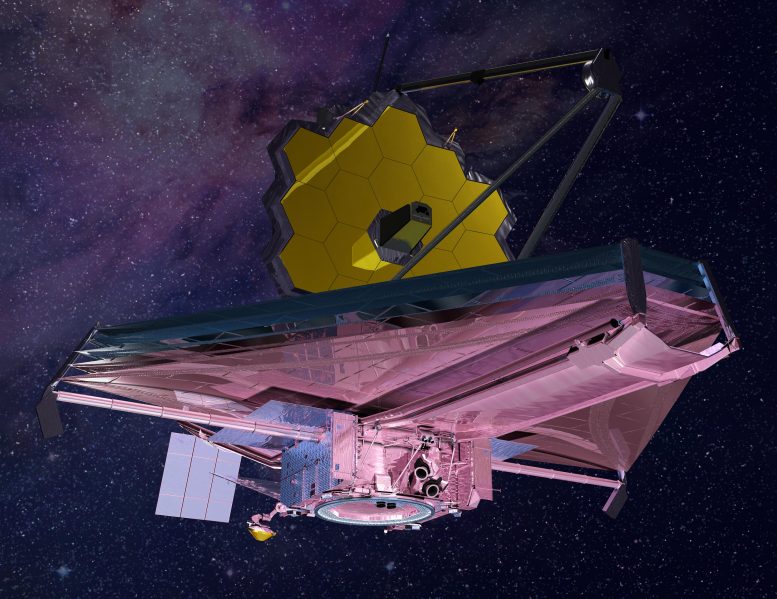
Artist’s rendering of the James Webb Space Telescope. Credit: Northrop Grumman
Telescopes Are Time Machines
Interestingly, astrophysicists armed with powerful telescopes possess a unique form of time travel . As they peer into the vast expanse of the cosmos, they gaze into the past universe. Light from all galaxies and stars takes time to travel, and these beams of light carry information from the distant past. When astrophysicists observe a star or a galaxy through a telescope, they are not seeing it as it is in the present, but as it existed when the light began its journey to Earth millions to billions of years ago.
NASA’s newest space telescope , the James Webb Space Telescope , is peering at galaxies that were formed at the very beginning of the Big Bang , about 13.7 billion years ago.
While we aren’t likely to have time machines like the ones in movies anytime soon, scientists are actively researching and exploring new ideas. But for now, we’ll have to enjoy the idea of time travel in our favorite books, movies, and dreams.
Written by Adi Foord, Assistant Professor of Astronomy and Astrophysics, University of Maryland, Baltimore County.
More on SciTechDaily
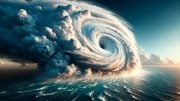
A Significant Surge: How Tropical Cyclones Increase the Cost of Carbon
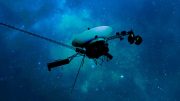
Voyager’s New Horizon: NASA Engineers Tackle Thruster Buildup & Software Glitches
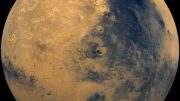
New ScienceCast Video Previews the ‘Opposition of Mars’
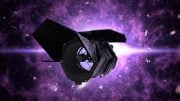
“Our Solar System May Be Unusual”: Rogue Planets Unveiled With NASA’s Roman Space Telescope
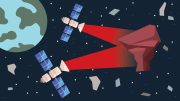
AI-Powered Lasers: A Modern Solution to Space Debris
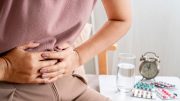
IBS Relief: Common Drug Improves Symptoms of Irritable Bowel Syndrome
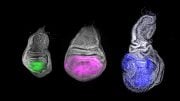
Scientists Discover Chinmo – “The Youth Gene”
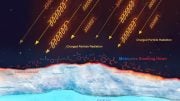
Jupiter’s Moon Europa Could Be Pulling Oxygen Down Below the Ice To Feed Life
8 comments on "exploring the reality of time travel: science fact vs. science fiction".
Until the problem of the second law of thermodynamics(entropy) is solved, the concept of time travel will remain the subject of science fiction. Since this is a basic law of our universe, there is no conceivable way that we know of to do this. The great thing about our knowledge of the universe is that it continues to grow and with that our view of what is possible continues to change. After all, at one time it was believed we could never leave earth!
The 7 planets are soul pollen in the space @ life has been the world has been prepared @ The pollen of the universe is hidden @ Around the axis of the galaxy, the universe is hidden@@ The pollen of the galaxies is a hidden cluster Itis made that we thought about what wisdom is in God’s work and how it is arranged in the form of words.The verse that is made is as follows @@ John, you are in time @ worlds, planets around the axis of the branches of galaxies @@ 8 Prophets, divine prophets, God-aware witnesses @ God’s words of revelation, they are aware @@ 48 What the words of revelation that every prophet has about @ sometimes Sometimes the message of God has become a verbal cliché in the head @@ 62 The message of God was given to every prophet @ The message was made around the power of God @@ 46 The truth of the religions of the cradle of time is said in the world @ Prophets always came for justice in time @@ 62 A warrior became brave in time @ Delaver Ghahrmani Boud Taarani@@ 43 Omar Noah never died, who knows!@ Imransan Is there an unseen world, immortality!?@@ 49 Men’s rights in the sign @ Human rights, the observance of world justice @@ 40 We have God’s love @ A love that is not patched, separation!!!: @@ 39 Take one word from the end of the first and second stanzas to the bottom of these eight verbal verses, and this sentence is made @@ God-aware, the world is born, you know the sign of God @ Agah,the beginning of time, the world, eternity, the world of separation @@ The meaning of this sentence is That God, who before us humans lived on the earth, formed the earth’s crusts with full knowledge, and we humans know the sign of God, which is on the earth, on the continents and countries, and some names have been shown by God for our knowledge since the end of time.In a later video, if I have a lifetime left, I will explain exactly about these poems, God willing @@ The word of the Prophet 17 is the 17th and Muhammad (PBUH) said that the Bedouin Arabs should pray 17 rakats so that theyperform ablution and be clean and not kill and loot.From the caravans, these were all God’s will, and he is good everywhere, in every nation, God does not like evildoers, sinners, and oppressors.Muhammad (PBUH) was God’s last messenger to the Arab people, he was God’s best prophet, and the third verse is because they do not accept Muhammad (PBUH).Some Iranians who were in contact with me, that’s why in the third verse of this surah, he said that his message was given to every prophet, the message is based on divine power, and the word truth, the first two letters of which istruth, is truth, and truth is the 43rd and forty-third word, and the word is time.It is exactly 46, and this song of the Prophets was revealed at the age of 46 ببخشید اگر قبلآ مطالبی فرستادم که به مذاهب مذاهم ارتباط داد شاید به درد شما نمیخورد من در کامنت های بعدی سعی میکنم از نجوم و حیات زمین سخن بگویم این چند بیت را به خارجی انگلیسی که تبدیل کردم معنی آن حیرت انگیز تعغیر کرد گفتم برای شما بفرستم و بداند که این کلام من نبوده کلام خداوند بوده شما نظرتان در مورد خداوند چیست من میگویم خداوند که پدر ومادر انسان بودند قادر به ساختن ما بودند اما آیا آنها قادر به ساختن ستاره ها هم بودند
Your comment has validity to God. But it surely has no place here, it is only fare if the hole comment were in english and has less of a convincing push in a belief a person either believes or not.
It’s too bad physics can’t come to a complete consensus about time, I would like to add some thought about discoveries it has been proven that time travels in only one direction forward, the experiment dealt with light thru glass and how it reacts in the middle and what change happens after light exits the other side, a simple explanation of this experiment. Brings me to theorize and start that time existed before the big bang and is outside of our universes influence, when time is acted on by gravity the ( Form ) of time is changed until the influence no longer has effect, this could go hand in hand with light photons the photon has a influence in the Form that time has. This can not be a observed difference unless we were to see beyond the speed of light. We do agree that physics changes at a subatomic state and also does some strange changing once the speed of light has been exceeded.
The experiment I referred to was posted on IFL in October 2023 headlined ( Solution to complex light problem shows that time can only go Forward ).
One of the problem with travel time is the one people keep forever. And, that is that the earth is always move through space. Matter of fact, the earth is not in the same place that it was 50 years ago. So you will have to move through space as well as time.
Ironically, the only science fiction that seems to handle this is the original story “The Time Machine”.
Time travel is happening now. It has been done since the 1950s. The method satisfies all the requirements. Traveler can’t change the past, but only observe. You can’t go farther back than when the machine was first invented (1950s). There’s one more limitation, you can only observe what the machine was directed. The time machine, the common video camera, and video tape recorder. Now it’s the camera, and file capture computer. Yes, viewing a video tape is effectively going back in time. It’s more than the video, it’s the sound too. There are working versions of smell, and touch which can be recorded too. If you record, and replicate all the senses, you have effectively complete time travel. The most primitive form is the picture. This technology has been around for thousands of years, and is manually intensive. Later many pictures were strung together to make a film. Using a camera to record film was the first example of complete visual time travel (back to when the film was made). Later sound was added, and we have the movies. A way of going back in time that included sight, and sound. Now we have video systems (YouTub) that can play back past events selected by you. Yes, video systems are virtual time travel machines we have now.
How Stellar Cannibalism Illuminates Cosmic Evolution
جزایر فیلیپین دایناسوری که توسط انسان خلق شده در بیش ده ها میلیون سال و بخاطر ریخته شدن دوهزار متر خاک غرق شده بخاطر بالا آمدن آب اقیانوس اما جزایر فیلیپین شبیه دایناسوریست
The address of the above comment on the site about a thief who was trained by a dinosaur bird who was trained by humans tens of millions of years ago and who arrested murderers and robbers.The police were arrested by big birds.don’t the scientists of the world think about this?They were buried in the bed of important cities, they were buried with all the tools and machines, the traces of humans tens of millions of years ago, they had a civilization and a history of hundreds of thousands of years, they built a base underthe earth, from the meteorites that explode from the planets when they hit the sun, and they knew that several thousand meters of soil is poured on the surface of the seas and islands, and they knew that the shapes they made of the islands in thecountry of Papua may go under the ocean, of course, the Philippine islands.The picture is of a baby dinosaur that went under the ocean, but the northern island of Australia, which is Papua, is quite clear.It is a big dinosaur whose tail is towards the east and its mouth is open towards the west.There is the Philippines, but it was more difficult to take the soil to the Philippine islands to create a dinosaur than the island of Papua, that is why the height of the soil in the Philippines is lower, and when the meteorites fell a few kilometerson the surface of the ocean, the image created by humans under the ocean in the shape of a dinosaur is hidden in the American continent The picture is of a bird in the shape of a dinosaur that is flying, and this bird was made to flyby the Indians of the tribe, that bird was talking to people, but its spirit might have heard something from the police because a thief while in the bird’s mouth He was handcuffed by the police and the weapon, which is a machine gun, is fromthe east of the American continent on the coast
The country of Florida is a machine gun.When you continue to New York City, you will reach the mouth of the dinosaur, where a thief is trapped in the mouth of a bird, and the little finger of the police handcuffed the thief’s hand, and a small colt is in the hand ofthe thief, who the police caught in the mouth of the dinosaur.put in the mouth of the bird dinosaur, you can clearly see that the thief fell on the ground and was shot in the head, and it is clear that his forehead was pierced, the bird’s mouth is open in flight, the head of the birdis from the east of the American continent, and a fish is placed in the bird’s mouth in the water of the ocean.The stretched glove of the police, which is in the form of a fist, with a handcuff attached to the left hand of the thief who fell on the ground in the sea and the mouth of the bird, the head of the thief and the killer, is located towards the southwest and west coast of America.All these images were created from the American continent and islands by Humans were created, but they didn’t have enough fuel and time to create more accurate images and meteorites ruined the beauty of the images, but it is clear that all these changes were createdby humans, but you have to consider that two thousand meters of soil from meteorites are fish.And they buried the whales under the beaches, and after a very long time, the bodies of the whales turned into oil under the two thousand meters of soil on the beaches, and on the other hand, the presence of two thousand meters of soil onthe surface of the seas and droughts could not make the created images disappear.
Leave a comment Cancel reply
Email address is optional. If provided, your email will not be published or shared.
Save my name, email, and website in this browser for the next time I comment.

Is time travel even possible? An astrophysicist explains the science behind the science fiction
Published: Nov 13, 2023
By: Magazine Editor
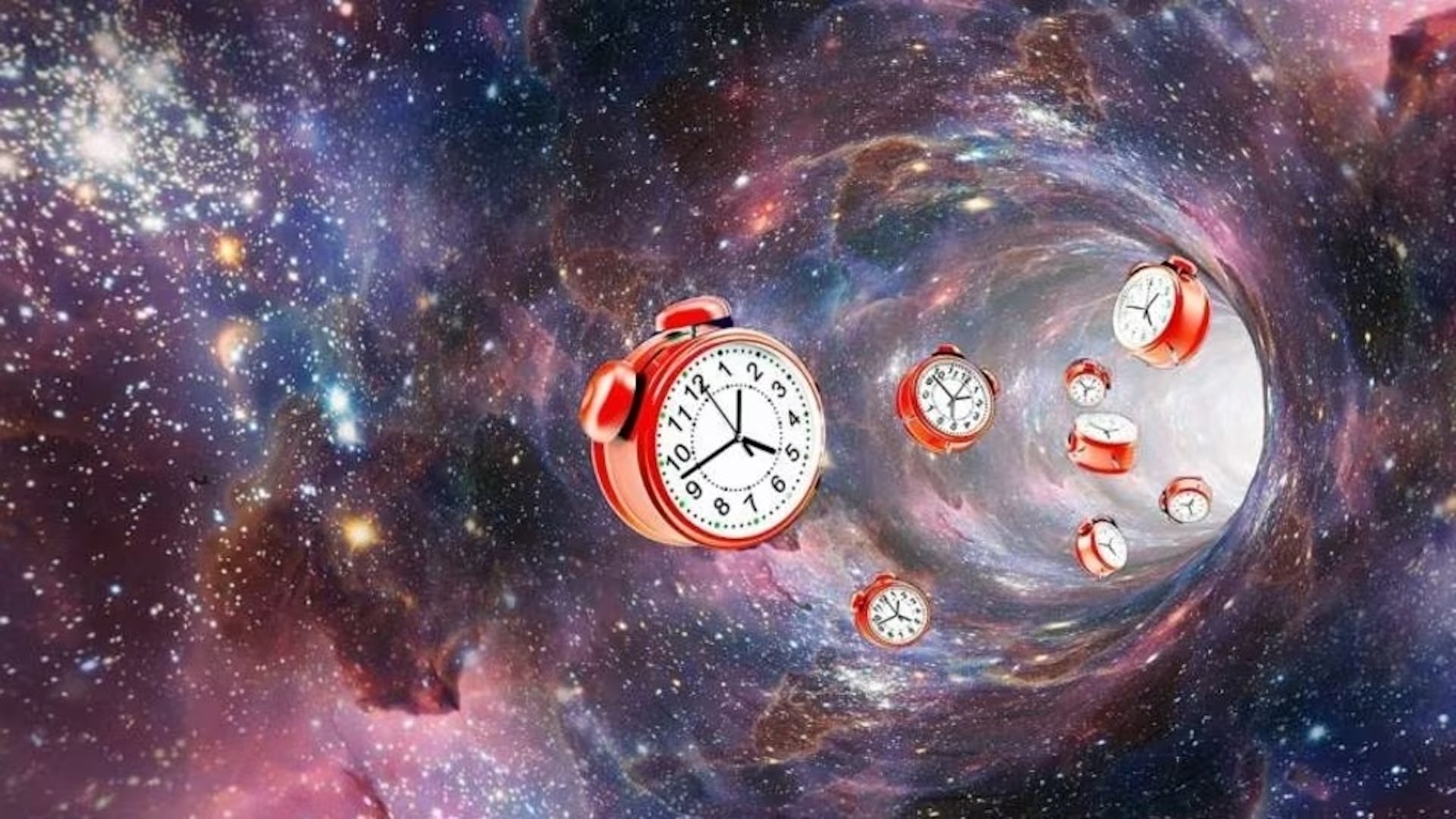
Written by Adi Foord , assistant professor of physics , UMBC
Curious Kids is a series for children of all ages. If you have a question you’d like an expert to answer, send it to [email protected] .
Will it ever be possible for time travel to occur? – Alana C., age 12, Queens, New York
Have you ever dreamed of traveling through time, like characters do in science fiction movies? For centuries, the concept of time travel has captivated people’s imaginations. Time travel is the concept of moving between different points in time, just like you move between different places. In movies, you might have seen characters using special machines, magical devices or even hopping into a futuristic car to travel backward or forward in time.
But is this just a fun idea for movies, or could it really happen?
The question of whether time is reversible remains one of the biggest unresolved questions in science. If the universe follows the laws of thermodynamics , it may not be possible. The second law of thermodynamics states that things in the universe can either remain the same or become more disordered over time.
It’s a bit like saying you can’t unscramble eggs once they’ve been cooked. According to this law, the universe can never go back exactly to how it was before. Time can only go forward, like a one-way street.
Time is relative
However, physicist Albert Einstein’s theory of special relativity suggests that time passes at different rates for different people. Someone speeding along on a spaceship moving close to the speed of light – 671 million miles per hour! – will experience time slower than a person on Earth.
People have yet to build spaceships that can move at speeds anywhere near as fast as light, but astronauts who visit the International Space Station orbit around the Earth at speeds close to 17,500 mph. Astronaut Scott Kelly has spent 520 days at the International Space Station, and as a result has aged a little more slowly than his twin brother – and fellow astronaut – Mark Kelly. Scott used to be 6 minutes younger than his twin brother. Now, because Scott was traveling so much faster than Mark and for so many days, he is 6 minutes and 5 milliseconds younger .
Some scientists are exploring other ideas that could theoretically allow time travel. One concept involves wormholes , or hypothetical tunnels in space that could create shortcuts for journeys across the universe. If someone could build a wormhole and then figure out a way to move one end at close to the speed of light – like the hypothetical spaceship mentioned above – the moving end would age more slowly than the stationary end. Someone who entered the moving end and exited the wormhole through the stationary end would come out in their past.
However, wormholes remain theoretical: Scientists have yet to spot one. It also looks like it would be incredibly challenging to send humans through a wormhole space tunnel.
Paradoxes and failed dinner parties
There are also paradoxes associated with time travel. The famous “ grandfather paradox ” is a hypothetical problem that could arise if someone traveled back in time and accidentally prevented their grandparents from meeting. This would create a paradox where you were never born, which raises the question: How could you have traveled back in time in the first place? It’s a mind-boggling puzzle that adds to the mystery of time travel.
Famously, physicist Stephen Hawking tested the possibility of time travel by throwing a dinner party where invitations noting the date, time and coordinates were not sent out until after it had happened. His hope was that his invitation would be read by someone living in the future, who had capabilities to travel back in time. But no one showed up.
As he pointed out : “The best evidence we have that time travel is not possible, and never will be, is that we have not been invaded by hordes of tourists from the future.”
Telescopes are time machines
Interestingly, astrophysicists armed with powerful telescopes possess a unique form of time travel. As they peer into the vast expanse of the cosmos, they gaze into the past universe. Light from all galaxies and stars takes time to travel, and these beams of light carry information from the distant past. When astrophysicists observe a star or a galaxy through a telescope, they are not seeing it as it is in the present, but as it existed when the light began its journey to Earth millions to billions of years ago. https://www.youtube.com/embed/QeRtcJi3V38?wmode=transparent&start=0 Telescopes are a kind of time machine – they let you peer into the past.
NASA’s newest space telescope, the James Webb Space Telescope , is peering at galaxies that were formed at the very beginning of the Big Bang, about 13.7 billion years ago.
While we aren’t likely to have time machines like the ones in movies anytime soon, scientists are actively researching and exploring new ideas. But for now, we’ll have to enjoy the idea of time travel in our favorite books, movies and dreams.
This article is republished from The Conversation under a Creative Commons license. Read the original article and see more than 250 UMBC articles available in The Conversation.
Tags: CNMS , Physics , The Conversation
Related Posts
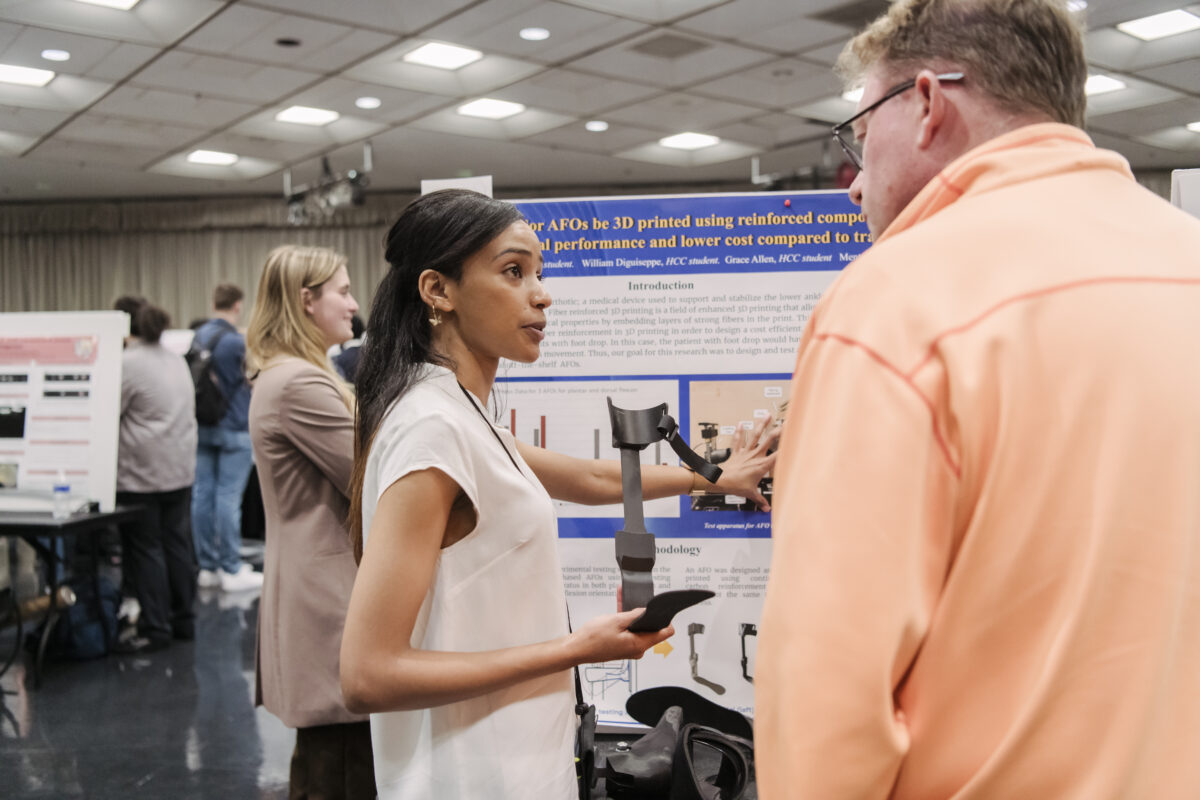
Watch: A recap of URCAD 2024
- Science & Tech
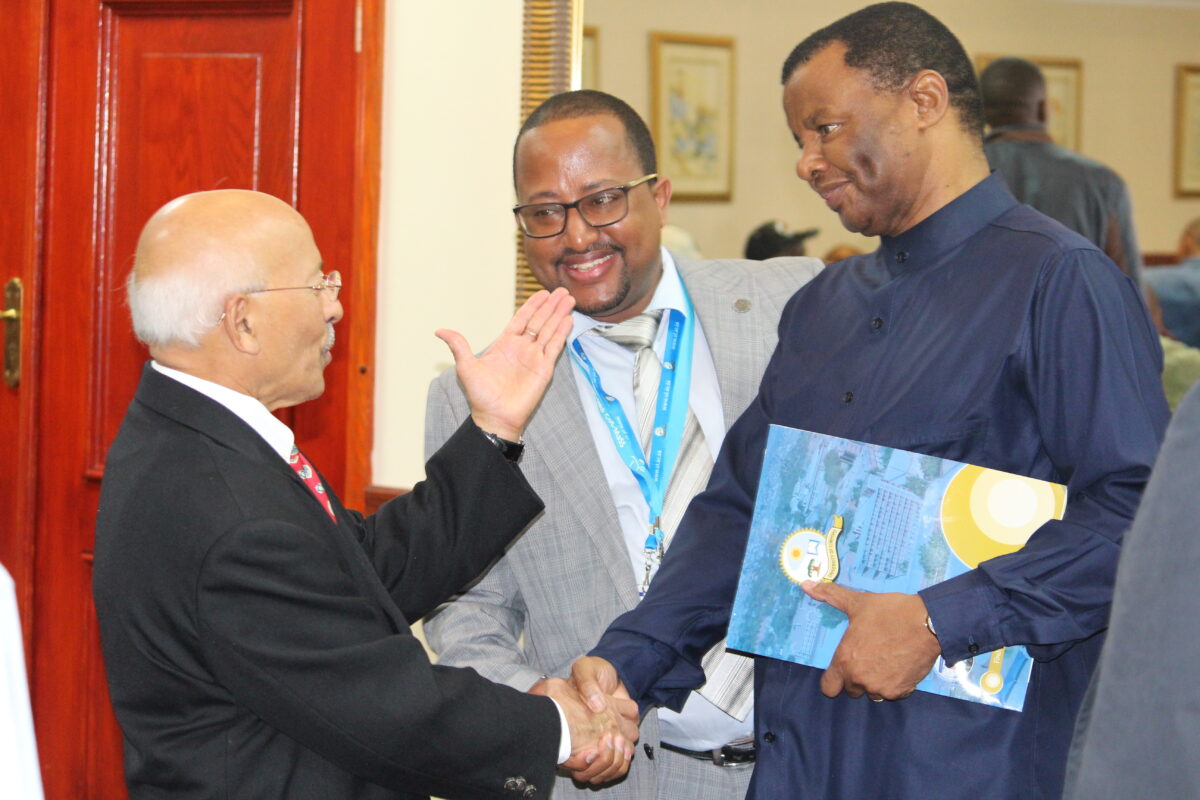
UMBC partners with American Statistical Association to organize annual African International Conference on Statistics
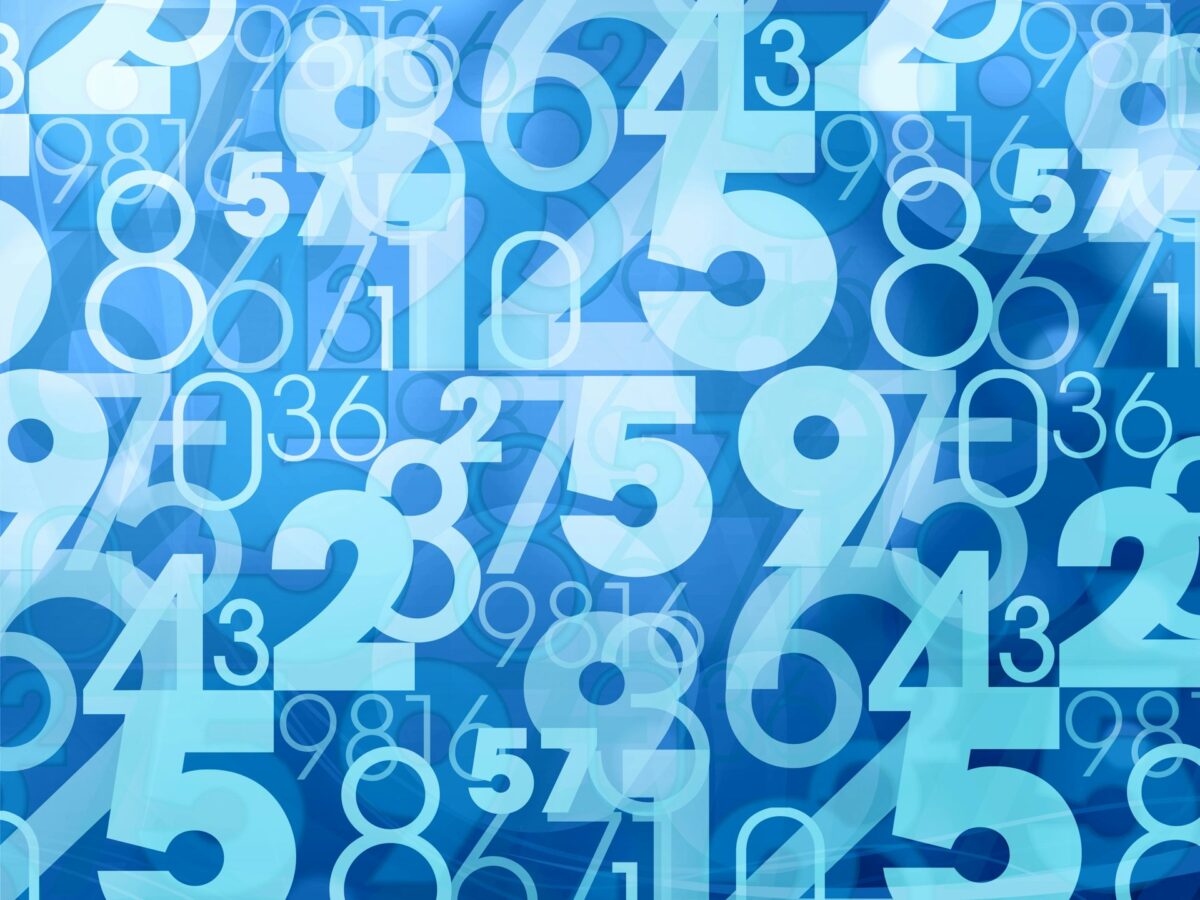
From thousands to millions to billions to trillions to quadrillions and beyond: Do numbers ever end?
Search UMBC Search
- Accreditation
- Consumer Information
- Equal Opportunity
- Privacy PDF Download
- Web Accessibility
Search UMBC.edu
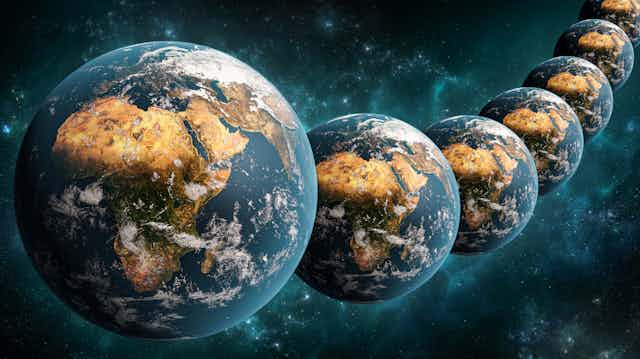
Time travel could be possible, but only with parallel timelines
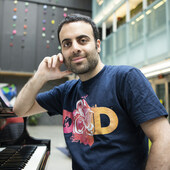
Assistant Professor, Physics, Brock University
Disclosure statement
Barak Shoshany does not work for, consult, own shares in or receive funding from any company or organisation that would benefit from this article, and has disclosed no relevant affiliations beyond their academic appointment.
Brock University provides funding as a member of The Conversation CA-FR.
Brock University provides funding as a member of The Conversation CA.
View all partners
Have you ever made a mistake that you wish you could undo? Correcting past mistakes is one of the reasons we find the concept of time travel so fascinating. As often portrayed in science fiction, with a time machine, nothing is permanent anymore — you can always go back and change it. But is time travel really possible in our universe , or is it just science fiction?
Read more: Curious Kids: is time travel possible for humans?
Our modern understanding of time and causality comes from general relativity . Theoretical physicist Albert Einstein’s theory combines space and time into a single entity — “spacetime” — and provides a remarkably intricate explanation of how they both work, at a level unmatched by any other established theory. This theory has existed for more than 100 years, and has been experimentally verified to extremely high precision, so physicists are fairly certain it provides an accurate description of the causal structure of our universe.
For decades, physicists have been trying to use general relativity to figure out if time travel is possible . It turns out that you can write down equations that describe time travel and are fully compatible and consistent with relativity. But physics is not mathematics, and equations are meaningless if they do not correspond to anything in reality.
Arguments against time travel
There are two main issues which make us think these equations may be unrealistic. The first issue is a practical one: building a time machine seems to require exotic matter , which is matter with negative energy. All the matter we see in our daily lives has positive energy — matter with negative energy is not something you can just find lying around. From quantum mechanics, we know that such matter can theoretically be created, but in too small quantities and for too short times .
However, there is no proof that it is impossible to create exotic matter in sufficient quantities. Furthermore, other equations may be discovered that allow time travel without requiring exotic matter. Therefore, this issue may just be a limitation of our current technology or understanding of quantum mechanics.
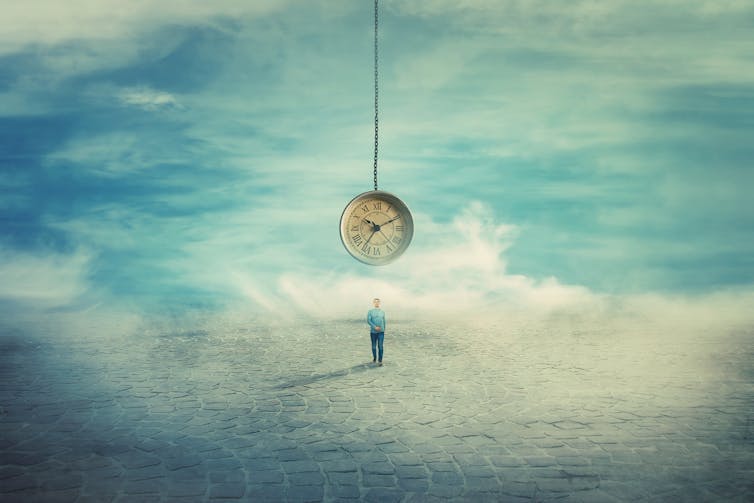
The other main issue is less practical, but more significant: it is the observation that time travel seems to contradict logic, in the form of time travel paradoxes . There are several types of such paradoxes, but the most problematic are consistency paradoxes .
A popular trope in science fiction, consistency paradoxes happen whenever there is a certain event that leads to changing the past, but the change itself prevents this event from happening in the first place.
For example, consider a scenario where I enter my time machine, use it to go back in time five minutes, and destroy the machine as soon as I get to the past. Now that I destroyed the time machine, it would be impossible for me to use it five minutes later.
But if I cannot use the time machine, then I cannot go back in time and destroy it. Therefore, it is not destroyed, so I can go back in time and destroy it. In other words, the time machine is destroyed if and only if it is not destroyed. Since it cannot be both destroyed and not destroyed simultaneously, this scenario is inconsistent and paradoxical.
Eliminating the paradoxes
There’s a common misconception in science fiction that paradoxes can be “created.” Time travellers are usually warned not to make significant changes to the past and to avoid meeting their past selves for this exact reason. Examples of this may be found in many time travel movies, such as the Back to the Future trilogy.
But in physics, a paradox is not an event that can actually happen — it is a purely theoretical concept that points towards an inconsistency in the theory itself. In other words, consistency paradoxes don’t merely imply time travel is a dangerous endeavour, they imply it simply cannot be possible.
This was one of the motivations for theoretical physicist Stephen Hawking to formulate his chronology protection conjecture , which states that time travel should be impossible. However, this conjecture so far remains unproven. Furthermore, the universe would be a much more interesting place if instead of eliminating time travel due to paradoxes, we could just eliminate the paradoxes themselves.
One attempt at resolving time travel paradoxes is theoretical physicist Igor Dmitriyevich Novikov’s self-consistency conjecture , which essentially states that you can travel to the past, but you cannot change it.
According to Novikov, if I tried to destroy my time machine five minutes in the past, I would find that it is impossible to do so. The laws of physics would somehow conspire to preserve consistency.
Introducing multiple histories
But what’s the point of going back in time if you cannot change the past? My recent work, together with my students Jacob Hauser and Jared Wogan, shows that there are time travel paradoxes that Novikov’s conjecture cannot resolve. This takes us back to square one, since if even just one paradox cannot be eliminated, time travel remains logically impossible.
So, is this the final nail in the coffin of time travel? Not quite. We showed that allowing for multiple histories (or in more familiar terms, parallel timelines) can resolve the paradoxes that Novikov’s conjecture cannot. In fact, it can resolve any paradox you throw at it.
The idea is very simple. When I exit the time machine, I exit into a different timeline. In that timeline, I can do whatever I want, including destroying the time machine, without changing anything in the original timeline I came from. Since I cannot destroy the time machine in the original timeline, which is the one I actually used to travel back in time, there is no paradox.
After working on time travel paradoxes for the last three years , I have become increasingly convinced that time travel could be possible, but only if our universe can allow multiple histories to coexist. So, can it?
Quantum mechanics certainly seems to imply so, at least if you subscribe to Everett’s “many-worlds” interpretation , where one history can “split” into multiple histories, one for each possible measurement outcome – for example, whether Schrödinger’s cat is alive or dead, or whether or not I arrived in the past.
But these are just speculations. My students and I are currently working on finding a concrete theory of time travel with multiple histories that is fully compatible with general relativity. Of course, even if we manage to find such a theory, this would not be sufficient to prove that time travel is possible, but it would at least mean that time travel is not ruled out by consistency paradoxes.
Time travel and parallel timelines almost always go hand-in-hand in science fiction, but now we have proof that they must go hand-in-hand in real science as well. General relativity and quantum mechanics tell us that time travel might be possible, but if it is, then multiple histories must also be possible.
- Time travel
- Theoretical physics
- Time machine
- Albert Einstein
- Listen to this article
- Time travel paradox

Program Manager, Teaching & Learning Initiatives

Lecturer/Senior Lecturer, Earth System Science (School of Science)

Sydney Horizon Educators (Identified)

Deputy Social Media Producer

Associate Professor, Occupational Therapy
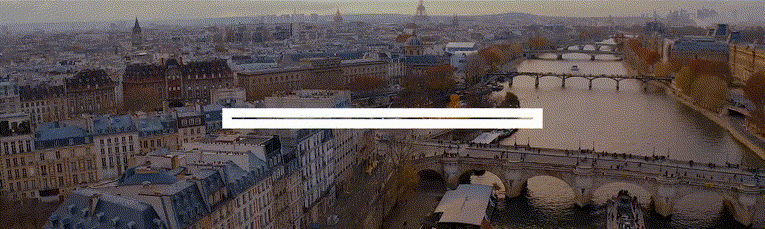
Is Time Travel Possible?
- Cosmology & Astrophysics
- Physics Laws, Concepts, and Principles
- Quantum Physics
- Important Physicists
- Thermodynamics
- Weather & Climate
:max_bytes(150000):strip_icc():format(webp)/AZJFaceShot-56a72b155f9b58b7d0e783fa.jpg)
- M.S., Mathematics Education, Indiana University
- B.A., Physics, Wabash College
Stories regarding travel into the past and the future have long captured our imagination, but the question of whether time travel is possible is a thorny one that gets right to the heart of understanding what physicists mean when they use the word "time."
Modern physics teaches us that time is one of the most mysterious aspects of our universe, though it may at first seem straightforward. Einstein revolutionized our understanding of the concept, but even with this revised understanding, some scientists still ponder the question of whether or not time actually exists or whether it is a mere "stubbornly persistent illusion" (as Einstein once called it). Whatever time is, though, physicists (and fiction writers) have found some interesting ways to manipulate it to consider traversing it in unorthodox ways.
Time and Relativity
Though referenced in H.G. Wells' The Time Machine (1895), the actual science of time travel didn't come into being until well into the twentieth century, as a side-effect of Albert Einstein 's theory of general relativity (developed in 1915). Relativity describes the physical fabric of the universe in terms of a 4-dimensional spacetime, which includes three spatial dimensions (up/down, left/right, and front/back) along with one time dimension. Under this theory, which has been proven by numerous experiments over the last century, gravity is a result of the bending of this spacetime in response to the presence of matter. In other words, given a certain configuration of matter, the actual spacetime fabric of the universe can be altered in significant ways.
One of the amazing consequences of relativity is that movement can result in a difference in the way time passes, a process known as time dilation . This is most dramatically manifested in the classic Twin Paradox . In this method of "time travel," you can move into the future faster than normal, but there's not really any way back. (There's a slight exception, but more on that later in the article.)
Early Time Travel
In 1937, Scottish physicist W. J. van Stockum first applied general relativity in a way that opened the door for time travel. By applying the equation of general relativity to a situation with an infinitely long, extremely dense rotating cylinder (kind of like an endless barbershop pole). The rotation of such a massive object actually creates a phenomenon known as "frame dragging," which is that it actually drags spacetime along with it. Van Stockum found that in this situation, you could create a path in 4-dimensional spacetime which began and ended at the same point - something called a closed timelike curve - which is the physical result that allows time travel. You can set off in a space ship and travel a path which brings you back to the exact same moment you started out at.
Though an intriguing result, this was a fairly contrived situation, so there wasn't really much concern about it taking place. A new interpretation was about to come along, however, which was much more controversial.
In 1949, the mathematician Kurt Godel - a friend of Einstein's and a colleague at Princeton University's Institute for Advanced Study - decided to tackle a situation where the whole universe is rotating. In Godel's solutions, time travel was actually allowed by the equations if the universe were rotating. A rotating universe could itself function as a time machine.
Now, if the universe were rotating, there would be ways to detect it (light beams would bend, for example, if the whole universe were rotating), and so far the evidence is overwhelmingly strong that there is no sort of universal rotation. So again, time travel is ruled out by this particular set of results. But the fact is that things in the universe do rotate, and that again opens up the possibility.
Time Travel and Black Holes
In 1963, New Zealand mathematician Roy Kerr used the field equations to analyze a rotating black hole , called a Kerr black hole, and found that the results allowed a path through a wormhole in the black hole, missing the singularity at the center, and make it out the other end. This scenario also allows for closed timelike curves, as theoretical physicist Kip Thorne realized years later.
In the early 1980s, while Carl Sagan worked on his 1985 novel Contact , he approached Kip Thorne with a question about the physics of time travel, which inspired Thorne to examine the concept of using a black hole as a means of time travel. Together with the physicist Sung-Won Kim, Thorne realized that you could (in theory) have a black hole with a wormhole connecting it to another point in space held open by some form of negative energy.
But just because you have a wormhole doesn't mean that you have a time machine. Now, let's assume that you could move one end of the wormhole (the "movable end). You place the movable end on a spaceship, shooting it off into space at nearly the speed of light . Time dilation kicks in, and the time experienced by the movable end is much less than the time experienced by the fixed end. Let's assume that you move the movable end 5,000 years into the future of the Earth, but the movable end only "ages" 5 years. So you leave in 2010 AD, say, and arrive in 7010 AD.
However, if you travel through the movable end, you will actually pop out of the fixed end in 2015 AD (since 5 years have passed back on Earth). What? How does this work?
Well, the fact is that the two ends of the wormhole are connected. No matter how far apart they are, in spacetime, they're still basically "near" each other. Since the movable end is only five years older than when it left, going through it will send you back to the related point on the fixed wormhole. And if someone from 2015 AD Earth steps through the fixed wormhole, they'd come out in 7010 AD from the movable wormhole. (If someone stepped through the wormhole in 2012 AD, they'd end up on the spaceship somewhere in the middle of the trip and so on.)
Though this is the most physically reasonable description of a time machine, there are still problems. No one knows if wormholes or negative energy exist, nor how to put them together in this way if they do exist. But it is (in theory) possible.
- Can We Travel Through Time to the Past?
- Closed Timelike Curve
- Wormholes: What Are They and Can We Use Them?
- What Is the Twin Paradox? Real Time Travel
- Time Travel: Dream or Possible Reality?
- Einstein's Theory of Relativity
- The Science of Star Trek
- What Is Time? A Simple Explanation
- Understanding Time Dilation Effects in Physics
- Is Warp Drive From 'Star Trek' Possible?
- Understanding Cosmology and Its Impact
- An Introduction to Black Holes
- Cosmos Episode 4 Viewing Worksheet
- The History of Gravity
- Spiral Galaxies
- Black Holes and Hawking Radiation
To revisit this article, visit My Profile, then View saved stories .
- Backchannel
- Newsletters
- WIRED Insider
- WIRED Consulting
Oliver Franklin-Wallis
A brief history of time travel
Subscribe to WIRED
Of all time travel's paradoxes, here's the strangest of them all: hop on a TARDIS back to 1894 and the concept didn't even exist. "Time travel is a new idea," explains New York-based author James Gleick, 62. "It's a very modern myth." Gleick's entertaining Time Travel: A History , out in hardback in February, quantum leaps from HG Wells's The Time Machine - the original - via Proust and alt-history right up to your Twitter timeline. Until we get the DeLorean working for real, fellow travellers, consider it the next best thing.
The Mahabharata
Time travel appears in Hindu text The Mahabharata, and in stories such as Washington Irving's Rip Van Winkle (1819) - but it usually only involved a one-way trip. "People fell asleep, and woke up in the future," says Gleick.
HG Wells's The Time Machine
"The idea of time travel with volition, in either direction, didn't arrive until Wells," says Gleick. It explains that time is a dimension - something not widely accepted until Einstein's theories in 1905.
Henri Bergson's Time And Free Will
Bergson's thesis is published soon after Wells's novel. "Bergson is a friend of Marcel Proust," says Gleick. Soon Proust et al are jumping on the idea of time travel to explore free will - and influencing new sci-fi in return.
Time Capsules
The idea of preserving a time stamp only arose in the 1930s in Scientific American. "It's the most pedestrian form of time travel: sending something into the future at a rate of one minute per minute."
Robert A Heinlein's By His Bootstraps
Heinlein's short story, published in Astounding Science Fiction, introduces the idea of a character appearing in multiple timelines, meeting themselves amid complex - and funny - paradoxes.
William Gibson's The Peripheral
Gleick cites Gibson's unique twist on the genre: "We can't send people, but what if you could send information back to the past?" It's a chilling new take. "It shows how our cultural conception of time is changing."
This article was originally published by WIRED UK
Matt Jancer
David Nield
David Kushner
Matt Reynolds
Rachel Lance
Jessica Rawnsley
Emily Mullin

- Table of Contents
- Random Entry
- Chronological
- Editorial Information
- About the SEP
- Editorial Board
- How to Cite the SEP
- Special Characters
- Advanced Tools
- Support the SEP
- PDFs for SEP Friends
- Make a Donation
- SEPIA for Libraries
- Entry Contents
Bibliography
Academic tools.
- Friends PDF Preview
- Author and Citation Info
- Back to Top
Time Travel and Modern Physics
Time travel has been a staple of science fiction. With the advent of general relativity it has been entertained by serious physicists. But, especially in the philosophy literature, there have been arguments that time travel is inherently paradoxical. The most famous paradox is the grandfather paradox: you travel back in time and kill your grandfather, thereby preventing your own existence. To avoid inconsistency some circumstance will have to occur which makes you fail in this attempt to kill your grandfather. Doesn’t this require some implausible constraint on otherwise unrelated circumstances? We examine such worries in the context of modern physics.
1. Paradoxes Lost?
2. topology and constraints, 3. the general possibility of time travel in general relativity, 4. two toy models, 5. slightly more realistic models of time travel, 6. the possibility of time travel redux, 7. even if there are constraints, so what, 8. computational models, 9. quantum mechanics to the rescue, 10. conclusions, other internet resources, related entries.
- Supplement: Remarks and Limitations on the Toy Models
Modern physics strips away many aspects of the manifest image of time. Time as it appears in the equations of classical mechanics has no need for a distinguished present moment, for example. Relativity theory leads to even sharper contrasts. It replaces absolute simultaneity, according to which it is possible to unambiguously determine the time order of distant events, with relative simultaneity: extending an “instant of time” throughout space is not unique, but depends on the state of motion of an observer. More dramatically, in general relativity the mathematical properties of time (or better, of spacetime)—its topology and geometry—depend upon how matter is arranged rather than being fixed once and for all. So physics can be, and indeed has to be, formulated without treating time as a universal, fixed background structure. Since general relativity represents gravity through spacetime geometry, the allowed geometries must be as varied as the ways in which matter can be arranged. Alongside geometrical models used to describe the solar system, black holes, and much else, the scope of variation extends to include some exotic structures unlike anything astrophysicists have observed. In particular, there are spacetime geometries with curves that loop back on themselves: closed timelike curves (CTCs), which describe the possible trajectory of an observer who returns exactly back to their earlier state—without any funny business, such as going faster than the speed of light. These geometries satisfy the relevant physical laws, the equations of general relativity, and in that sense time travel is physically possible.
Yet circular time generates paradoxes, familiar from science fiction stories featuring time travel: [ 1 ]
- Consistency: Kurt plans to murder his own grandfather Adolph, by traveling along a CTC to an appropriate moment in the past. He is an able marksman, and waits until he has a clear shot at grandpa. Normally he would not miss. Yet if he succeeds, there is no way that he will then exist to plan and carry out the mission. Kurt pulls the trigger: what can happen?
- Underdetermination: Suppose that Kurt first travels back in order to give his earlier self a copy of How to Build a Time Machine. This is the same book that allows him to build a time machine, which he then carries with him on his journey to the past. Who wrote the book?
- Easy Knowledge: A fan of classical music enhances their computer with a circuit that exploits a CTC. This machine efficiently solves problems at a higher level of computational complexity than conventional computers, leading (among other things) to finding the smallest circuits that can generate Bach’s oeuvre—and to compose new pieces in the same style. Such easy knowledge is at odds with our understanding of our epistemic predicament. (This third paradox has not drawn as much attention.)
The first two paradoxes were once routinely taken to show that solutions with CTCs should be rejected—with charges varying from violating logic, to being “physically unreasonable”, to undermining the notion of free will. Closer analysis of the paradoxes has largely reversed this consensus. Physicists have discovered many solutions with CTCs and have explored their properties in pursuing foundational questions, such as whether physics is compatible with the idea of objective temporal passage (starting with Gödel 1949). Philosophers have also used time travel scenarios to probe questions about, among other things, causation, modality, free will, and identity (see, e.g., Earman 1972 and Lewis’s seminal 1976 paper).
We begin below with Consistency , turning to the other paradoxes in later sections. A standard, stone-walling response is to insist that the past cannot be changed, as a matter of logic, even by a time traveler (e.g., Gödel 1949, Clarke 1977, Horwich 1987). Adolph cannot both die and survive, as a matter of logic, so any scheme to alter the past must fail. In many of the best time travel fictions, the actions of a time traveler are constrained in novel and unexpected ways. Attempts to change the past fail, and they fail, often tragically, in just such a way that they set the stage for the time traveler’s self-defeating journey. The first question is whether there is an analog of the consistent story when it comes to physics in the presence of CTCs. As we will see, there is a remarkable general argument establishing the existence of consistent solutions. Yet a second question persists: why can’t time-traveling Kurt kill his own grandfather? Doesn’t the necessity of failures to change the past put unusual and unexpected constraints on time travelers, or objects that move along CTCs? The same argument shows that there are in fact no constraints imposed by the existence of CTCs, in some cases. After discussing this line of argument, we will turn to the palatability and further implications of such constraints if they are required, and then turn to the implications of quantum mechanics.
Wheeler and Feynman (1949) were the first to claim that the fact that nature is continuous could be used to argue that causal influences from later events to earlier events, as are made possible by time travel, will not lead to paradox without the need for any constraints. Maudlin (1990) showed how to make their argument precise and more general, and argued that nonetheless it was not completely general.
Imagine the following set-up. We start off having a camera with a black and white film ready to take a picture of whatever comes out of the time machine. An object, in fact a developed film, comes out of the time machine. We photograph it, and develop the film. The developed film is subsequently put in the time machine, and set to come out of the time machine at the time the picture is taken. This surely will create a paradox: the developed film will have the opposite distribution of black, white, and shades of gray, from the object that comes out of the time machine. For developed black and white films (i.e., negatives) have the opposite shades of gray from the objects they are pictures of. But since the object that comes out of the time machine is the developed film itself it we surely have a paradox.
However, it does not take much thought to realize that there is no paradox here. What will happen is that a uniformly gray picture will emerge, which produces a developed film that has exactly the same uniform shade of gray. No matter what the sensitivity of the film is, as long as the dependence of the brightness of the developed film depends in a continuous manner on the brightness of the object being photographed, there will be a shade of gray that, when photographed, will produce exactly the same shade of gray on the developed film. This is the essence of Wheeler and Feynman’s idea. Let us first be a bit more precise and then a bit more general.
For simplicity let us suppose that the film is always a uniform shade of gray (i.e., at any time the shade of gray does not vary by location on the film). The possible shades of gray of the film can then be represented by the (real) numbers from 0, representing pure black, to 1, representing pure white.
Let us now distinguish various stages in the chronological order of the life of the film. In stage \(S_1\) the film is young; it has just been placed in the camera and is ready to be exposed. It is then exposed to the object that comes out of the time machine. (That object in fact is a later stage of the film itself). By the time we come to stage \(S_2\) of the life of the film, it has been developed and is about to enter the time machine. Stage \(S_3\) occurs just after it exits the time machine and just before it is photographed. Stage \(S_4\) occurs after it has been photographed and before it starts fading away. Let us assume that the film starts out in stage \(S_1\) in some uniform shade of gray, and that the only significant change in the shade of gray of the film occurs between stages \(S_1\) and \(S_2\). During that period it acquires a shade of gray that depends on the shade of gray of the object that was photographed. In other words, the shade of gray that the film acquires at stage \(S_2\) depends on the shade of gray it has at stage \(S_3\). The influence of the shade of gray of the film at stage \(S_3\), on the shade of gray of the film at stage \(S_2\), can be represented as a mapping, or function, from the real numbers between 0 and 1 (inclusive), to the real numbers between 0 and 1 (inclusive). Let us suppose that the process of photography is such that if one imagines varying the shade of gray of an object in a smooth, continuous manner then the shade of gray of the developed picture of that object will also vary in a smooth, continuous manner. This implies that the function in question will be a continuous function. Now any continuous function from the real numbers between 0 and 1 (inclusive) to the real numbers between 0 and 1 (inclusive) must map at least one number to itself. One can quickly convince oneself of this by graphing such functions. For one will quickly see that any continuous function \(f\) from \([0,1]\) to \([0,1]\) must intersect the line \(x=y\) somewhere, and thus there must be at least one point \(x\) such that \(f(x)=x\). Such points are called fixed points of the function. Now let us think about what such a fixed point represents. It represents a shade of gray such that, when photographed, it will produce a developed film with exactly that same shade of gray. The existence of such a fixed point implies a solution to the apparent paradox.
Let us now be more general and allow color photography. One can represent each possible color of an object (of uniform color) by the proportions of blue, green and red that make up that color. (This is why television screens can produce all possible colors.) Thus one can represent all possible colors of an object by three points on three orthogonal lines \(x, y\) and \(z\), that is to say, by a point in a three-dimensional cube. This cube is also known as the “Cartesian product” of the three line segments. Now, one can also show that any continuous map from such a cube to itself must have at least one fixed point. So color photography can not be used to create time travel paradoxes either!
Even more generally, consider some system \(P\) which, as in the above example, has the following life. It starts in some state \(S_1\), it interacts with an object that comes out of a time machine (which happens to be its older self), it travels back in time, it interacts with some object (which happens to be its younger self), and finally it grows old and dies. Let us assume that the set of possible states of \(P\) can be represented by a Cartesian product of \(n\) closed intervals of the reals, i.e., let us assume that the topology of the state-space of \(P\) is isomorphic to a finite Cartesian product of closed intervals of the reals. Let us further assume that the development of \(P\) in time, and the dependence of that development on the state of objects that it interacts with, is continuous. Then, by a well-known fixed point theorem in topology (see, e.g., Hocking & Young 1961: 273), no matter what the nature of the interaction is, and no matter what the initial state of the object is, there will be at least one state \(S_3\) of the older system (as it emerges from the time travel machine) that will influence the initial state \(S_1\) of the younger system (when it encounters the older system) so that, as the younger system becomes older, it develops exactly into state \(S_3\). Thus without imposing any constraints on the initial state \(S_1\) of the system \(P\), we have shown that there will always be perfectly ordinary, non-paradoxical, solutions, in which everything that happens, happens according to the usual laws of development. Of course, there is looped causation, hence presumably also looped explanation, but what do you expect if there is looped time?
Unfortunately, for the fan of time travel, a little reflection suggests that there are systems for which the needed fixed point theorem does not hold. Imagine, for instance, that we have a dial that can only rotate in a plane. We are going to put the dial in the time machine. Indeed we have decided that if we see the later stage of the dial come out of the time machine set at angle \(x\), then we will set the dial to \(x+90\), and throw it into the time machine. Now it seems we have a paradox, since the mapping that consists of a rotation of all points in a circular state-space by 90 degrees does not have a fixed point. And why wouldn’t some state-spaces have the topology of a circle?
However, we have so far not used another continuity assumption which is also a reasonable assumption. So far we have only made the following demand: the state the dial is in at stage \(S_2\) must be a continuous function of the state of the dial at stage \(S_3\). But, the state of the dial at stage \(S_2\) is arrived at by taking the state of the dial at stage \(S_1\), and rotating it over some angle. It is not merely the case that the effect of the interaction, namely the state of the dial at stage \(S_2\), should be a continuous function of the cause, namely the state of the dial at stage \(S_3\). It is additionally the case that path taken to get there, the way the dial is rotated between stages \(S_1\) and \(S_2\) must be a continuous function of the state at stage \(S_3\). And, rather surprisingly, it turns out that this can not be done. Let us illustrate what the problem is before going to a more general demonstration that there must be a fixed point solution in the dial case.
Forget time travel for the moment. Suppose that you and I each have a watch with a single dial neither of which is running. My watch is set at 12. You are going to announce what your watch is set at. My task is going to be to adjust my watch to yours no matter what announcement you make. And my actions should have a continuous (single valued) dependence on the time that you announce. Surprisingly, this is not possible! For instance, suppose that if you announce “12”, then I achieve that setting on my watch by doing nothing. Now imagine slowly and continuously increasing the announced times, starting at 12. By continuity, I must achieve each of those settings by rotating my dial to the right. If at some point I switch and achieve the announced goal by a rotation of my dial to the left, I will have introduced a discontinuity in my actions, a discontinuity in the actions that I take as a function of the announced angle. So I will be forced, by continuity, to achieve every announcement by rotating the dial to the right. But, this rotation to the right will have to be abruptly discontinued as the announcements grow larger and I eventually approach 12 again, since I achieved 12 by not rotating the dial at all. So, there will be a discontinuity at 12 at the latest. In general, continuity of my actions as a function of announced times can not be maintained throughout if I am to be able to replicate all possible settings. Another way to see the problem is that one can similarly reason that, as one starts with 12, and imagines continuously making the announced times earlier, one will be forced, by continuity, to achieve the announced times by rotating the dial to the left. But the conclusions drawn from the assumption of continuous increases and the assumption of continuous decreases are inconsistent. So we have an inconsistency following from the assumption of continuity and the assumption that I always manage to set my watch to your watch. So, a dial developing according to a continuous dynamics from a given initial state, can not be set up so as to react to a second dial, with which it interacts, in such a way that it is guaranteed to always end up set at the same angle as the second dial. Similarly, it can not be set up so that it is guaranteed to always end up set at 90 degrees to the setting of the second dial. All of this has nothing to do with time travel. However, the impossibility of such set ups is what prevents us from enacting the rotation by 90 degrees that would create paradox in the time travel setting.
Let us now give the positive result that with such dials there will always be fixed point solutions, as long as the dynamics is continuous. Let us call the state of the dial before it interacts with its older self the initial state of the dial. And let us call the state of the dial after it emerges from the time machine the final state of the dial. There is also an intermediate state of the dial, after it interacts with its older self and before it is put into the time machine. We can represent the initial or intermediate states of the dial, before it goes into the time machine, as an angle \(x\) in the horizontal plane and the final state of the dial, after it comes out of the time machine, as an angle \(y\) in the vertical plane. All possible \(\langle x,y\rangle\) pairs can thus be visualized as a torus with each \(x\) value picking out a vertical circular cross-section and each \(y\) picking out a point on that cross-section. See figure 1 .
Figure 1 [An extended description of figure 1 is in the supplement.]
Suppose that the dial starts at angle \(i\) which picks out vertical circle \(I\) on the torus. The initial angle \(i\) that the dial is at before it encounters its older self, and the set of all possible final angles that the dial can have when it emerges from the time machine is represented by the circle \(I\) on the torus (see figure 1 ). Given any possible angle of the emerging dial, the dial initially at angle \(i\) will develop to some other angle. One can picture this development by rotating each point on \(I\) in the horizontal direction by the relevant amount. Since the rotation has to depend continuously on the angle of the emerging dial, circle \(I\) during this development will deform into some loop \(L\) on the torus. Loop \(L\) thus represents all possible intermediate angles \(x\) that the dial is at when it is thrown into the time machine, given that it started at angle \(i\) and then encountered a dial (its older self) which was at angle \(y\) when it emerged from the time machine. We therefore have consistency if \(x=y\) for some \(x\) and \(y\) on loop \(L\). Now, let loop \(C\) be the loop which consists of all the points on the torus for which \(x=y\). Ring \(I\) intersects \(C\) at point \(\langle i,i\rangle\). Obviously any continuous deformation of \(I\) must still intersect \(C\) somewhere. So \(L\) must intersect \(C\) somewhere, say at \(\langle j,j\rangle\). But that means that no matter how the development of the dial starting at \(I\) depends on the angle of the emerging dial, there will be some angle for the emerging dial such that the dial will develop exactly into that angle (by the time it enters the time machine) under the influence of that emerging dial. This is so no matter what angle one starts with, and no matter how the development depends on the angle of the emerging dial. Thus even for a circular state-space there are no constraints needed other than continuity.
Unfortunately there are state-spaces that escape even this argument. Consider for instance a pointer that can be set to all values between 0 and 1, where 0 and 1 are not possible values. That is, suppose that we have a state-space that is isomorphic to an open set of real numbers. Now suppose that we have a machine that sets the pointer to half the value that the pointer is set at when it emerges from the time machine.
Figure 2 [An extended description of figure 2 is in the supplement.]
Suppose the pointer starts at value \(I\). As before we can represent the combination of this initial position and all possible final positions by the line \(I\). Under the influence of the pointer coming out of the time machine the pointer value will develop to a value that equals half the value of the final value that it encountered. We can represent this development as the continuous deformation of line \(I\) into line \(L\), which is indicated by the arrows in figure 2 . This development is fully continuous. Points \(\langle x,y\rangle\) on line \(I\) represent the initial position \(x=I\) of the (young) pointer, and the position \(y\) of the older pointer as it emerges from the time machine. Points \(\langle x,y\rangle\) on line \(L\) represent the position \(x\) that the younger pointer should develop into, given that it encountered the older pointer emerging from the time machine set at position \(y\). Since the pointer is designed to develop to half the value of the pointer that it encounters, the line \(L\) corresponds to \(x=1/2 y\). We have consistency if there is some point such that it develops into that point, if it encounters that point. Thus, we have consistency if there is some point \(\langle x,y\rangle\) on line \(L\) such that \(x=y\). However, there is no such point: lines \(L\) and \(C\) do not intersect. Thus there is no consistent solution, despite the fact that the dynamics is fully continuous.
Of course if 0 were a possible value, \(L\) and \(C\) would intersect at 0. This is surprising and strange: adding one point to the set of possible values of a quantity here makes the difference between paradox and peace. One might be tempted to just add the extra point to the state-space in order to avoid problems. After all, one might say, surely no measurements could ever tell us whether the set of possible values includes that exact point or not. Unfortunately there can be good theoretical reasons for supposing that some quantity has a state-space that is open: the set of all possible speeds of massive objects in special relativity surely is an open set, since it includes all speeds up to, but not including, the speed of light. Quantities that have possible values that are not bounded also lead to counter examples to the presented fixed point argument. And it is not obvious to us why one should exclude such possibilities. So the argument that no constraints are needed is not fully general.
An interesting question of course is: exactly for which state-spaces must there be such fixed points? The arguments above depend on a well-known fixed point theorem (due to Schauder) that guarantees the existence of a fixed point for compact, convex state spaces. We do not know what subsequent extensions of this result imply regarding fixed points for a wider variety of systems, or whether there are other general results along these lines. (See Kutach 2003 for more on this issue.)
A further interesting question is whether this line of argument is sufficient to resolve Consistency (see also Dowe 2007). When they apply, these results establish the existence of a solution, such as the shade of uniform gray in the first example. But physicists routinely demand more than merely the existence of a solution, namely that solutions to the equations are stable—such that “small” changes of the initial state lead to “small” changes of the resulting trajectory. (Clarifying the two senses of “small” in this statement requires further work, specifying the relevant topology.) Stability in this sense underwrites the possibility of applying equations to real systems given our inability to fix initial states with indefinite precision. (See Fletcher 2020 for further discussion.) The fixed point theorems guarantee that for an initial state \(S_1\) there is a solution, but this solution may not be “close” to the solution for a nearby initial state, \(S'\). We are not aware of any proofs that the solutions guaranteed to exist by the fixed point theorems are also stable in this sense.
Time travel has recently been discussed quite extensively in the context of general relativity. General relativity places few constraints on the global structure of space and time. This flexibility leads to a possibility first described in print by Hermann Weyl:
Every world-point is the origin of the double-cone of the active future and the passive past [i.e., the two lobes of the light cone]. Whereas in the special theory of relativity these two portions are separated by an intervening region, it is certainly possible in the present case [i.e., general relativity] for the cone of the active future to overlap with that of the passive past; so that, in principle, it is possible to experience events now that will in part be an effect of my future resolves and actions. Moreover, it is not impossible for a world-line (in particular, that of my body), although it has a timelike direction at every point, to return to the neighborhood of a point which it has already once passed through. (Weyl 1918/1920 [1952: 274])
A time-like curve is simply a space-time trajectory such that the speed of light is never equaled or exceeded along this trajectory. Time-like curves represent possible trajectories of ordinary objects. In general relativity a curve that is everywhere timelike locally can nonetheless loop back on itself, forming a CTC. Weyl makes the point vividly in terms of the light cones: along such a curve, the future lobe of the light cone (the “active future”) intersects the past lobe of the light cone (the “passive past”). Traveling along such a curve one would never exceed the speed of light, and yet after a certain amount of (proper) time one would return to a point in space-time that one previously visited. Or, by staying close to such a CTC, one could come arbitrarily close to a point in space-time that one previously visited. General relativity, in a straightforward sense, allows time travel: there appear to be many space-times compatible with the fundamental equations of general relativity in which there are CTC’s. Space-time, for instance, could have a Minkowski metric everywhere, and yet have CTC’s everywhere by having the temporal dimension (topologically) rolled up as a circle. Or, one can have wormhole connections between different parts of space-time which allow one to enter “mouth \(A\)” of such a wormhole connection, travel through the wormhole, exit the wormhole at “mouth \(B\)” and re-enter “mouth \(A\)” again. CTCs can even arise when the spacetime is topologically \(\mathbb{R}^4\), due to the “tilting” of light cones produced by rotating matter (as in Gödel 1949’s spacetime).
General relativity thus appears to provide ample opportunity for time travel. Note that just because there are CTC’s in a space-time, this does not mean that one can get from any point in the space-time to any other point by following some future directed timelike curve—there may be insurmountable practical obstacles. In Gödel’s spacetime, it is the case that there are CTCs passing through every point in the spacetime. Yet these CTCs are not geodesics, so traversing them requires acceleration. Calculations of the minimal fuel required to travel along the appropriate curve should discourage any would-be time travelers (Malament 1984, 1985; Manchak 2011). But more generally CTCs may be confined to smaller regions; some parts of space-time can have CTC’s while other parts do not. Let us call the part of a space-time that has CTC’s the “time travel region” of that space-time, while calling the rest of that space-time the “normal region”. More precisely, the “time travel region” consists of all the space-time points \(p\) such that there exists a (non-zero length) timelike curve that starts at \(p\) and returns to \(p\). Now let us turn to examining space-times with CTC’s a bit more closely for potential problems.
In order to get a feeling for the sorts of implications that closed timelike curves can have, it may be useful to consider two simple models. In space-times with closed timelike curves the traditional initial value problem cannot be framed in the usual way. For it presupposes the existence of Cauchy surfaces, and if there are CTCs then no Cauchy surface exists. (A Cauchy surface is a spacelike surface such that every inextendable timelike curve crosses it exactly once. One normally specifies initial conditions by giving the conditions on such a surface.) Nonetheless, if the topological complexities of the manifold are appropriately localized, we can come quite close. Let us call an edgeless spacelike surface \(S\) a quasi-Cauchy surface if it divides the rest of the manifold into two parts such that
- every point in the manifold can be connected by a timelike curve to \(S\), and
- any timelike curve which connects a point in one region to a point in the other region intersects \(S\) exactly once.
It is obvious that a quasi-Cauchy surface must entirely inhabit the normal region of the space-time; if any point \(p\) of \(S\) is in the time travel region, then any timelike curve which intersects \(p\) can be extended to a timelike curve which intersects \(S\) near \(p\) again. In extreme cases of time travel, a model may have no normal region at all (e.g., Minkowski space-time rolled up like a cylinder in a time-like direction), in which case our usual notions of temporal precedence will not apply. But temporal anomalies like wormholes (and time machines) can be sufficiently localized to permit the existence of quasi-Cauchy surfaces.
Given a timelike orientation, a quasi-Cauchy surface unproblematically divides the manifold into its past (i.e., all points that can be reached by past-directed timelike curves from \(S)\) and its future (ditto mutatis mutandis ). If the whole past of \(S\) is in the normal region of the manifold, then \(S\) is a partial Cauchy surface : every inextendable timelike curve which exists to the past of \(S\) intersects \(S\) exactly once, but (if there is time travel in the future) not every inextendable timelike curve which exists to the future of \(S\) intersects \(S\). Now we can ask a particularly clear question: consider a manifold which contains a time travel region, but also has a partial Cauchy surface \(S\), such that all of the temporal funny business is to the future of \(S\). If all you could see were \(S\) and its past, you would not know that the space-time had any time travel at all. The question is: are there any constraints on the sort of data which can be put on \(S\) and continued to a global solution of the dynamics which are different from the constraints (if any) on the data which can be put on a Cauchy surface in a simply connected manifold and continued to a global solution? If there is time travel to our future, might we we able to tell this now, because of some implied oddity in the arrangement of present things?
It is not at all surprising that there might be constraints on the data which can be put on a locally space-like surface which passes through the time travel region: after all, we never think we can freely specify what happens on a space-like surface and on another such surface to its future, but in this case the surface at issue lies to its own future. But if there were particular constraints for data on a partial Cauchy surface then we would apparently need to have to rule out some sorts of otherwise acceptable states on \(S\) if there is to be time travel to the future of \(S\). We then might be able to establish that there will be no time travel in the future by simple inspection of the present state of the universe. As we will see, there is reason to suspect that such constraints on the partial Cauchy surface are non-generic. But we are getting ahead of ourselves: first let’s consider the effect of time travel on a very simple dynamics.
The simplest possible example is the Newtonian theory of perfectly elastic collisions among equally massive particles in one spatial dimension. The space-time is two-dimensional, so we can represent it initially as the Euclidean plane, and the dynamics is completely specified by two conditions. When particles are traveling freely, their world lines are straight lines in the space-time, and when two particles collide, they exchange momenta, so the collision looks like an “\(X\)” in space-time, with each particle changing its momentum at the impact. [ 2 ] The dynamics is purely local, in that one can check that a set of world-lines constitutes a model of the dynamics by checking that the dynamics is obeyed in every arbitrarily small region. It is also trivial to generate solutions from arbitrary initial data if there are no CTCs: given the initial positions and momenta of a set of particles, one simply draws a straight line from each particle in the appropriate direction and continues it indefinitely. Once all the lines are drawn, the worldline of each particle can be traced from collision to collision. The boundary value problem for this dynamics is obviously well-posed: any set of data at an instant yields a unique global solution, constructed by the method sketched above.
What happens if we change the topology of the space-time by hand to produce CTCs? The simplest way to do this is depicted in figure 3 : we cut and paste the space-time so it is no longer simply connected by identifying the line \(L-\) with the line \(L+\). Particles “going in” to \(L+\) from below “emerge” from \(L-\) , and particles “going in” to \(L-\) from below “emerge” from \(L+\).
Figure 3: Inserting CTCs by Cut and Paste. [An extended description of figure 3 is in the supplement.]
How is the boundary-value problem changed by this alteration in the space-time? Before the cut and paste, we can put arbitrary data on the simultaneity slice \(S\) and continue it to a unique solution. After the change in topology, \(S\) is no longer a Cauchy surface, since a CTC will never intersect it, but it is a partial Cauchy surface. So we can ask two questions. First, can arbitrary data on \(S\) always be continued to a global solution? Second, is that solution unique? If the answer to the first question is \(no\), then we have a backward-temporal constraint: the existence of the region with CTCs places constraints on what can happen on \(S\) even though that region lies completely to the future of \(S\). If the answer to the second question is \(no\), then we have an odd sort of indeterminism, analogous to the unwritten book: the complete physical state on \(S\) does not determine the physical state in the future, even though the local dynamics is perfectly deterministic and even though there is no other past edge to the space-time region in \(S\)’s future (i.e., there is nowhere else for boundary values to come from which could influence the state of the region).
In this case the answer to the first question is yes and to the second is no : there are no constraints on the data which can be put on \(S\), but those data are always consistent with an infinitude of different global solutions. The easy way to see that there always is a solution is to construct the minimal solution in the following way. Start drawing straight lines from \(S\) as required by the initial data. If a line hits \(L-\) from the bottom, just continue it coming out of the top of \(L+\) in the appropriate place, and if a line hits \(L+\) from the bottom, continue it emerging from \(L-\) at the appropriate place. Figure 4 represents the minimal solution for a single particle which enters the time-travel region from the left:
Figure 4: The Minimal Solution. [An extended description of figure 4 is in the supplement.]
The particle “travels back in time” three times. It is obvious that this minimal solution is a global solution, since the particle always travels inertially.
But the same initial state on \(S\) is also consistent with other global solutions. The new requirement imposed by the topology is just that the data going into \(L+\) from the bottom match the data coming out of \(L-\) from the top, and the data going into \(L-\) from the bottom match the data coming out of \(L+\) from the top. So we can add any number of vertical lines connecting \(L-\) and \(L+\) to a solution and still have a solution. For example, adding a few such lines to the minimal solution yields:
Figure 5: A Non-Minimal Solution. [An extended description of figure 5 is in the supplement.]
The particle now collides with itself twice: first before it reaches \(L+\) for the first time, and again shortly before it exits the CTC region. From the particle’s point of view, it is traveling to the right at a constant speed until it hits an older version of itself and comes to rest. It remains at rest until it is hit from the right by a younger version of itself, and then continues moving off, and the same process repeats later. It is clear that this is a global model of the dynamics, and that any number of distinct models could be generating by varying the number and placement of vertical lines.
Knowing the data on \(S\), then, gives us only incomplete information about how things will go for the particle. We know that the particle will enter the CTC region, and will reach \(L+\), we know that it will be the only particle in the universe, we know exactly where and with what speed it will exit the CTC region. But we cannot determine how many collisions the particle will undergo (if any), nor how long (in proper time) it will stay in the CTC region. If the particle were a clock, we could not predict what time it would indicate when exiting the region. Furthermore, the dynamics gives us no handle on what to think of the various possibilities: there are no probabilities assigned to the various distinct possible outcomes.
Changing the topology has changed the mathematics of the situation in two ways, which tend to pull in opposite directions. On the one hand, \(S\) is no longer a Cauchy surface, so it is perhaps not surprising that data on \(S\) do not suffice to fix a unique global solution. But on the other hand, there is an added constraint: data “coming out” of \(L-\) must exactly match data “going in” to \(L+\), even though what comes out of \(L-\) helps to determine what goes into \(L+\). This added consistency constraint tends to cut down on solutions, although in this case the additional constraint is more than outweighed by the freedom to consider various sorts of data on \({L+}/{L-}\).
The fact that the extra freedom outweighs the extra constraint also points up one unexpected way that the supposed paradoxes of time travel may be overcome. Let’s try to set up a paradoxical situation using the little closed time loop above. If we send a single particle into the loop from the left and do nothing else, we know exactly where it will exit the right side of the time travel region. Now suppose we station someone at the other side of the region with the following charge: if the particle should come out on the right side, the person is to do something to prevent the particle from going in on the left in the first place. In fact, this is quite easy to do: if we send a particle in from the right, it seems that it can exit on the left and deflect the incoming left-hand particle.
Carrying on our reflection in this way, we further realize that if the particle comes out on the right, we might as well send it back in order to deflect itself from entering in the first place. So all we really need to do is the following: set up a perfectly reflecting particle mirror on the right-hand side of the time travel region, and launch the particle from the left so that— if nothing interferes with it —it will just barely hit \(L+\). Our paradox is now apparently complete. If, on the one hand, nothing interferes with the particle it will enter the time-travel region on the left, exit on the right, be reflected from the mirror, re-enter from the right, and come out on the left to prevent itself from ever entering. So if it enters, it gets deflected and never enters. On the other hand, if it never enters then nothing goes in on the left, so nothing comes out on the right, so nothing is reflected back, and there is nothing to deflect it from entering. So if it doesn’t enter, then there is nothing to deflect it and it enters. If it enters, then it is deflected and doesn’t enter; if it doesn’t enter then there is nothing to deflect it and it enters: paradox complete.
But at least one solution to the supposed paradox is easy to construct: just follow the recipe for constructing the minimal solution, continuing the initial trajectory of the particle (reflecting it the mirror in the obvious way) and then read of the number and trajectories of the particles from the resulting diagram. We get the result of figure 6 :
Figure 6: Resolving the “Paradox”. [An extended description of figure 6 is in the supplement.]
As we can see, the particle approaching from the left never reaches \(L+\): it is deflected first by a particle which emerges from \(L-\). But it is not deflected by itself , as the paradox suggests, it is deflected by another particle. Indeed, there are now four particles in the diagram: the original particle and three particles which are confined to closed time-like curves. It is not the leftmost particle which is reflected by the mirror, nor even the particle which deflects the leftmost particle; it is another particle altogether.
The paradox gets it traction from an incorrect presupposition. If there is only one particle in the world at \(S\) then there is only one particle which could participate in an interaction in the time travel region: the single particle would have to interact with its earlier (or later) self. But there is no telling what might come out of \(L-\): the only requirement is that whatever comes out must match what goes in at \(L+\). So if you go to the trouble of constructing a working time machine, you should be prepared for a different kind of disappointment when you attempt to go back and kill yourself: you may be prevented from entering the machine in the first place by some completely unpredictable entity which emerges from it. And once again a peculiar sort of indeterminism appears: if there are many self-consistent things which could prevent you from entering, there is no telling which is even likely to materialize. This is just like the case of the unwritten book: the book is never written, so nothing determines what fills its pages.
So when the freedom to put data on \(L-\) outweighs the constraint that the same data go into \(L+\), instead of paradox we get an embarrassment of riches: many solution consistent with the data on \(S\), or many possible books. To see a case where the constraint “outweighs” the freedom, we need to construct a very particular, and frankly artificial, dynamics and topology. Consider the space of all linear dynamics for a scalar field on a lattice. (The lattice can be though of as a simple discrete space-time.) We will depict the space-time lattice as a directed graph. There is to be a scalar field defined at every node of the graph, whose value at a given node depends linearly on the values of the field at nodes which have arrows which lead to it. Each edge of the graph can be assigned a weighting factor which determines how much the field at the input node contributes to the field at the output node. If we name the nodes by the letters a , b , c , etc., and the edges by their endpoints in the obvious way, then we can label the weighting factors by the edges they are associated with in an equally obvious way.
Suppose that the graph of the space-time lattice is acyclic , as in figure 7 . (A graph is Acyclic if one can not travel in the direction of the arrows and go in a loop.)
Figure 7: An Acyclic Lattice. [An extended description of figure 7 is in the supplement.]
It is easy to regard a set of nodes as the analog of a Cauchy surface, e.g., the set \(\{a, b, c\}\), and it is obvious if arbitrary data are put on those nodes the data will generate a unique solution in the future. [ 3 ] If the value of the field at node \(a\) is 3 and at node \(b\) is 7, then its value at node \(d\) will be \(3W_{ad}\) and its value at node \(e\) will be \(3W_{ae} + 7W_{be}\). By varying the weighting factors we can adjust the dynamics, but in an acyclic graph the future evolution of the field will always be unique.
Let us now again artificially alter the topology of the lattice to admit CTCs, so that the graph now is cyclic. One of the simplest such graphs is depicted in figure 8 : there are now paths which lead from \(z\) back to itself, e.g., \(z\) to \(y\) to \(z\).
Figure 8: Time Travel on a Lattice. [An extended description of figure 8 is in the supplement.]
Can we now put arbitrary data on \(v\) and \(w\), and continue that data to a global solution? Will the solution be unique?
In the generic case, there will be a solution and the solution will be unique. The equations for the value of the field at \(x, y\), and \(z\) are:
Solving these equations for \(z\) yields
which gives a unique value for \(z\) in the generic case. But looking at the space of all possible dynamics for this lattice (i.e., the space of all possible weighting factors), we find a singularity in the case where \(1-W_{zx}W_{xz} - W_{zy}W_{yz} = 0\). If we choose weighting factors in just this way, then arbitrary data at \(v\) and \(w\) cannot be continued to a global solution. Indeed, if the scalar field is everywhere non-negative, then this particular choice of dynamics puts ironclad constraints on the value of the field at \(v\) and \(w\): the field there must be zero (assuming \(W_{vx}\) and \(W_{wy}\) to be non-zero), and similarly all nodes in their past must have field value zero. If the field can take negative values, then the values at \(v\) and \(w\) must be so chosen that \(vW_{vx}W_{xz} = -wW_{wy}W_{yz}\). In either case, the field values at \(v\) and \(w\) are severely constrained by the existence of the CTC region even though these nodes lie completely to the past of that region. It is this sort of constraint which we find to be unlike anything which appears in standard physics.
Our toy models suggest three things. The first is that it may be impossible to prove in complete generality that arbitrary data on a partial Cauchy surface can always be continued to a global solution: our artificial case provides an example where it cannot. The second is that such odd constraints are not likely to be generic: we had to delicately fine-tune the dynamics to get a problem. The third is that the opposite problem, namely data on a partial Cauchy surface being consistent with many different global solutions, is likely to be generic: we did not have to do any fine-tuning to get this result.
This third point leads to a peculiar sort of indeterminism, illustrated by the case of the unwritten book: the entire state on \(S\) does not determine what will happen in the future even though the local dynamics is deterministic and there are no other “edges” to space-time from which data could influence the result. What happens in the time travel region is constrained but not determined by what happens on \(S\), and the dynamics does not even supply any probabilities for the various possibilities. The example of the photographic negative discussed in section 2, then, seems likely to be unusual, for in that case there is a unique fixed point for the dynamics, and the set-up plus the dynamical laws determine the outcome. In the generic case one would rather expect multiple fixed points, with no room for anything to influence, even probabilistically, which would be realized. (See the supplement on
Remarks and Limitations on the Toy Models .
It is ironic that time travel should lead generically not to contradictions or to constraints (in the normal region) but to underdetermination of what happens in the time travel region by what happens everywhere else (an underdetermination tied neither to a probabilistic dynamics nor to a free edge to space-time). The traditional objection to time travel is that it leads to contradictions: there is no consistent way to complete an arbitrarily constructed story about how the time traveler intends to act. Instead, though, it appears that the more significant problem is underdetermination: the story can be consistently completed in many different ways.
Echeverria, Klinkhammer, and Thorne (1991) considered the case of 3-dimensional single hard spherical ball that can go through a single time travel wormhole so as to collide with its younger self.
Figure 9 [An extended description of figure 9 is in the supplement.]
The threat of paradox in this case arises in the following form. Consider the initial trajectory of a ball as it approaches the time travel region. For some initial trajectories, the ball does not undergo a collision before reaching mouth 1, but upon exiting mouth 2 it will collide with its earlier self. This leads to a contradiction if the collision is strong enough to knock the ball off its trajectory and deflect it from entering mouth 1. Of course, the Wheeler-Feynman strategy is to look for a “glancing blow” solution: a collision which will produce exactly the (small) deviation in trajectory of the earlier ball that produces exactly that collision. Are there always such solutions? [ 4 ]
Echeverria, Klinkhammer & Thorne found a large class of initial trajectories that have consistent “glancing blow” continuations, and found none that do not (but their search was not completely general). They did not produce a rigorous proof that every initial trajectory has a consistent continuation, but suggested that it is very plausible that every initial trajectory has a consistent continuation. That is to say, they have made it very plausible that, in the billiard ball wormhole case, the time travel structure of such a wormhole space-time does not result in constraints on states on spacelike surfaces in the non-time travel region.
In fact, as one might expect from our discussion in the previous section, they found the opposite problem from that of inconsistency: they found underdetermination. For a large class of initial trajectories there are multiple different consistent “glancing blow” continuations of that trajectory (many of which involve multiple wormhole traversals). For example, if one initially has a ball that is traveling on a trajectory aimed straight between the two mouths, then one obvious solution is that the ball passes between the two mouths and never time travels. But another solution is that the younger ball gets knocked into mouth 1 exactly so as to come out of mouth 2 and produce that collision. Echeverria et al. do not note the possibility (which we pointed out in the previous section) of the existence of additional balls in the time travel region. We conjecture (but have no proof) that for every initial trajectory of \(A\) there are some, and generically many, multiple-ball continuations.
Friedman, Morris, et al. (1990) examined the case of source-free non-self-interacting scalar fields traveling through such a time travel wormhole and found that no constraints on initial conditions in the non-time travel region are imposed by the existence of such time travel wormholes. In general there appear to be no known counter examples to the claim that in “somewhat realistic” time-travel space-times with a partial Cauchy surface there are no constraints imposed on the state on such a partial Cauchy surface by the existence of CTC’s. (See, e.g., Friedman & Morris 1991; Thorne 1994; Earman 1995; Earman, Smeenk, & Wüthrich 2009; and Dowe 2007.)
How about the issue of constraints in the time travel region \(T\)? Prima facie , constraints in such a region would not appear to be surprising. But one might still expect that there should be no constraints on states on a spacelike surface, provided one keeps the surface “small enough”. In the physics literature the following question has been asked: for any point \(p\) in \(T\), and any space-like surface \(S\) that includes \(p\) is there a neighborhood \(E\) of \(p\) in \(S\) such that any solution on \(E\) can be extended to a solution on the whole space-time? With respect to this question, there are some simple models in which one has this kind of extendability of local solutions to global ones, and some simple models in which one does not have such extendability, with no clear general pattern. The technical mathematical problems are amplified by the more conceptual problem of what it might mean to say that one could create a situation which forces the creation of closed timelike curves. (See, e.g., Yurtsever 1990; Friedman, Morris, et al. 1990; Novikov 1992; Earman 1995; and Earman, Smeenk, & Wüthrich 2009). What are we to think of all of this?
The toy models above all treat billiard balls, fields, and other objects propagating through a background spacetime with CTCs. Even if we can show that a consistent solution exists, there is a further question: what kind of matter and dynamics could generate CTCs to begin with? There are various solutions of Einstein’s equations with CTCs, but how do these exotic spacetimes relate to the models actually used in describing the world? In other words, what positive reasons might we have to take CTCs seriously as a feature of the actual universe, rather than an exotic possibility of primarily mathematical interest?
We should distinguish two different kinds of “possibility” that we might have in mind in posing such questions (following Stein 1970). First, we can consider a solution as a candidate cosmological model, describing the (large-scale gravitational degrees of freedom of the) entire universe. The case for ruling out spacetimes with CTCs as potential cosmological models strikes us as, surprisingly, fairly weak. Physicists used to simply rule out solutions with CTCs as unreasonable by fiat, due to the threat of paradoxes, which we have dismantled above. But it is also challenging to make an observational case. Observations tell us very little about global features, such as the existence of CTCs, because signals can only reach an observer from a limited region of spacetime, called the past light cone. Our past light cone—and indeed the collection of all the past light cones for possible observers in a given spacetime—can be embedded in spacetimes with quite different global features (Malament 1977, Manchak 2009). This undercuts the possibility of using observations to constrain global topology, including (among other things) ruling out the existence of CTCs.
Yet the case in favor of taking cosmological models with CTCs seriously is also not particularly strong. Some solutions used to describe black holes, which are clearly relevant in a variety of astrophysical contexts, include CTCs. But the question of whether the CTCs themselves play an essential representational role is subtle: the CTCs arise in the maximal extensions of these solutions, and can plausibly be regarded as extraneous to successful applications. Furthermore, many of the known solutions with CTCs have symmetries, raising the possibility that CTCs are not a stable or robust feature. Slight departures from symmetry may lead to a solution without CTCs, suggesting that the CTCs may be an artifact of an idealized model.
The second sense of possibility regards whether “reasonable” initial conditions can be shown to lead to, or not to lead to, the formation of CTCs. As with the toy models above, suppose that we have a partial Cauchy surface \(S\), such that all the temporal funny business lies to the future. Rather than simply assuming that there is a region with CTCs to the future, we can ask instead whether it is possible to create CTCs by manipulating matter in the initial, well-behaved region—that is, whether it is possible to build a time machine. Several physicists have pursued “chronology protection theorems” aiming to show that the dynamics of general relativity (or some other aspects of physics) rules this out, and to clarify why this is the case. The proof of such a theorem would justify neglecting solutions with CTCs as a source of insight into the nature of time in the actual world. But as of yet there are several partial results that do not fully settle the question. One further intriguing possibility is that even if general relativity by itself does protect chronology, it may not be possible to formulate a sensible theory describing matter and fields in solutions with CTCs. (See SEP entry on Time Machines; Smeenk and Wüthrich 2011 for more.)
There is a different question regarding the limitations of these toy models. The toy models and related examples show that there are consistent solutions for simple systems in the presence of CTCs. As usual we have made the analysis tractable by building toy models, selecting only a few dynamical degrees of freedom and tracking their evolution. But there is a large gap between the systems we have described and the time travel stories they evoke, with Kurt traveling along a CTC with murderous intentions. In particular, many features of the manifest image of time are tied to the thermodynamical properties of macroscopic systems. Rovelli (unpublished) considers a extremely simple system to illustrate the problem: can a clock move along a CTC? A clock consists of something in periodic motion, such as a pendulum bob, and something that counts the oscillations, such as an escapement mechanism. The escapement mechanism cannot work without friction; this requires dissipation and increasing entropy. For a clock that counts oscillations as it moves along a time-like trajectory, the entropy must be a monotonically increasing function. But that is obviously incompatible with the clock returning to precisely the same state at some future time as it completes a loop. The point generalizes, obviously, to imply that anything like a human, with memory and agency, cannot move along a CTC.
Since it is not obvious that one can rid oneself of all constraints in realistic models, let us examine the argument that time travel is implausible, and we should think it unlikely to exist in our world, in so far as it implies such constraints. The argument goes something like the following. In order to satisfy such constraints one needs some pre-established divine harmony between the global (time travel) structure of space-time and the distribution of particles and fields on space-like surfaces in it. But it is not plausible that the actual world, or any world even remotely like ours, is constructed with divine harmony as part of the plan. In fact, one might argue, we have empirical evidence that conditions in any spatial region can vary quite arbitrarily. So we have evidence that such constraints, whatever they are, do not in fact exist in our world. So we have evidence that there are no closed time-like lines in our world or one remotely like it. We will now examine this argument in more detail by presenting four possible responses, with counterresponses, to this argument.
Response 1. There is nothing implausible or new about such constraints. For instance, if the universe is spatially closed, there has to be enough matter to produce the needed curvature, and this puts constraints on the matter distribution on a space-like hypersurface. Thus global space-time structure can quite unproblematically constrain matter distributions on space-like hypersurfaces in it. Moreover we have no realistic idea what these constraints look like, so we hardly can be said to have evidence that they do not obtain.
Counterresponse 1. Of course there are constraining relations between the global structure of space-time and the matter in it. The Einstein equations relate curvature of the manifold to the matter distribution in it. But what is so strange and implausible about the constraints imposed by the existence of closed time-like curves is that these constraints in essence have nothing to do with the Einstein equations. When investigating such constraints one typically treats the particles and/or field in question as test particles and/or fields in a given space-time, i.e., they are assumed not to affect the metric of space-time in any way. In typical space-times without closed time-like curves this means that one has, in essence, complete freedom of matter distribution on a space-like hypersurface. (See response 2 for some more discussion of this issue). The constraints imposed by the possibility of time travel have a quite different origin and are implausible. In the ordinary case there is a causal interaction between matter and space-time that results in relations between global structure of space-time and the matter distribution in it. In the time travel case there is no such causal story to be told: there simply has to be some pre-established harmony between the global space-time structure and the matter distribution on some space-like surfaces. This is implausible.
Response 2. Constraints upon matter distributions are nothing new. For instance, Maxwell’s equations constrain electric fields \(\boldsymbol{E}\) on an initial surface to be related to the (simultaneous) charge density distribution \(\varrho\) by the equation \(\varrho = \text{div}(\boldsymbol{E})\). (If we assume that the \(E\) field is generated solely by the charge distribution, this conditions amounts to requiring that the \(E\) field at any point in space simply be the one generated by the charge distribution according to Coulomb’s inverse square law of electrostatics.) This is not implausible divine harmony. Such constraints can hold as a matter of physical law. Moreover, if we had inferred from the apparent free variation of conditions on spatial regions that there could be no such constraints we would have mistakenly inferred that \(\varrho = \text{div}(\boldsymbol{E})\) could not be a law of nature.
Counterresponse 2. The constraints imposed by the existence of closed time-like lines are of quite a different character from the constraint imposed by \(\varrho = \text{div}(\boldsymbol{E})\). The constraints imposed by \(\varrho = \text{div}(\boldsymbol{E})\) on the state on a space-like hypersurface are:
- local constraints (i.e., to check whether the constraint holds in a region you just need to see whether it holds at each point in the region),
- quite independent of the global space-time structure,
- quite independent of how the space-like surface in question is embedded in a given space-time, and
- very simply and generally stateable.
On the other hand, the consistency constraints imposed by the existence of closed time-like curves (i) are not local, (ii) are dependent on the global structure of space-time, (iii) depend on the location of the space-like surface in question in a given space-time, and (iv) appear not to be simply stateable other than as the demand that the state on that space-like surface embedded in such and such a way in a given space-time, do not lead to inconsistency. On some views of laws (e.g., David Lewis’ view) this plausibly implies that such constraints, even if they hold, could not possibly be laws. But even if one does not accept such a view of laws, one could claim that the bizarre features of such constraints imply that it is implausible that such constraints hold in our world or in any world remotely like ours.
Response 3. It would be strange if there are constraints in the non-time travel region. It is not strange if there are constraints in the time travel region. They should be explained in terms of the strange, self-interactive, character of time travel regions. In this region there are time-like trajectories from points to themselves. Thus the state at such a point, in such a region, will, in a sense, interact with itself. It is a well-known fact that systems that interact with themselves will develop into an equilibrium state, if there is such an equilibrium state, or else will develop towards some singularity. Normally, of course, self-interaction isn’t true instantaneous self-interaction, but consists of a feed-back mechanism that takes time. But in time travel regions something like true instantaneous self-interaction occurs. This explains why constraints on states occur in such time travel regions: the states “ ab initio ” have to be “equilibrium states”. Indeed in a way this also provides some picture of why indeterminism occurs in time travel regions: at the onset of self-interaction states can fork into different equi-possible equilibrium states.
Counterresponse 3. This is explanation by woolly analogy. It all goes to show that time travel leads to such bizarre consequences that it is unlikely that it occurs in a world remotely like ours.
Response 4. All of the previous discussion completely misses the point. So far we have been taking the space-time structure as given, and asked the question whether a given time travel space-time structure imposes constraints on states on (parts of) space-like surfaces. However, space-time and matter interact. Suppose that one is in a space-time with closed time-like lines, such that certain counterfactual distributions of matter on some neighborhood of a point \(p\) are ruled out if one holds that space-time structure fixed. One might then ask
Why does the actual state near \(p\) in fact satisfy these constraints? By what divine luck or plan is this local state compatible with the global space-time structure? What if conditions near \(p\) had been slightly different?
And one might take it that the lack of normal answers to these questions indicates that it is very implausible that our world, or any remotely like it, is such a time travel universe. However the proper response to these question is the following. There are no constraints in any significant sense. If they hold they hold as a matter of accidental fact, not of law. There is no more explanation of them possible than there is of any contingent fact. Had conditions in a neighborhood of \(p\) been otherwise, the global structure of space-time would have been different. So what? The only question relevant to the issue of constraints is whether an arbitrary state on an arbitrary spatial surface \(S\) can always be embedded into a space-time such that that state on \(S\) consistently extends to a solution on the entire space-time.
But we know the answer to that question. A well-known theorem in general relativity says the following: any initial data set on a three dimensional manifold \(S\) with positive definite metric has a unique embedding into a maximal space-time in which \(S\) is a Cauchy surface (see, e.g., Geroch & Horowitz 1979: 284 for more detail), i.e., there is a unique largest space-time which has \(S\) as a Cauchy surface and contains a consistent evolution of the initial value data on \(S\). Now since \(S\) is a Cauchy surface this space-time does not have closed time like curves. But it may have extensions (in which \(S\) is not a Cauchy surface) which include closed timelike curves, indeed it may be that any maximal extension of it would include closed timelike curves. (This appears to be the case for extensions of states on certain surfaces of Taub-NUT space-times. See Earman, Smeenk, & Wüthrich 2009). But these extensions, of course, will be consistent. So properly speaking, there are no constraints on states on space-like surfaces. Nonetheless the space-time in which these are embedded may or may not include closed time-like curves.
Counterresponse 4. This, in essence, is the stonewalling answer which we indicated in section 1. However, whether or not you call the constraints imposed by a given space-time on distributions of matter on certain space-like surfaces “genuine constraints”, whether or not they can be considered lawlike, and whether or not they need to be explained, the existence of such constraints can still be used to argue that time travel worlds are so bizarre that it is implausible that our world or any world remotely like ours is a time travel world.
Suppose that one is in a time travel world. Suppose that given the global space-time structure of this world, there are constraints imposed upon, say, the state of motion of a ball on some space-like surface when it is treated as a test particle, i.e., when it is assumed that the ball does not affect the metric properties of the space-time it is in. (There is lots of other matter that, via the Einstein equation, corresponds exactly to the curvature that there is everywhere in this time travel worlds.) Now a real ball of course does have some effect on the metric of the space-time it is in. But let us consider a ball that is so small that its effect on the metric is negligible. Presumably it will still be the case that certain states of this ball on that space-like surface are not compatible with the global time travel structure of this universe.
This means that the actual distribution of matter on such a space-like surface can be extended into a space-time with closed time-like lines, but that certain counterfactual distributions of matter on this space-like surface can not be extended into the same space-time. But note that the changes made in the matter distribution (when going from the actual to the counterfactual distribution) do not in any non-negligible way affect the metric properties of the space-time. (Recall that the changes only effect test particles.) Thus the reason why the global time travel properties of the counterfactual space-time have to be significantly different from the actual space-time is not that there are problems with metric singularities or alterations in the metric that force significant global changes when we go to the counterfactual matter distribution. The reason that the counterfactual space-time has to be different is that in the counterfactual world the ball’s initial state of motion starting on the space-like surface, could not “meet up” in a consistent way with its earlier self (could not be consistently extended) if we were to let the global structure of the counterfactual space-time be the same as that of the actual space-time. Now, it is not bizarre or implausible that there is a counterfactual dependence of manifold structure, even of its topology, on matter distributions on spacelike surfaces. For instance, certain matter distributions may lead to singularities, others may not. We may indeed in some sense have causal power over the topology of the space-time we live in. But this power normally comes via the Einstein equations. But it is bizarre to think that there could be a counterfactual dependence of global space-time structure on the arrangement of certain tiny bits of matter on some space-like surface, where changes in that arrangement by assumption do not affect the metric anywhere in space-time in any significant way . It is implausible that we live in such a world, or that a world even remotely like ours is like that.
Let us illustrate this argument in a different way by assuming that wormhole time travel imposes constraints upon the states of people prior to such time travel, where the people have so little mass/energy that they have negligible effect, via the Einstein equation, on the local metric properties of space-time. Do you think it more plausible that we live in a world where wormhole time travel occurs but it only occurs when people’s states are such that these local states happen to combine with time travel in such a way that nobody ever succeeds in killing their younger self, or do you think it more plausible that we are not in a wormhole time travel world? [ 5 ]
An alternative approach to time travel (initiated by Deutsch 1991) abstracts away from the idealized toy models described above. [ 6 ] This computational approach considers instead the evolution of bits (simple physical systems with two discrete states) through a network of interactions, which can be represented by a circuit diagram with gates corresponding to the interactions. Motivated by the possibility of CTCs, Deutsch proposed adding a new kind of channel that connects the output of a given gate back to its input —in essence, a backwards-time step. More concretely, given a gate that takes \(n\) bits as input, we can imagine taking some number \(i \lt n\) of these bits through a channel that loops back and then do double-duty as inputs. Consistency requires that the state of these \(i\) bits is the same for output and input. (We will consider an illustration of this kind of system in the next section.) Working through examples of circuit diagrams with a CTC channel leads to similar treatments of Consistency and Underdetermination as the discussion above (see, e.g., Wallace 2012: § 10.6). But the approach offers two new insights (both originally due to Deutsch): the Easy Knowledge paradox, and a particularly clear extension to time travel in quantum mechanics.
A computer equipped with a CTC channel can exploit the need to find consistent evolution to solve remarkably hard problems. (This is quite different than the first idea that comes to mind to enhance computational power: namely to just devote more time to a computation, and then send the result back on the CTC to an earlier state.) The gate in a circuit incorporating a CTC implements a function from the input bits to the output bits, under the constraint that the output and input match the i bits going through the CTC channel. This requires, in effect, finding the fixed point of the relevant function. Given the generality of the model, there are few limits on the functions that could be implemented on the CTC circuit. Nature has to solve a hard computational problem just to ensure consistent evolution. This can then be extended to other complex computational problems—leading, more precisely, to solutions of NP -complete problems in polynomial time (see Aaronson 2013: Chapter 20 for an overview and further references). The limits imposed by computational complexity are an essential part of our epistemic situation, and computers with CTCs would radically change this.
We now turn to the application of the computational approach to the quantum physics of time travel (see Deutsch 1991; Deutsch & Lockwood 1994). By contrast with the earlier discussions of constraints in classical systems, they claim to show that time travel never imposes any constraints on the pre-time travel state of quantum systems. The essence of this account is as follows. [ 7 ]
A quantum system starts in state \(S_1\), interacts with its older self, after the interaction is in state \(S_2\), time travels while developing into state \(S_3\), then interacts with its younger self, and ends in state \(S_4\) (see figure 10 ).
Figure 10 [An extended description of figure 10 is in the supplement.]
Deutsch assumes that the set of possible states of this system are the mixed states, i.e., are represented by the density matrices over the Hilbert space of that system. Deutsch then shows that for any initial state \(S_1\), any unitary interaction between the older and younger self, and any unitary development during time travel, there is a consistent solution, i.e., there is at least one pair of states \(S_2\) and \(S_3\) such that when \(S_1\) interacts with \(S_3\) it will change to state \(S_2\) and \(S_2\) will then develop into \(S_3\). The states \(S_2, S_3\) and \(S_4\) will typically be not be pure states, i.e., will be non-trivial mixed states, even if \(S_1\) is pure. In order to understand how this leads to interpretational problems let us give an example. Consider a system that has a two dimensional Hilbert space with as a basis the states \(\vc{+}\) and \(\vc{-}\). Let us suppose that when state \(\vc{+}\) of the young system encounters state \(\vc{+}\) of the older system, they interact and the young system develops into state \(\vc{-}\) and the old system remains in state \(\vc{+}\). In obvious notation:
Similarly, suppose that:
Let us furthermore assume that there is no development of the state of the system during time travel, i.e., that \(\vc{+}_2\) develops into \(\vc{+}_3\), and that \(\vc{-}_2\) develops into \(\vc{-}_3\).
Now, if the only possible states of the system were \(\vc{+}\) and \(\vc{-}\) (i.e., if there were no superpositions or mixtures of these states), then there is a constraint on initial states: initial state \(\vc{+}_1\) is impossible. For if \(\vc{+}_1\) interacts with \(\vc{+}_3\) then it will develop into \(\vc{-}_2\), which, during time travel, will develop into \(\vc{-}_3\), which inconsistent with the assumed state \(\vc{+}_3\). Similarly if \(\vc{+}_1\) interacts with \(\vc{-}_3\) it will develop into \(\vc{+}_2\), which will then develop into \(\vc{+}_3\) which is also inconsistent. Thus the system can not start in state \(\vc{+}_1\).
But, says Deutsch, in quantum mechanics such a system can also be in any mixture of the states \(\vc{+}\) and \(\vc{-}\). Suppose that the older system, prior to the interaction, is in a state \(S_3\) which is an equal mixture of 50% \(\vc{+}_3\) and 50% \(\vc{-}_3\). Then the younger system during the interaction will develop into a mixture of 50% \(\vc{+}_2\) and 50% \(\vc{-}_2\), which will then develop into a mixture of 50% \(\vc{+}_3\) and 50% \(\vc{-}_3\), which is consistent! More generally Deutsch uses a fixed point theorem to show that no matter what the unitary development during interaction is, and no matter what the unitary development during time travel is, for any state \(S_1\) there is always a state \(S_3\) (which typically is not a pure state) which causes \(S_1\) to develop into a state \(S_2\) which develops into that state \(S_3\). Thus quantum mechanics comes to the rescue: it shows in all generality that no constraints on initial states are needed!
One might wonder why Deutsch appeals to mixed states: will superpositions of states \(\vc{+}\) and \(\vc{-}\) not suffice? Unfortunately such an idea does not work. Suppose again that the initial state is \(\vc{+}_1\). One might suggest that that if state \(S_3\) is
one will obtain a consistent development. For one might think that when initial state \(\vc{+}_1\) encounters the superposition
it will develop into superposition
and that this in turn will develop into
as desired. However this is not correct. For initial state \(\vc{+}_1\) when it encounters
will develop into the entangled state
In so far as one can speak of the state of the young system after this interaction, it is in the mixture of 50% \(\vc{+}_2\) and 50% \(\vc{-}_2\), not in the superposition
So Deutsch does need his recourse to mixed states.
This clarification of why Deutsch needs his mixtures does however indicate a serious worry about the simplifications that are part of Deutsch’s account. After the interaction the old and young system will (typically) be in an entangled state. Although for purposes of a measurement on one of the two systems one can say that this system is in a mixed state, one can not represent the full state of the two systems by specifying the mixed state of each separate part, as there are correlations between observables of the two systems that are not represented by these two mixed states, but are represented in the joint entangled state. But if there really is an entangled state of the old and young systems directly after the interaction, how is one to represent the subsequent development of this entangled state? Will the state of the younger system remain entangled with the state of the older system as the younger system time travels and the older system moves on into the future? On what space-like surfaces are we to imagine this total entangled state to be? At this point it becomes clear that there is no obvious and simple way to extend elementary non-relativistic quantum mechanics to space-times with closed time-like curves: we apparently need to characterize not just the entanglement between two systems, but entanglement relative to specific spacetime descriptions.
How does Deutsch avoid these complications? Deutsch assumes a mixed state \(S_3\) of the older system prior to the interaction with the younger system. He lets it interact with an arbitrary pure state \(S_1\) younger system. After this interaction there is an entangled state \(S'\) of the two systems. Deutsch computes the mixed state \(S_2\) of the younger system which is implied by this entangled state \(S'\). His demand for consistency then is just that this mixed state \(S_2\) develops into the mixed state \(S_3\). Now it is not at all clear that this is a legitimate way to simplify the problem of time travel in quantum mechanics. But even if we grant him this simplification there is a problem: how are we to understand these mixtures?
If we take an ignorance interpretation of mixtures we run into trouble. For suppose that we assume that in each individual case each older system is either in state \(\vc{+}_3\) or in state \(\vc{-}_3\) prior to the interaction. Then we regain our paradox. Deutsch instead recommends the following, many worlds, picture of mixtures. Suppose we start with state \(\vc{+}_1\) in all worlds. In some of the many worlds the older system will be in the \(\vc{+}_3\) state, let us call them A -worlds, and in some worlds, B -worlds, it will be in the \(\vc{-}_3\) state. Thus in A -worlds after interaction we will have state \(\vc{-}_2\) , and in B -worlds we will have state \(\vc{+}_2\). During time travel the \(\vc{-}_2\) state will remain the same, i.e., turn into state \(\vc{-}_3\), but the systems in question will travel from A -worlds to B -worlds. Similarly the \(\vc{+}\) \(_2\) states will travel from the B -worlds to the A -worlds, thus preserving consistency.
Now whatever one thinks of the merits of many worlds interpretations, and of this understanding of it applied to mixtures, in the end one does not obtain genuine time travel in Deutsch’s account. The systems in question travel from one time in one world to another time in another world, but no system travels to an earlier time in the same world. (This is so at least in the normal sense of the word “world”, the sense that one means when, for instance, one says “there was, and will be, only one Elvis Presley in this world.”) Thus, even if it were a reasonable view, it is not quite as interesting as it may have initially seemed. (See Wallace 2012 for a more sympathetic treatment, that explores several further implications of accepting time travel in conjunction with the many worlds interpretation.)
We close by acknowledging that Deutsch’s starting point—the claim that this computational model captures the essential features of quantum systems in a spacetime with CTCs—has been the subject of some debate. Several physicists have pursued a quite different treatment of evolution of quantum systems through CTC’s, based on considering the “post-selected” state (see Lloyd et al. 2011). Their motivations for implementing the consistency condition in terms of the post-selected state reflects a different stance towards quantum foundations. A different line of argument aims to determine whether Deutsch’s treatment holds as an appropriate limiting case of a more rigorous treatment, such as quantum field theory in curved spacetimes. For example, Verch (2020) establishes several results challenging the assumption that Deutsch’s treatment is tied to the presence of CTC’s, or that it is compatible with the entanglement structure of quantum fields.
What remains of the grandfather paradox in general relativistic time travel worlds is the fact that in some cases the states on edgeless spacelike surfaces are “overconstrained”, so that one has less than the usual freedom in specifying conditions on such a surface, given the time-travel structure, and in some cases such states are “underconstrained”, so that states on edgeless space-like surfaces do not determine what happens elsewhere in the way that they usually do, given the time travel structure. There can also be mixtures of those two types of cases. The extent to which states are overconstrained and/or underconstrained in realistic models is as yet unclear, though it would be very surprising if neither obtained. The extant literature has primarily focused on the problem of overconstraint, since that, often, either is regarded as a metaphysical obstacle to the possibility time travel, or as an epistemological obstacle to the plausibility of time travel in our world. While it is true that our world would be quite different from the way we normally think it is if states were overconstrained, underconstraint seems at least as bizarre as overconstraint. Nonetheless, neither directly rules out the possibility of time travel.
If time travel entailed contradictions then the issue would be settled. And indeed, most of the stories employing time travel in popular culture are logically incoherent: one cannot “change” the past to be different from what it was, since the past (like the present and the future) only occurs once. But if the only requirement demanded is logical coherence, then it seems all too easy. A clever author can devise a coherent time-travel scenario in which everything happens just once and in a consistent way. This is just too cheap: logical coherence is a very weak condition, and many things we take to be metaphysically impossible are logically coherent. For example, it involves no logical contradiction to suppose that water is not molecular, but if both chemistry and Kripke are right it is a metaphysical impossibility. We have been interested not in logical possibility but in physical possibility. But even so, our conditions have been relatively weak: we have asked only whether time-travel is consistent with the universal validity of certain fundamental physical laws and with the notion that the physical state on a surface prior to the time travel region be unconstrained. It is perfectly possible that the physical laws obey this condition, but still that time travel is not metaphysically possible because of the nature of time itself. Consider an analogy. Aristotle believed that water is homoiomerous and infinitely divisible: any bit of water could be subdivided, in principle, into smaller bits of water. Aristotle’s view contains no logical contradiction. It was certainly consistent with Aristotle’s conception of water that it be homoiomerous, so this was, for him, a conceptual possibility. But if chemistry is right, Aristotle was wrong both about what water is like and what is possible for it. It can’t be infinitely divided, even though no logical or conceptual analysis would reveal that.
Similarly, even if all of our consistency conditions can be met, it does not follow that time travel is physically possible, only that some specific physical considerations cannot rule it out. The only serious proof of the possibility of time travel would be a demonstration of its actuality. For if we agree that there is no actual time travel in our universe, the supposition that there might have been involves postulating a substantial difference from actuality, a difference unlike in kind from anything we could know if firsthand. It is unclear to us exactly what the content of possible would be if one were to either maintain or deny the possibility of time travel in these circumstances, unless one merely meant that the possibility is not ruled out by some delineated set of constraints. As the example of Aristotle’s theory of water shows, conceptual and logical “possibility” do not entail possibility in a full-blooded sense. What exactly such a full-blooded sense would be in case of time travel, and whether one could have reason to believe it to obtain, remain to us obscure.
- Aaronson, Scott, 2013, Quantum Computing since Democritus , Cambridge: Cambridge University Press. doi:10.1017/CBO9780511979309
- Arntzenius, Frank, 2006, “Time Travel: Double Your Fun”, Philosophy Compass , 1(6): 599–616. doi:10.1111/j.1747-9991.2006.00045.x
- Clarke, C.J.S., 1977, “Time in General Relativity” in Foundations of Space-Time Theory , Minnesota Studies in the Philosophy of Science , Vol VIII, Earman, J., Glymour, C., and Stachel, J. (eds), pp. 94–108. Minneapolis: University of Minnesota Press.
- Deutsch, David, 1991, “Quantum Mechanics near Closed Timelike Lines”, Physical Review D , 44(10): 3197–3217. doi:10.1103/PhysRevD.44.3197
- Deutsch, David and Michael Lockwood, 1994, “The Quantum Physics of Time Travel”, Scientific American , 270(3): 68–74. doi:10.1038/scientificamerican0394-68
- Dowe, Phil, 2007, “Constraints on Data in Worlds with Closed Timelike Curves”, Philosophy of Science , 74(5): 724–735. doi:10.1086/525617
- Earman, John, 1972, “Implications of Causal Propagation Outside the Null Cone”, Australasian Journal of Philosophy , 50(3): 222–237. doi:10.1080/00048407212341281
- Earman, John, 1995, Bangs, Crunches, Whimpers, and Shrieks: Singularities and Acausalities in Relativistic Spacetimes , New York: Oxford University Press.
- Earman, John, Christopher Smeenk, and Christian Wüthrich, 2009, “Do the Laws of Physics Forbid the Operation of Time Machines?”, Synthese , 169(1): 91–124. doi:10.1007/s11229-008-9338-2
- Echeverria, Fernando, Gunnar Klinkhammer, and Kip S. Thorne, 1991, “Billiard Balls in Wormhole Spacetimes with Closed Timelike Curves: Classical Theory”, Physical Review D , 44(4): 1077–1099. doi:10.1103/PhysRevD.44.1077
- Effingham, Nikk, 2020, Time Travel: Probability and Impossibility , Oxford: Oxford University Press. doi:10.1093/oso/9780198842507.001.0001
- Fletcher, Samuel C., 2020, “The Principle of Stability”, Philosopher’s Imprint , 20: article 3. [ Fletcher 2020 available online ]
- Friedman, John and Michael Morris, 1991, “The Cauchy Problem for the Scalar Wave Equation Is Well Defined on a Class of Spacetimes with Closed Timelike Curves”, Physical Review Letters , 66(4): 401–404. doi:10.1103/PhysRevLett.66.401
- Friedman, John, Michael S. Morris, Igor D. Novikov, Fernando Echeverria, Gunnar Klinkhammer, Kip S. Thorne, and Ulvi Yurtsever, 1990, “Cauchy Problem in Spacetimes with Closed Timelike Curves”, Physical Review D , 42(6): 1915–1930. doi:10.1103/PhysRevD.42.1915
- Geroch, Robert and Gary Horowitz, 1979, “Global Structures of Spacetimes”, in General Relativity: An Einstein Centenary Survey , Stephen Hawking and W. Israel (eds.), Cambridge/New York: Cambridge University Press, Chapter 5, pp. 212–293.
- Gödel, Kurt, 1949, “A Remark About the Relationship Between Relativity Theory and Idealistic Philosophy”, in Albert Einstein, Philosopher-Scientist , Paul Arthur Schilpp (ed.), Evanston, IL: Library of Living Philosophers, 557–562.
- Hocking, John G. and Gail S. Young, 1961, Topology , (Addison-Wesley Series in Mathematics), Reading, MA: Addison-Wesley.
- Horwich, Paul, 1987, “Time Travel”, in his Asymmetries in Time: Problems in the Philosophy of Science , , Cambridge, MA: MIT Press, 111–128.
- Kutach, Douglas N., 2003, “Time Travel and Consistency Constraints”, Philosophy of Science , 70(5): 1098–1113. doi:10.1086/377392
- Lewis, David, 1976, “The Paradoxes of Time Travel”, American Philosophical Quarterly , 13(2): 145–152.
- Lloyd, Seth, Lorenzo Maccone, Raul Garcia-Patron, Vittorio Giovannetti, and Yutaka Shikano, 2011, “Quantum Mechanics of Time Travel through Post-Selected Teleportation”, Physical Review D , 84(2): 025007. doi:10.1103/PhysRevD.84.025007
- Malament, David B., 1977, “Observationally Indistinguishable Spacetimes: Comments on Glymour’s Paper”, in Foundations of Space-Time Theories , John Earman, Clark N. Glymour, and John J. Stachel (eds.), (Minnesota Studies in the Philosophy of Science 8), Minneapolis, MN: University of Minnesota Press, 61–80.
- –––, 1984, “‘Time Travel’ in the Gödel Universe”, PSA: Proceedings of the Biennial Meeting of the Philosophy of Science Association , 1984(2): 91–100. doi:10.1086/psaprocbienmeetp.1984.2.192497
- –––, 1985, “Minimal Acceleration Requirements for ‘Time Travel’, in Gödel Space‐time”, Journal of Mathematical Physics , 26(4): 774–777. doi:10.1063/1.526566
- Manchak, John Byron, 2009, “Can We Know the Global Structure of Spacetime?”, Studies in History and Philosophy of Science Part B: Studies in History and Philosophy of Modern Physics , 40(1): 53–56. doi:10.1016/j.shpsb.2008.07.004
- –––, 2011, “On Efficient ‘Time Travel’ in Gödel Spacetime”, General Relativity and Gravitation , 43(1): 51–60. doi:10.1007/s10714-010-1068-3
- Maudlin, Tim, 1990, “Time-Travel and Topology”, PSA: Proceedings of the Biennial Meeting of the Philosophy of Science Association , 1990(1): 303–315. doi:10.1086/psaprocbienmeetp.1990.1.192712
- Novikov, I. D., 1992, “Time Machine and Self-Consistent Evolution in Problems with Self-Interaction”, Physical Review D , 45(6): 1989–1994. doi:10.1103/PhysRevD.45.1989
- Smeenk, Chris and Christian Wüthrich, 2011, “Time Travel and Time Machines”, in the Oxford Handbook on Time , Craig Callender (ed.), Oxford: Oxford University Press, 577–630..
- Stein, Howard, 1970, “On the Paradoxical Time-Structures of Gödel”, Philosophy of Science , 37(4): 589–601. doi:10.1086/288328
- Thorne, Kip S., 1994, Black Holes and Time Warps: Einstein’s Outrageous Legacy , (Commonwealth Fund Book Program), New York: W.W. Norton.
- Verch, Rainer, 2020, “The D-CTC Condition in Quantum Field Theory”, in Progress and Visions in Quantum Theory in View of Gravity , Felix Finster, Domenico Giulini, Johannes Kleiner, and Jürgen Tolksdorf (eds.), Cham: Springer International Publishing, 221–232. doi:10.1007/978-3-030-38941-3_9
- Wallace, David, 2012, The Emergent Multiverse: Quantum Theory According to the Everett Interpretation , Oxford: Oxford University Press. doi:10.1093/acprof:oso/9780199546961.001.0001
- Wasserman, Ryan, 2018, Paradoxes of Time Travel , Oxford: Oxford University Press. doi:10.1093/oso/9780198793335.001.0001
- Weyl, Hermann, 1918/1920 [1922/1952], Raum, Zeit, Materie , Berlin: Springer; fourth edition 1920. Translated as Space—Time—Matter , Henry Leopold Brose (trans.), New York: Dutton, 1922. Reprinted 1952, New York: Dover Publications.
- Wheeler, John Archibald and Richard Phillips Feynman, 1949, “Classical Electrodynamics in Terms of Direct Interparticle Action”, Reviews of Modern Physics , 21(3): 425–433. doi:10.1103/RevModPhys.21.425
- Yurtsever, Ulvi, 1990, “Test Fields on Compact Space‐times”, Journal of Mathematical Physics , 31(12): 3064–3078. doi:10.1063/1.528960
How to cite this entry . Preview the PDF version of this entry at the Friends of the SEP Society . Look up topics and thinkers related to this entry at the Internet Philosophy Ontology Project (InPhO). Enhanced bibliography for this entry at PhilPapers , with links to its database.
- Adlam, Emily, unpublished, “ Is There Causation in Fundamental Physics? New Insights from Process Matrices and Quantum Causal Modelling ”, 2022, arXiv: 2208.02721. doi:10.48550/ARXIV.2208.02721
- Rovelli, Carlo, unpublished, “ Can We Travel to the Past? Irreversible Physics along Closed Timelike Curves ”, arXiv: 1912.04702. doi:10.48550/ARXIV.1912.04702
causation: backward | determinism: causal | quantum mechanics | quantum mechanics: retrocausality | space and time: being and becoming in modern physics | time machines | time travel
Copyright © 2023 by Christopher Smeenk < csmeenk2 @ uwo . ca > Frank Arntzenius Tim Maudlin
- Accessibility
Support SEP
Mirror sites.
View this site from another server:
- Info about mirror sites
The Stanford Encyclopedia of Philosophy is copyright © 2023 by The Metaphysics Research Lab , Department of Philosophy, Stanford University
Library of Congress Catalog Data: ISSN 1095-5054
A history of time travel: the how, the why and the when of turning back the clock
Pop on Aqua's 'Turn Back Time' and settle in
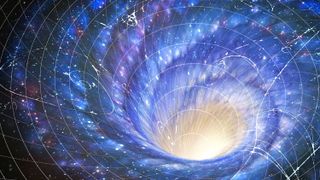
For most of human history, the world didn’t change very quickly. Until the 1700s, kids could largely expect their lives to be similar to their parents, and that their children would have an experience very similar to their own, too. There were obviously changes in how humans lived over longer stretches of time, but nothing that even different generations could easily observe.
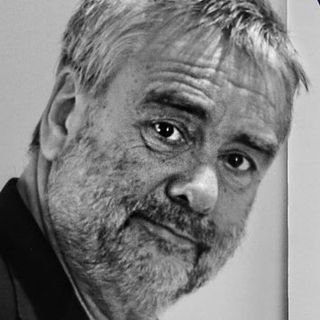
My first introduction to science fiction was Valérian and Laureline. I was ten years old. Every Wednesday there was a magazine called Pilote in France, and there was two pages of Valerian every week. It was the first time I’d seen a girl and a guy in space, agents travelling in time and space. That was amazing.
The past is written. The present? We have to deal with it. But the future is a white page. So I don’t understand why people on this white page are putting all this darkness.
God! Let’s have some color! Let’s have some fun! Let’s at least imagine a better world. Maybe we won’t be able to do it, but we have to try.
The industrial revolution changed all of this. For the first time in human history, the pace of technological change was visible within a human lifespan.
It is not a coincidence that it was only after science and technological change became a normal part of the human experience, that time travel became something we dreamed of.
Time travel is actually somewhat unique in science fiction. Many core concepts have their origins earlier in history.
The historical roots of the concept of a 'robot' can be seen in Jewish folklore for example: Golems were anthropomorphic beings sculpted from clay. In Greek mythology, characters would travel to other worlds, and it's no coincidence that The Matrix features a character called Persephone. But time travel is different.
The first real work to envisage travelling in time was The Time Machine by HG Wells, which was published in 1895.
The book tells the story of a scientist who builds a machine that will take him to the year 802,701 - a world in which ape-like Morlocks are evolutionary descendants of humanity, and have regressed to a primitive lifestyle.
Get daily insight, inspiration and deals in your inbox
Get the hottest deals available in your inbox plus news, reviews, opinion, analysis and more from the TechRadar team.
The book was a product of its time - both in terms of the science played upon (Charles Darwin had only published Origin of the Species 35 years earlier), and the racist attitudes: it is speculated that the Morlocks were inspired by the Morlachs, a real ethnic group in the Balkans who were often characterised as “primitive”.
Real science
But of course, this was science fiction - what about science fact? The two have always been closely linked, and during the early days it was no different. In 1907, the physicist Hermann Minkowski first argued that Einstein’s Special Relativity could be expressed in geometric terms as a fourth dimension (to add to our known three) - which is exactly how Wells visualised time travel in his work of fiction.
The development of Special and then General Relativity was significant as it provided the theoretical backbone for how time travel could be conceived in scientific terms. In 1949 Kurt Gödel took Einstein’s work and came up with a solution which as a mathematical necessity included what he called “closed timelike curves” - the idea that if you travel far enough, time will loop back around (like how if you keep flying East, you’ll eventually end up back where you started).
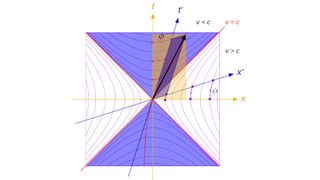
In other words, using what became known as the Gödel Metric, it is theoretically possible to travel between any one point in time and space and any other.
There was just one problem: for Gödel’s theory to be right, the universe would have to be spinning - and scientists don’t believe that it is. So while the maths might make sense, Gödel’s universe does not appear to be the one we’re actually living in. Though he never gave up hope that he might be right: Apparently even on this deathbed, he would ask if anyone has found evidence of a spinning universe. And if he does ever turn out to be right, it means that time travel can happen, and is actually fairly straightforward (well, as far as physics goes anyway).
Since Gödel, scientists have continued to hypothesise about time travel, with perhaps the best known example being tachyons - or particles that move faster than the speed of light (therefore, effectively travelling in time). So far, despite one false alarm at CERN in 2011, there is no evidence that they actually exist.
Chancers and hoaxes
Of course, the lack of real science when it comes to time travel has not stopped some people from claiming to have done it. With the likes of Marty McFly and Doctor Who on the brain, chancers and hoaxers have realised that time travel is immediately a compelling prospect. Here’s a couple of amusing examples.
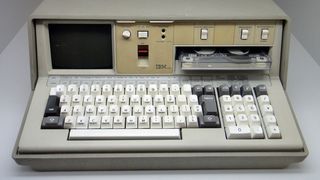
At the turn of the millennium, when the internet was still in its infancy, forums were captivated by the story of John Titor. Titor claimed he was from the year 2036, and had been sent back in time by the government to obtain an IBM 5100 computer. The thinking appeared to be that by obtaining the computer, the government could find a solution to the UNIX 2038 bug - in which clocks could be reset, Millennium Bug-style, leading to chaos everywhere.
Posting on the 'Time Travel Institute' forums, Titor went into details on how his time machine worked: It was powered by “two top-spin, dual positive singularities”, and used an X-ray venting system. He also gave a potted history of what humanity could expect: A new American civil war in 2004, and World War III in 2015. He also claimed the “many worlds” interpretation of quantum physics was true, hence why he wasn’t violating the so-called “grandfather paradox”.
Titor claimed he was from the year 2036, and had been sent back in time by the government to obtain an IBM 5100 computer.
Okay, so he probably wasn’t a real time traveller, but in the early days of the internet, when anonymity was more commonplace, he truly captured the imaginations of nerdy early adopters who perhaps, just a little bit, hoped that he might be the real thing.
More recently, in 2013, an Iranian scientist named Ali Razeghi claimed to have invented a time machine of sorts. It was supposedly capable of predicting the next 5-8 years for an individual, with up to 98% accuracy. According to The Telegraph , Razeghi said the invention fits into the size of a standard PC case and “It will not take you into the future, it will bring the future to you”. The idea is that the Iranian government could use it to predict future security threats and military confrontations. So perhaps it is time to check in and see if he managed to predict Donald Trump?
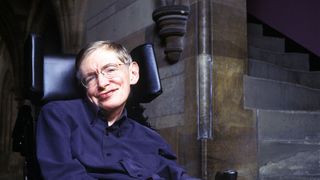
So is this the best we can do? Will we ever manage to crack time travel? Some scientists are still sceptical that it could ever be possible. This includes Stephen Hawking, who proposed the 'Chronology Protection Conjecture' – which is what it sounds like. Essentially, he argues that the laws of physics are as they are to specifically make time travel impossible – on all but “submicroscopic” scales. Essentially, this is to protect how causality works, as if we are suddenly allowed to travel back and kill our grandfathers, it would create massive time paradoxes.
Hawking revealed to Ars Technica in 2012 how he had held a party for time travellers, but only sent out invitations after the date it was held. So did the party support his argument that time travel is impossible? Or did he end up spending the evening in the company of John Titor and Doctor Who?
“I sat there a long time, but no one came”, he said, much to our disappointment.
Huge thanks to Stephen Jorgenson-Murray for walking us through some of the more brain-mangling science for this article.

To celebrate the release of Valerian and the City of a Thousand Planets , Luc Besson is today behind the lens at TechRadar. Here’s what we’ve got in store for you:
- Luc Besson presents TechRadar
- From Verne to Valerian: how France became the home of sci-fi
- Luc Besson talks streaming, viral videos and cinema tech
- Star spangled glamour: making space travel cooler than ever before
- A history of time travel: the how, the why and the when
- 20 best sci-fi films on Netflix and Amazon Prime
- Amazing future tech from sci-fi films that totally exist now
Valerian and the City of a Thousand Planets is released in UK cinemas August 2nd, and is out now in the US.
Best Buy's huge 3-day sale just went live - shop the 15 best tech deals from $59.99
eBay’s refurbished tech revolution – a quest to prevent e-waste and save you money
Paleblue Earth batteries eliminate the one big pain point of rechargeables
Most Popular
- 2 NYT Strands today — hints, answers and spangram for Thursday, April 25 (game #53)
- 3 Amazon has a ton of cheap tech gadgets on sale – I've found the 13 best ones
- 4 Meta’s massive OS announcement is more exciting than a Meta Quest 4 reveal, and VR will never be the same again
- 5 Fallout 4 current-gen update drops today with a performance and quality mode
- 2 Sony dropped OLED for its flagship 2024 TV – here's why
- 3 Sony’s wearable air conditioner is the first step towards a real Dune stillsuit
- 4 This Android phone for audiophiles offers a hi-res DAC, balanced output and 3.5mm jack – plus a cool cyberpunk look that puts Google and OnePlus to shame
- 5 Sony merging with Paramount Plus could be bad news for Netflix – here’s why
Engineers Garage
Time Travel: Theories, Possibilities, and Paradoxes Explained
By Neha Rastogi
Time Travel has been a matter of great interest for Science fiction since ages. Whether it’s the movies like Planet of the Apes (1968) or modern franchises like “Doctor Who” and “Star Trek” ; the concept is grabbing a lot of eyeballs. Not only movies and shows but even some mythological tales like Mahabharata and the Japanese story of Urashima Taro support the evidence that time travel exists. We often see stories where characters use time machines to jaunt through the years but the reality is far more complex and inexplicable.
Understanding the Concept of Time Travel
Time Travel is defined as the phenomenon of moving between different points in time through a hypothetical device called “Time Machine”. Despite being predominantly related to the field of philosophy and fiction, it’s somehow supported to a small extent by physics in conjunction with quantum mechanics. However, before getting into the argument of how real it is, let’s comprehend the fundamental meaning of time.
Basically, the whole idea of Time Travel is administered by the concept of time. Usually, people believe that time is constant but the famous Physicist Albert Einstein introduced the “Theory of Relativity” as per which, time is relative. In other words, time slows down or speeds up depending on how fast the observer moves relative to something else. According to him, a person traveling inside a spaceship at the speed of light would age much slower than his/her twin back at home.
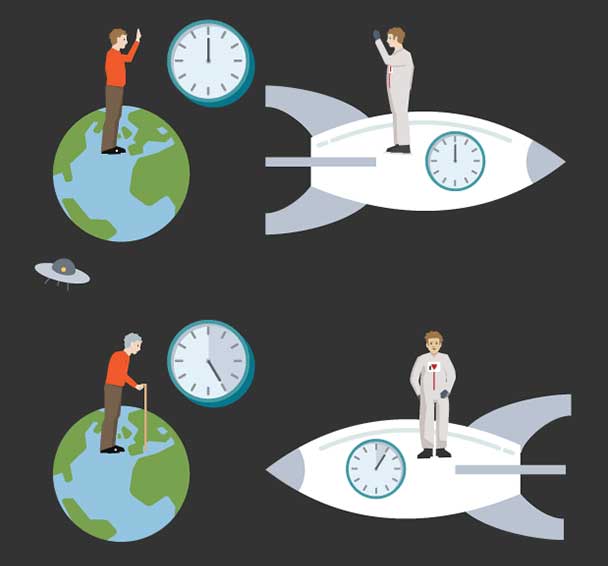
Time is Relative
After Einstein’s Theory of Relativity, his teacher Herman Minkowski emphasized on space-time, a mathematical model that joins both space and time in a continuum. This implies that time and space cannot exist without each other. Space is a 3-dimensional arena consisting of length, width, and height. This is joined by Time with the fourth dimension called direction. So anything that happens in the universe takes place in this space-time continuum. Although this validates that space travelers are slightly younger than their twins when they return to earth, yet a huge leap in the past or future is not possible with the current technology.
Time Machines
It is believed that in order to travel back or forward in time, one would require a device called Time Machine . The research on such a device would involve bending space-time to such an extent that time lines turn back on themselves to form a loop, which is termed as “closed time-like curve.” Such an action demands the use of an exotic form of matter with “negative energy density” that has a unique property of moving in the opposite direction of the normal matter when pushed. Even if it exists, the quantity would be too small to construct a machine.
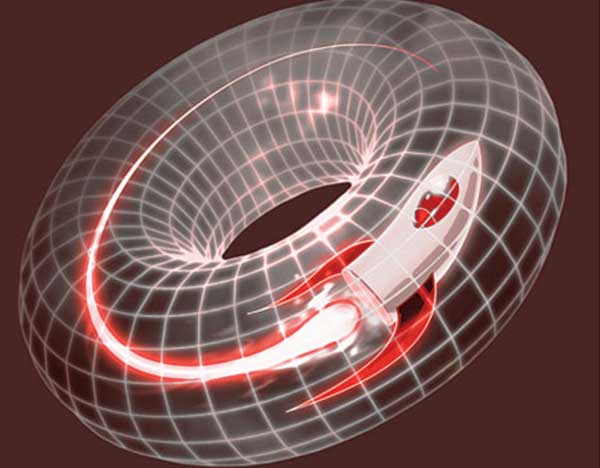
Pictorial Representation of Time Travel through closed time-like curve
However, some another research suggests that time machines can also be constructed by building a doughnut-shaped hole enveloped within a sphere of normal matter. Inside this doughnut-shaped hole filled with vacuum, gravitational force can be used to bend the space-time so as to form a closed time-like curve. After racing around inside this doughnut a traveler would be able to go back in time with each lap. But in reality, it’s quite complex because the gravitational fields have to be very strong and would demand precise manipulation.
Time Travel Approaches in Physics
After studying and researching about Time Travel, various physicists have come up with approaches that may support its possibility, at least theoretically. Let’s take a look at these concepts so as to understand how Time Travel could actually work someday.
Time Dilation
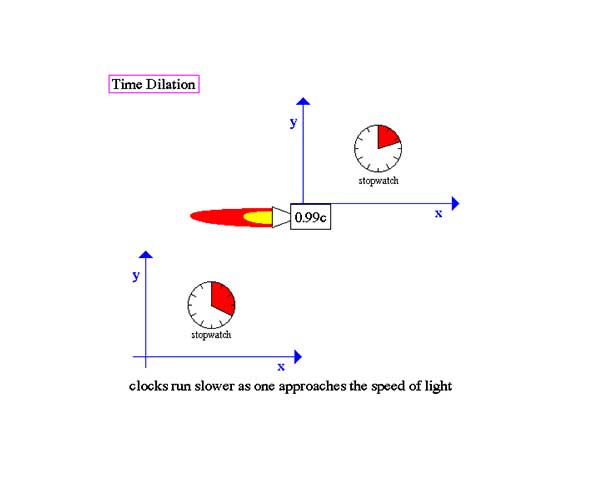
Time Dilation Explanation
An important aspect of Einstein’s relativity theory is the term “time dilation” , which is defined as the difference of elapsed time between two events as measured by observers who are either moving relative to each other or are situated at different locations from the gravitational mass. As per the theory, time dilation can be summarized as a phenomenon which occurs due to the difference in either gravity or relative velocity.
In special relativity the time dilation effect is reciprocal i.e. when two clocks are in motion with respect to each other, for both the observers, the other one will be time dilated or the other clock will move slower. However, in general relativity, an observer at the top of the tower will find the clock closer to the ground to be slower and the other observer would agree about the direction and magnitude of this difference.
Due to the concept of time dilation, the current human time travel record is held by Russian cosmonaut Sergei Krikalev . Owing to the high-speed (7.66 km/s) of ISS and the length of time spent in space, it is believed that the cosmonaut actually arrived 0.02 seconds in the future while returning to the earth.
Cosmic String
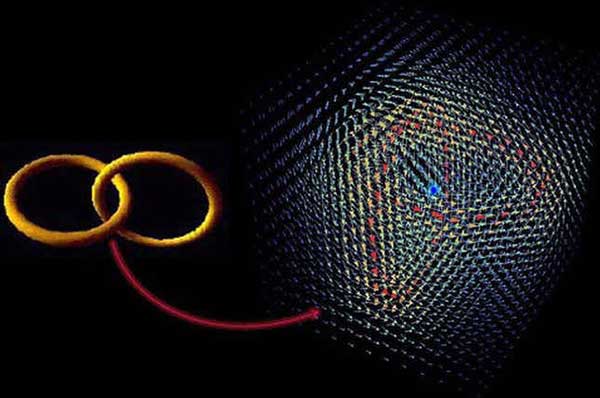
Diagram Depicting Cosmic Strings
In 1991 J Richard Gott gave the idea of Cosmic Strings , which are believed to be left over from the early cosmos. These are defined as string-like objects or narrow tubes of energy that are stretched across the entire length of the universe. Owing to the huge amount of mass and massive gravitational pull, it would allow objects attached to the Cosmic Strings to travel at the speed of light.
So if two strings are pulled close to each other or one of them is stretched near the black hole, it might warp space-time to such an extent that would lead to creating a closed time-like curve and hence leading to the possibility of time travel. Theoretically, the gravity generated by these two Cosmic strings would help in propelling a spaceship into the past.
However, coming to the reality, the loop of strings is required to contain half the mass-energy of an entire galaxy so as to travel one year back in time. This implies that powering a time machine would require splitting half the atoms present in the whole galaxy.
Black holes
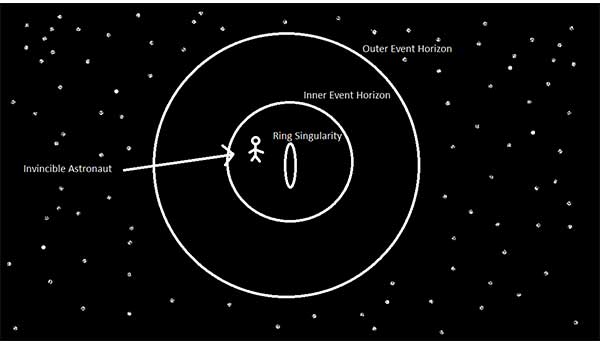
Illustration of Kerr Hole
When stars (having a mass of more than four times our sun) reach their end of life and all their fuel is burned up, they collapse under the pressure of their own weight creating “Black Holes” . The boundary of a Black Hole, called Event Horizon , has such a strong gravitational pull that it doesn’t even allow light to pass through it. Since light travels at the fastest speed, everything else traveling through a black hole is also dragged back. Such a non-rotating black hole is named as Schwarzschild black hole .
However, traveling to a parallel universe is possible through a rotating black hole named Kerr Hole . It was proposed in 1963 by a mathematician named Roy Kerr . As per his theory, if dying stars collapse into a rotating ring of neutron stars, that would produce enough centrifugal force to prevent the formation of singularity.
Note: Singularity can be perceived as the point into which the black hole tapers much like an ice-cream cone. At this point, the laws of Physics cease to exist and all the matter is crushed beyond recognition.
Since there will be no singularity, it would be safe to pass through a black hole without being crushed and exit out of a “White Hole” . A white hole is believed to be the exhaust end of a black hole which pushes everything away from it. Hence we may travel into another time or even another universe.
Although Kerr Holes are just theoretical, if they exist then we may find our way to a one-way trip to the past or future. However, physicist Kip Thorne believes that such a black hole doesn’t exist and it would suck everything before someone even reaches the Singularity.
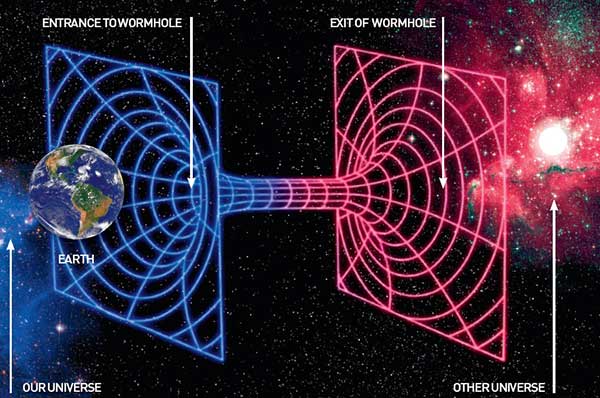
Diagrammatic Representation of Wormhole
Wormholes, also known as Einstein-Rosen Bridges , are believed to be the most potential means for time travel. It could allow us to travel several light years from earth and in much less time as compared to the conventional space travel methods. The possibility of wormholes is based on Einstein’s theory of relativity which says that any mass curves space-time. The following example is used to explain this curvature.
If two persons are holding a bed sheet stretching it tight and a baseball is placed on the sheet, its weight will make it roll to the middle of the sheet creating a curve at that point. Now if a marble is placed on the sheet, it would travel towards the baseball because of the curve. Here space is depicted as a two-dimensional plane than the four dimensions that actually makes up space-time.
Now if this sheet is folded over leaving a space at the top and bottom, placing the baseball on the top would form a curvature. If an equal mass is placed at the bottom part at a point corresponding to the location of the baseball, the second mass would eventually meet with the baseball. Similarly, wormholes might develop.
In space, masses that place pressure on different parts of the universe combine together to form a tunnel. Theoretically, this tunnel joins two separate times and allows passage between them. However, it’s possible that certain unforeseen physical properties may prevent the occurrence of wormholes and even if they exist, these might be really unstable.
Possibly someday human may learn to capture, stabilize and enlarge these tunnels but according to Dr. Hawking, prolonging the life of a tunnel through folded space-time may lead to a radiation feedback loop destroying the time tunnel.
Time Travel Paradoxes
If we ever work out a theory for time travel, we would give way to certain complexities known as paradoxes. A paradox is something that contradicts itself. In other words, time travel is not believed to be a practical concept because of certain situations that are likely to arise as the after-effects. These are broadly classified as -:
1. Closed Casual Loops: The cause and effect run in a circle causing a loop and is also internally consistent with the timeline’s history.
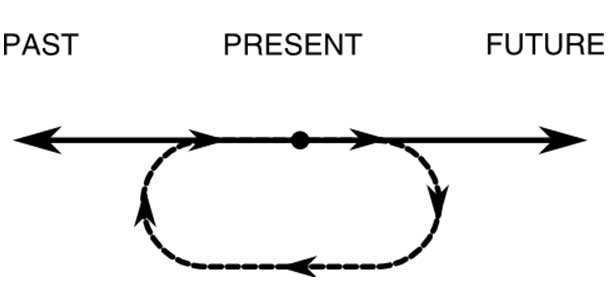
Diagram depicting time loop
• Predestination Paradox
It is defined as a situation when a traveler going back in time causes the event which he is trying to prevent from happening. It implies that any attempt to stop any event from occurring in the past would simply lead to the cause itself. The paradox suggests that things are destined to turn out the way they have happened and anyone attempting to change the past would find himself trapped in the repeating loop of time. For example, if you travel in the past to prevent your lover from dying in a road accident, you will find out that you were the one who accidentally ran over her.
• Bootstrap Paradox
A bootstrap paradox, also known as an Ontological Paradox where an object, person, or piece of information sent back in time leads to an infinite loop where the object has no discernible origin and is believed to exist without ever being created. It implies that the past, present and future and not defined, thus making it complicated to pinpoint the origin of anything. It raises questions like how were the objects created and by whom.
2. Consistency Paradox: It generates a number of timeline inconsistencies related to the possibility of altering the past. It can be further divided into the following categories.
• The Grandfather Paradox
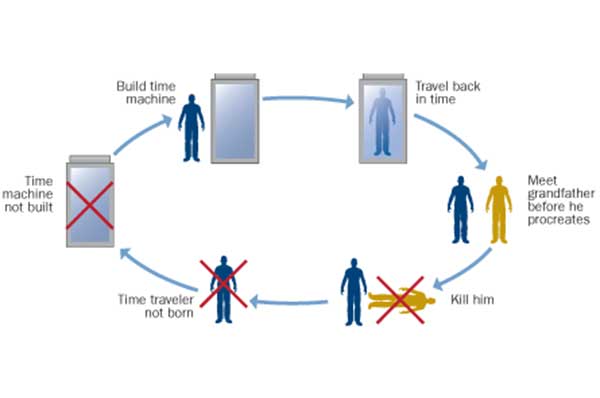
Grandfather Paradox
This paradox talks about a hypothetical situation where a person travels back in time and kills his paternal grandfather at the time when his grandfather didn’t even meet his grandmother. In such a situation, his father would never have been born and neither would the traveler himself. So if he was never born, how would he travel to the past to kill his grandfather?
The paradox also talks about auto-infanticide where a time traveler goes into the past to kill himself when he was an infant. Now if he killed himself when he was a kid, how would he exist in the future to come back in time? Some physicists say that you would be able to go back in time but you won’t be able to change it, while others suggest that you would be born in one universe but unborn in another universe.
• The Hitler Paradox
Similar to the grandfather paradox, the killing Hitler paradox erases the reason for which you would want to go into the past and kill Hitler. Moreover, killing grandfather might have a “butterfly effect” but killing Hitler would have a far-reaching impact on the History as it would change the whole course of events. If you were successful in killing Hitler, there’d be no reason that would make you want to go back in time and kill him.
This paradox has been explained very well in a Twilight Zone episode called “Cradle of Darkness” as well as an episode “Let’s Kill Her” from Dr. Who.
• Polchinski’s Paradox
American physicist Joseph Polchinski proposed a paradox where a billiard ball enters a wormhole and emerges out of the other end in the past just in time to collide with its younger version and prevents it from entering the wormhole in the first place. While proposing this scenario, Joseph had Novikov’s Self Consistency Principle in his mind which states that time travel is possible but time paradoxes are forbidden.
A number of solutions have been suggested to avoid these inconsistencies like the billiard ball will deliver a blow which changes the course of the younger version of the ball but it would not stop it from entering the wormhole. This also explains that if you go back in time to kill your grandfather then something or the other will happen to prevent you from making it happen thus preserving the consistency of the History.
Solutions for the Paradoxes
In order to come up with a solution for these above-mentioned paradoxes, scientists have proposed some explanations which are enlisted below
The Solution: Time Travel is impossible because of the paradoxes that it creates.
Self-Healing Hypothesis: If we succeed to change the events in the past, it will set off another set of events that will keep the present unchanged.
The Multiverse: Every time an event in the past is altered, an alternate parallel universe or timeline is created.
Erased Timeline Hypothesis: A person traveling to the past would exist in the new timeline but their own timeline would be erased.
Is Time Travel Possible?
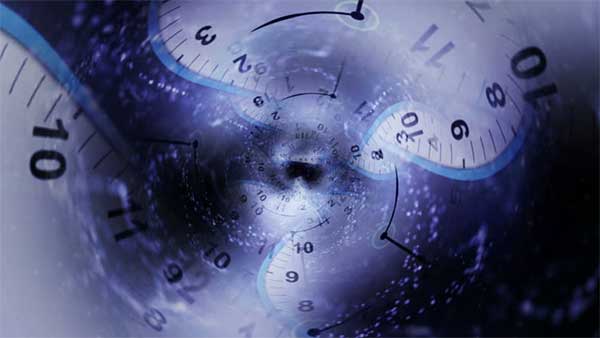
Nobody seems to have a definite answer in support or against the existence of Time Travel. On one hand, Einstein suggested to traveling at the speed of light in order to jaunt through the future but this would mean an unimaginable amount of energy would be required. Moreover, the centrifugal force on the body would prove to be fatal. Although it has been observed that space travelers age a little slower as compared to their identical twin on earth but some believe that there is no definite answer to travel back in space.
Theoretical physicist Brian Greene of Columbia University says that “No one has given a definite proof that you can’t travel to the past. But every time we look at the proposals and detail it seems kind of clear that they’re right at the edge of the known laws of physics.” Besides, Prof. Hawking feels that “Today’s science fiction is tomorrow’s science fact.”
However, the paradoxes, especially the grandfather paradox, have imposed a big question mark on the possibility of Time Travel. Basically, with the present laws and knowledge of Physics, the human won’t be able to survive in the process of Time Travel. So, we need certain developments in the quantum theories till we are sure as to how the paradoxes can be solved.
You may read our Blog and Article section for more topics on electronics engineering, industry, and technology.
Questions related to this article? 👉Ask and discuss on Electro-Tech-Online.com and EDAboard.com forums. Tell Us What You Think!! Cancel reply
You must be logged in to post a comment.
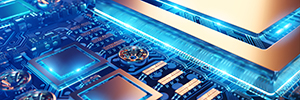
Search Engineers Garage
- Raspberry pi.
- BeagleBone.
- 8051 Microcontroller.
- PIC Microcontroller.
- Battery Management
- Electric Vehicles
- EMI/EMC/RFI
- Hardware Filters
- IoT tutorials
- Power Tutorials
- Circuit Design
- Project Videos
- Tech Articles
- Invention Stories
- Electronic Product News
- Business News
- Company/Start-up News
- DIY Reviews
- EDABoard.com
- Electro-Tech-Online
- EG Forum Archive
- Cables, Wires
- Connectors, Interconnect
- Electromechanical
- Embedded Computers
- Enclosures, Hardware, Office
- Integrated Circuits (ICs)
- LED/Optoelectronics
- Power, Circuit Protection
- Programmers
- RF, Wireless
- Semiconductors
- Sensors, Transducers
- Test Products
- eBooks/Tech Tips
- Design Guides
- Learning Center
- Webinars & Digital Events
- Digital Issues
- EE Training Days
- LEAP Awards
- Webinars / Digital Events
- White Papers
- Engineering Diversity & Inclusion
- Guest Post Guidelines
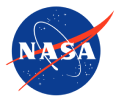
All Mars Resources
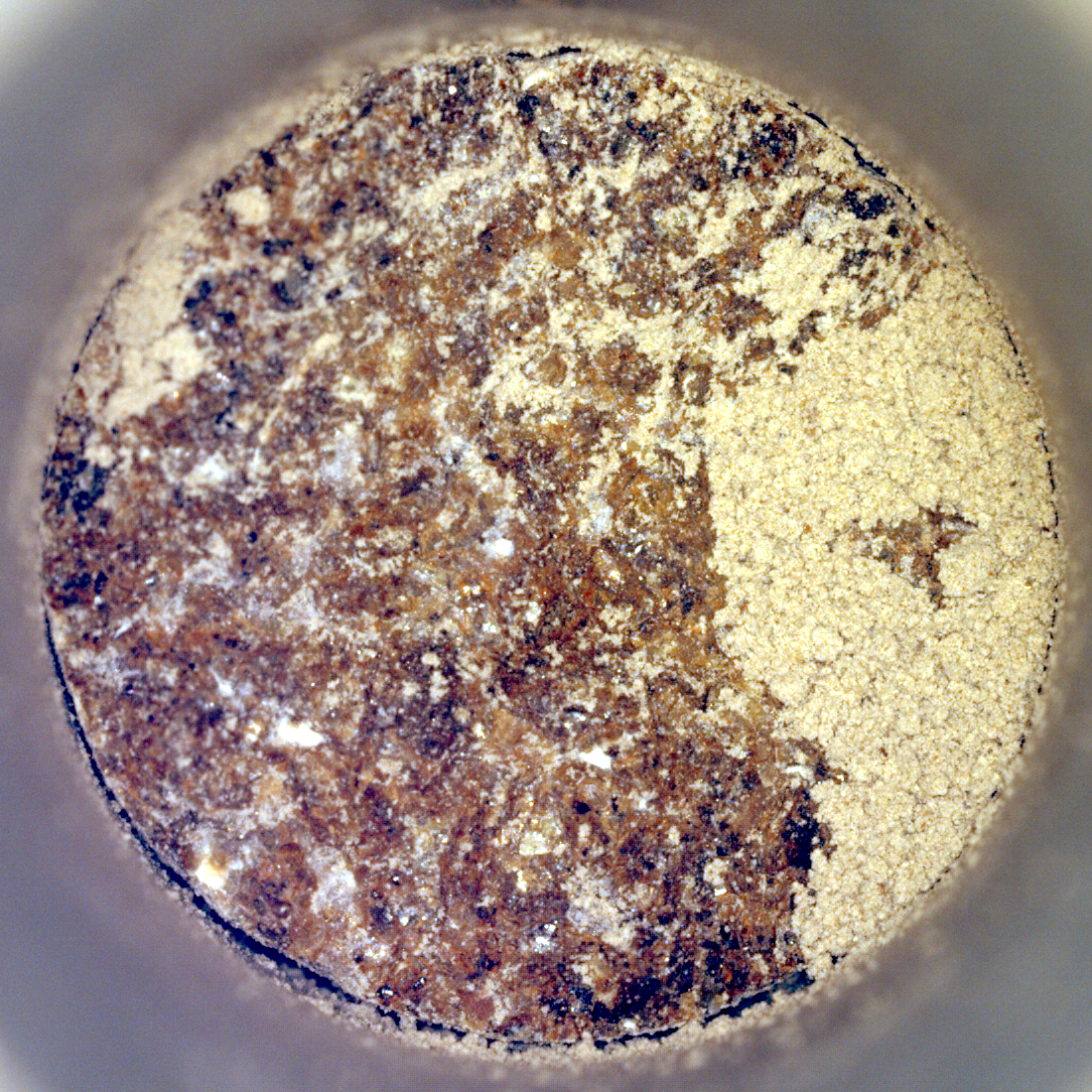
Perseverance’s ‘Bunsen Peak’ Sample
NASA’s Perseverance Mars rover captured this image of a sample cored from a rock called “Bunsen Peak” on March 11,…
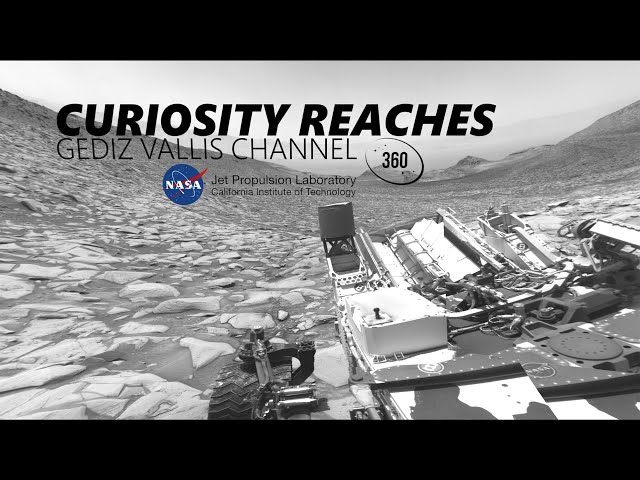
NASA’s Curiosity Rover Reaches Gediz Vallis Channel (360 View)
360-degree panorama provided by NASA’s Curiosity Mars rover. This view was captured at Gediz Vallis channel, a feature that formed…
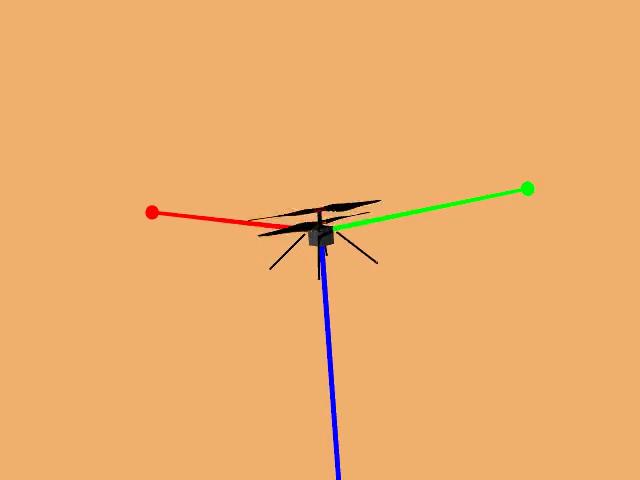
Animation of Mars Helicopter Flight Test
This animation shows a simulation of the response of NASA’s Ingenuity Mars Helicopter to the system identification, or “Sys-ID,” process.…
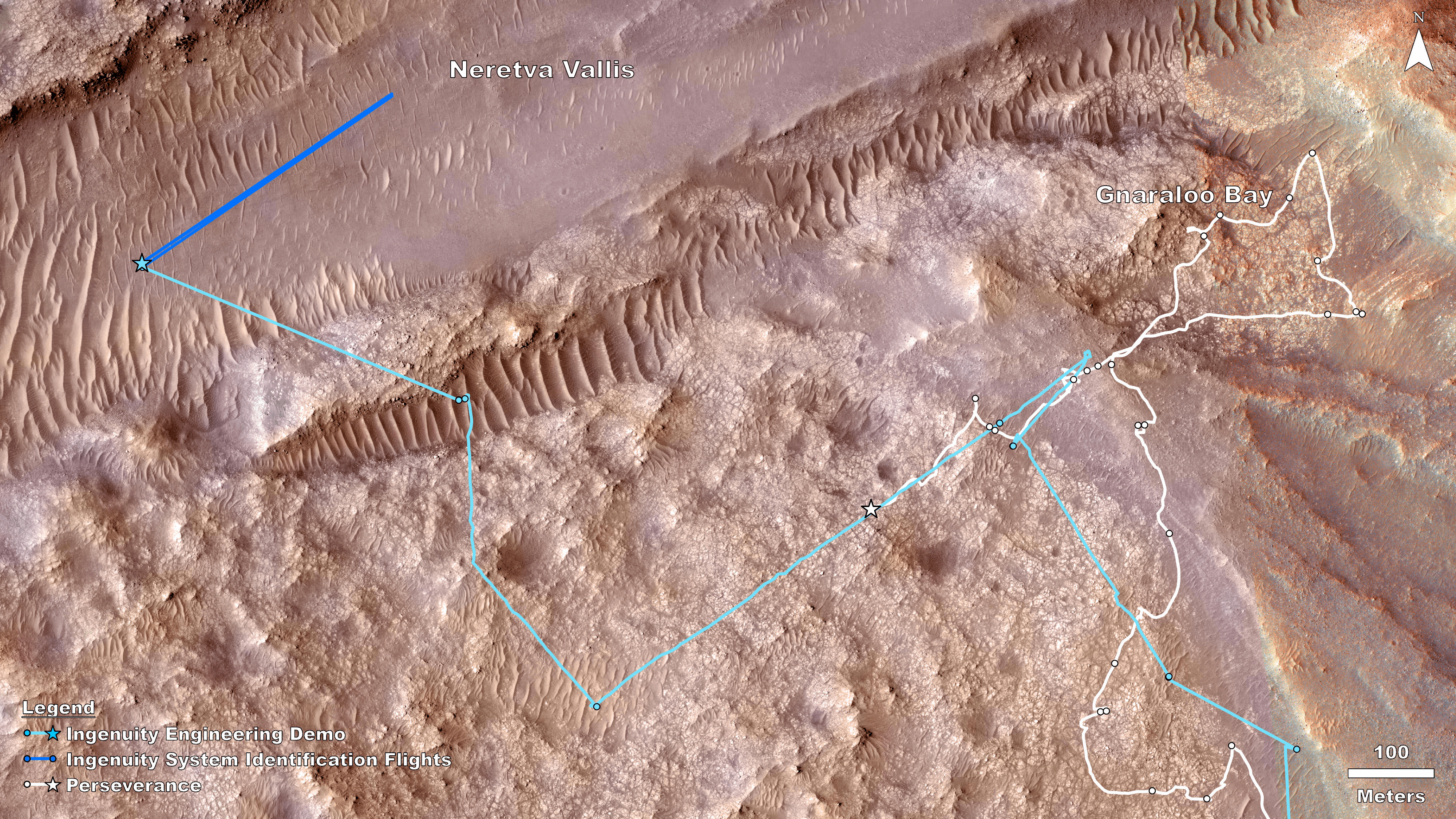
Rover, Helicopter Locations in Jezero Crater
This map shows the locations of NASA’ Perseverance rover (white star) and Ingenuity Mars Helicopter (cyan star) on Dec. 19,…
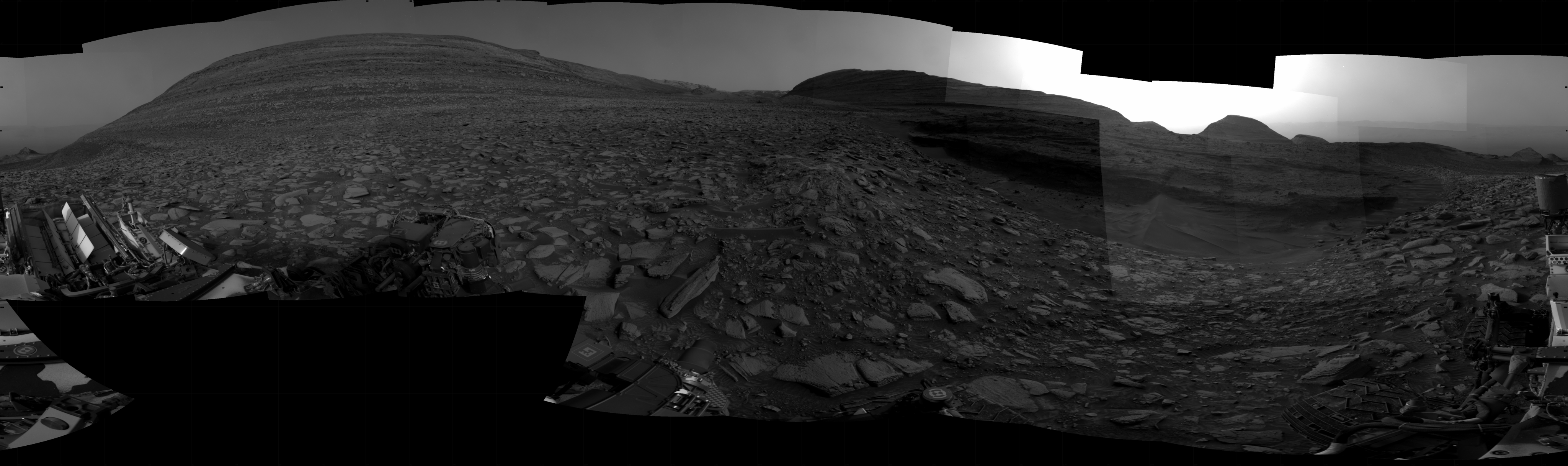
Sol 4132: Right Navigation Camera, Cylindrical Projection
NASA’s Mars rover Curiosity took 31 images in Gale Crater using its mast-mounted Right Navigation Camera (Navcam) to create this…
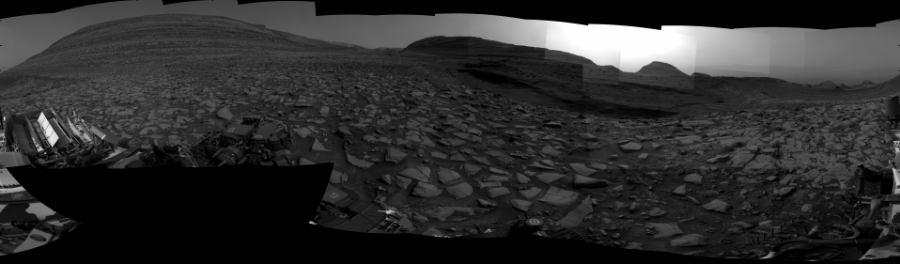
Sol 4130: Right Navigation Camera, Cylindrical Projection
NASA's Mars rover Curiosity took 31 images in Gale Crater using its mast-mounted Right Navigation Camera (Navcam) to create this…
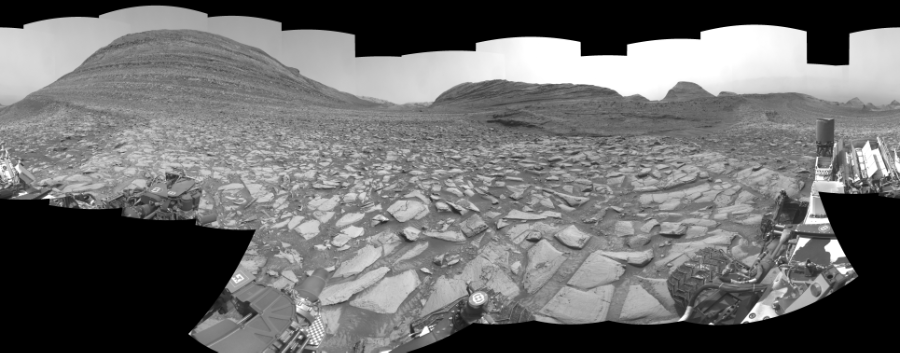
Sol 4128: Right Navigation Camera, Cylindrical Perspective
NASA's Mars rover Curiosity took 30 images in Gale Crater using its mast-mounted Right Navigation Camera (Navcam) to create this…
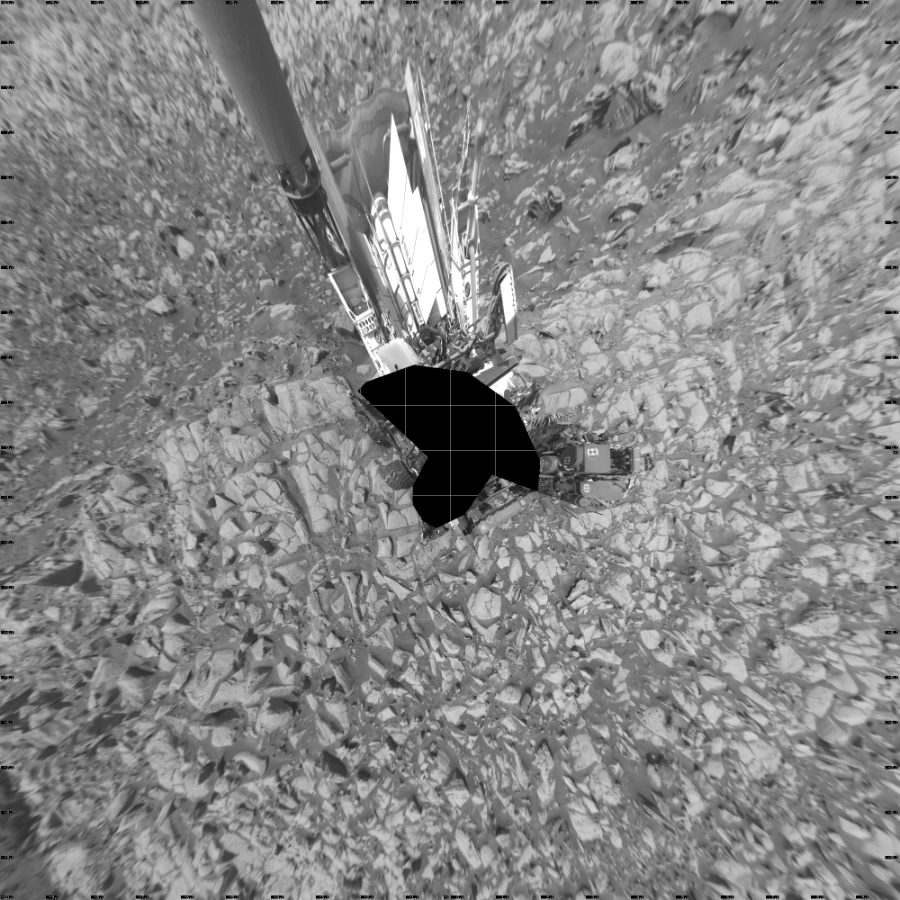
Sol 4128: Left Navigation Camera, Vertical Projection
NASA's Mars rover Curiosity took 30 images in Gale Crater using its mast-mounted Left Navigation Camera (Navcam) to create this…
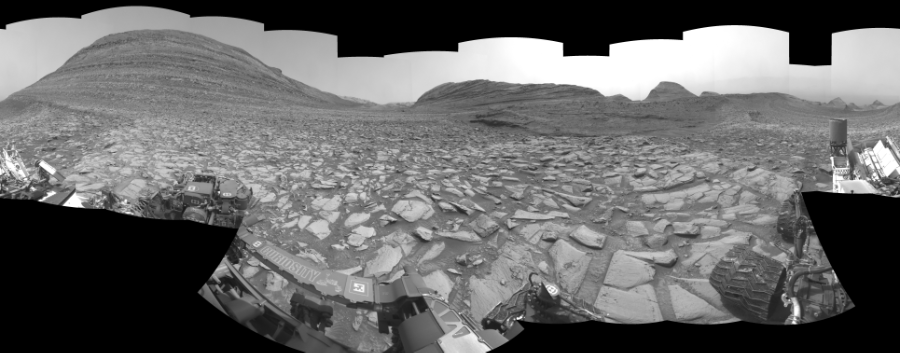
Sol 4128: Left Navigation Camera, Cylindrical Perspective
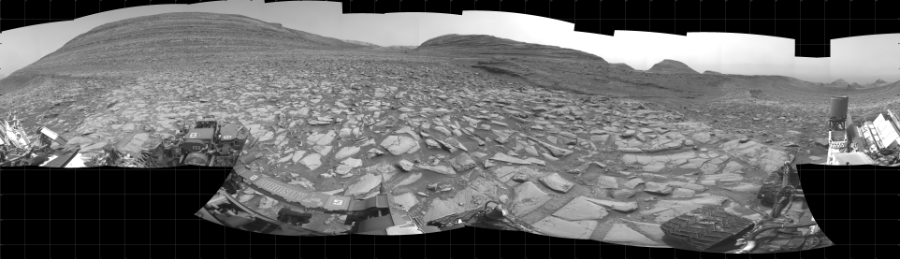
Sol 4128: Left Navigation Camera, Cylindrical Projection
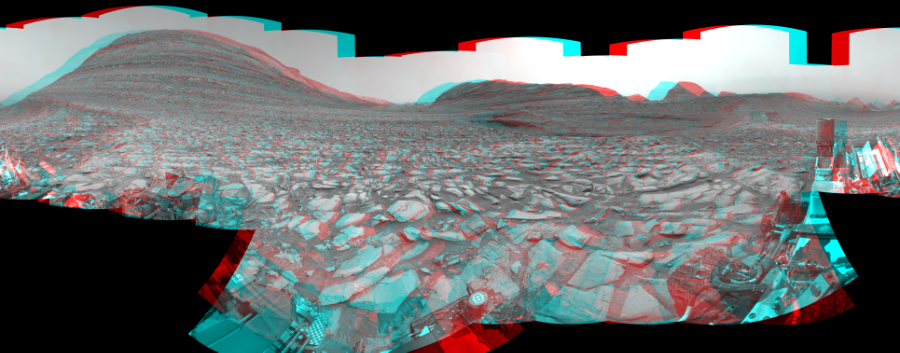
Sol 4128: Mast-Mounted Navigation Camera, Cylindrical Perspective
NASA's Mars rover Curiosity took 30 image pairs in Gale Crater using its mast-mounted Navigation Camera (Navcam) to create this…
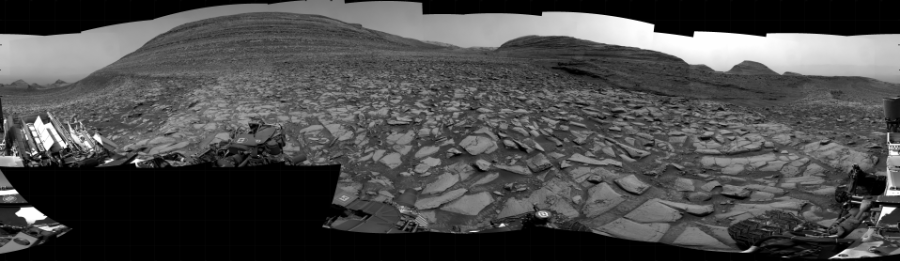
Sol 4128: Right Navigation Camera, Cylindrical Projection
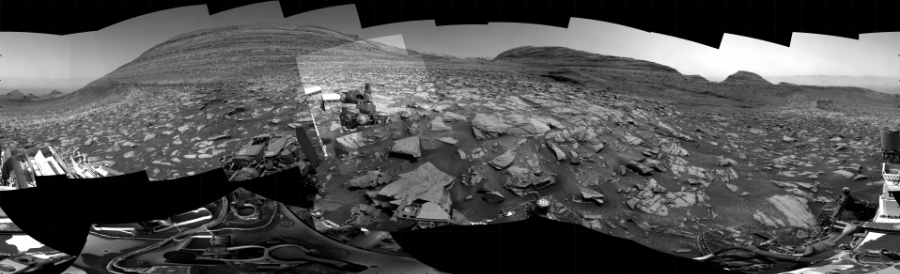
Sol 4125: Right Navigation Camera, Cylindrical Projection
NASA's Mars rover Curiosity took 52 images in Gale Crater using its mast-mounted Right Navigation Camera (Navcam) to create this…
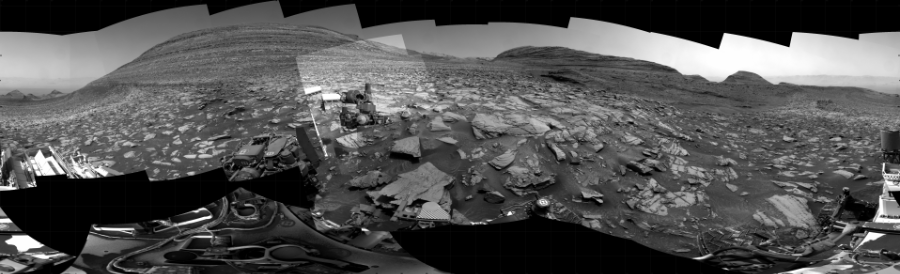
Sol 4123: Right Navigation Camera, Cylindrical Projection
NASA's Mars rover Curiosity took 51 images in Gale Crater using its mast-mounted Right Navigation Camera (Navcam) to create this…
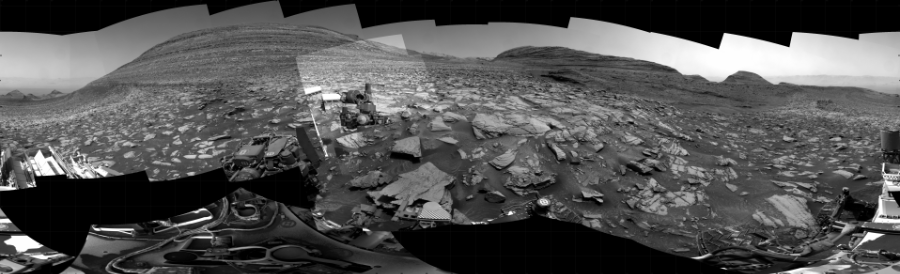
Sol 4118: Right Navigation Camera, Cylindrical Projection
NASA's Mars rover Curiosity took 49 images in Gale Crater using its mast-mounted Right Navigation Camera (Navcam) to create this…
The future of travel: 10 concepts that will change the way we experience the world
T ravel has come a long way since the age of Silk Road caravans, daunting sea voyages and steam locomotives -– and will continue to transform in years to come.
Concepts that feel plucked from sci-fi novels and films are quickly making their way into mainstream travel, shaping every step of the journey.
Sooner rather than later, we could be piloting passenger drones around Singapore or rocketing into Earth’s orbit to admire the world from the edge of space.
From autonomous taxis to passenger drones, biometric immigration tunnels, instant translation and hotels, here’s a peek at what’s coming down the runway.
The end of airport lines
Although controversial , biometric identification (automatically verifying one’s identity via fingerprints, facial recognition or iris scans) is quickly becoming the technology of choice at airports worldwide.
Considered a faster and more accurate way to screen passengers, biometrics can cut processing time for typical airport procedures – think baggage check-in, lounge access, boarding and immigration control – in half.
For example, in 2018, Dubai International Airport introduced biometric “Smart Gates” tunnels , which use facial recognition to verify travelers’ identities in as little as five seconds.
It’s as simple as it sounds: after deplaning, travelers walk into a tunnel, look at a green light, and then continue to baggage claim without waiting in line or interacting with an immigration officer.
Elsewhere in the world, facial recognition technology is already in use to some extent at Hong Kong International Airport , Tokyo Narita, Tokyo Haneda, Indira Gandhi International in Delhi, London Heathrow and Paris Charles de Gaulle, among other airports.
Meanwhile, the European Union plans to roll out an automated entry-exit system in 2024 that uses fingerprints and facial images to identify foreign travelers and streamline border control checks.
Airlines are also adopting biometric identification.
Emirates has created a “ biometric pathway ” at Dubai International Airport that enables passengers to pass through immigration and boarding without presenting their documents.
And in the US, major airlines like American Airlines, United and Delta have been experimenting with biometric check-in, bag drops and boarding gates at select airports for the last couple of years.
Less lost luggage
Have you arrived in a foreign country only to spend the first day of vacation stocking up on underwear, toiletries and essential clothing while your luggage takes an unexpected detour?
Given the millions of mishandled checked bags every year , it’s no wonder people are yearning for tech-savvy solutions to this common travel headache.
Some are turning to devices like SmartTags, Tile Pros and AirTags to keep track of their belongings. Others opt for sophisticated suitcases like the Samsara’s Tag Smart, which includes an integrated AirTag that syncs with Apple’s Find My app and uses Bluetooth to track the bag’s location.
Looking ahead, we’ll likely see digital bag tags containing RFID transmitters replace conventional paper tags – an evolution that would save time at check-in while simultaneously making tracking and identifying luggage easier for airlines.
Alaska Airlines, Lufthansa and Qatar Airways, among others, are ahead of the game, having partnered with Dutch digital bag tag pioneer BAGTAG. Such products enable travelers to register and activate their luggage tag at home, then drop luggage at a self-service kiosk and track it via an app.
Since tagging errors are just one reason for delayed or lost luggage , airlines and airports are also looking to solve common issues such as transfer mishandling, failure to load, ticketing errors and weather delays.
In the future, don’t be surprised if we see automated baggage handling, AI-powered bag recognition programs, AI security scanning and perhaps even an AI global database that links travelers with their bags – all solutions that could lead to fewer lost bags in the long run.
Next-level augmented reality
On a future trip to Europe, imagine exploring the Accademia Gallery in Florence guided by a talking “David” sculpture or embarking on a digital treasure hunt through the streets of Paris.
Requiring only a phone and internet connection, augmented reality (AR) can add another layer of intrigue to our travel experiences.
Specterras Productions , which strives to make the world’s natural and cultural wonders more accessible via technology, is already bringing such experiences to life.
“With AR, you can create animated busts at a museum – in the future, you’ll be able to walk up to a sculpture of Alexander the Great or Herodotus and interact with the art via your mobile phone, kind of like ‘Night at the Museum,” Michael Breer, chief creative officer at Specterras, tells CNN Travel.
AR and virtual reality (VR) became more mainstream during the Covid-19 pandemic when museums and destinations introduced interactive virtual experiences for would-be travelers.
What’s more, experimental artists like KAWS – known for his toy-like sculptures and collectables – famously embraced the technology in his “Expanded Holiday” project , which saw giant AR sculptures levitating in 12 cities around the world in 2020.
Breer says these technologies also enable people to explore parts of the world they might not be able to see in person.
“Economically, visiting places like Pompeii, Palmyra, Machu Picchu or the Great Barrier Reef is very difficult. So, for many people, VR and AR will provide a good substitute for these experiences,” Breer adds.
And in terms of planning, VR may also play a more vital role in decision-making in the future.
If travelers can explore a destination, hotel, restaurant or excursion via VR first, they will likely have more confidence when making reservations.
The rise of flying taxis
You’ve probably seen videos of passenger drones and eVTOL – electric vertical take-off and landing aircraft that don’t require a runway – taking over the internet.
These futuristic aircraft tend to be electric, ultralight and kitted out with autopilot software that makes it possible for everyday people to hop in the pilot seat – after an orientation session and VR flight simulation, of course.
That’s the strategy at LIFT Aircraft, which says that anyone can fly its amphibious HEXA aircraft in the US without a pilot’s license since it qualifies as an “ultra-light” vehicle under federal regulations. (That said, the Federal Aviation Administration recently proposed a comprehensive rule for training and certifying pilots of power-lifted aircraft.)
The aircraft offers many automated safety precautions like a collision-avoidance system, a triple-redundant flight computer and a ballistic parachute for the whole aircraft.
On track to start offering commercial flights in 2023, the company plans to let travelers fly the aircraft on short, scenic rides – about 8-15 minutes at a time – during a 25-city roadshow across the US.
In the future, customers will be able to find and book LIFT flights via a mobile app that provides flight simulation training, a proficiency test, a pre-flight checklist and ground crew support.
Other companies, like Ehang in China, are on a mission to alleviate traffic jams with flying taxi drones.
At the same time, New York-based Kelekona plans to offer flights on its battery-powered, 40-passenger drone bus as an eco-friendly alternative to mass transit.
Another notable company is Volocopter, which is on its way to bringing a fleet of electric air taxis to Singapore and Paris in 2024.
Then there’s the Jetson ONE – a single-person, all-electric eVTOL with auto-hover, stable flight and landing capabilities – which can fly for about 20 minutes up to 63 mph.
“A first application for a future flying car is to replace taxis in large cities,” Jetson co-founder Peter Ternstrom tells CNN Travel. “This will free up ground-level space and provide a much faster service for the passengers. The same goes for shuttle services from city centers to airports.
“Electric personal flying cars will revolutionize adventurous travel. Safaris, mountain tops, visiting Machu Picchu – all places that are currently difficult to get to will suddenly be possible.”
Robotaxis gain momentum
While some taxi services head into the clouds, others will stay firmly on terra firma – but with some serious upgrades.
Boston-based Motional, a joint venture between Hyundai and global tech company Aptiv, has seen some early success.
Available to public passengers in Las Vegas, Motional provides autonomous robotaxis (via Lyft and Uber apps) that can drop passengers at popular destinations on the Las Vegas Strip.
A person is still present in the robotaxi at the moment, but the cars are expected to become fully driverless later this year, the company tells CNN Travel.
Another company seeing momentum is Waymo (a subsidiary of Google’s parent company Alphabet Inc.), which has operated the first fully autonomous ride-hailing service in parts of Phoenix since 2020.
Available via the Waymo One app, the service has since expanded to downtown Phoenix, San Francisco and, next up, Los Angeles.
Of course, major players like Amazon, Tesla and Cruise are speeding forward in North America.
Amazon’s self-driving vehicle unit known as Zoox is being tested in San Francisco; an update to Tesla’s Full Self-Driving Beta software became available in mid-March, addressing the safety risks associated with an earlier recall ; and Cruise has operations underway in San Francisco and Austin.
On the other side of the world, in China, tech giant Baidu began operating a fully autonomous ride-hailing service, Apollo Go, in cities like Beijing, Chongqing and Wuhan in 2022 and plans to expand rapidly in 2023.
“Autonomous ride-hailing service is gradually becoming part of people’s everyday lives,” a Baidu spokesperson tells CNN Travel. “In cities like Beijing, Shanghai and Guangzhou, each robotaxi on Apollo Go can complete more than 15 rides per day on average.”
Net-zero flights on the horizon
As passenger drones and eVTOLs become commonplace, you may wonder: what’s next for good old-fashioned airplanes?
The future of travel is inextricably linked to climate change – a reality that led the International Civil Aviation Organization (ICAO) to announce a long-term goal to achieve net-zero carbon emissions by 2050 .
Thanks to a mix of emerging technologies ranging from sustainable aviation fuel to hydrogen-powered engines to all-electric planes, it’s certainly possible, though critics have expressed considerable doubt .
US-based Eviation Aircraft is leading the way, providing a peek into the future with its sleek, all-electric “Alice” plane.
Set to enter service in 2027, the nine-seater commuter plane aims to reduce the environmental impact of regional hops. So far, over 300 Alice aircraft have been ordered by the likes of Aerus airline in Mexico, DHL, Air New Zealand and more.
We may also see a supersonic comeback within the next decade. Denver-based Boom has been working on an updated version of the Concorde that aims to make supersonic travel much quieter, greener and more affordable.
According to the company, Boom’s “Overture” aircraft can carry up to 80 people at Mach 1.7 speeds (or about twice as fast as traditional airlines) while emitting zero carbon emissions.
Meaning, a flight from New York City to Frankfurt could take four hours instead of eight, or you could go from LA to Sydney in eight hours instead of 14.
And since Overture will fly at a higher altitude than today’s aircraft – up to 60,000 feet – travelers would get to see the curvature of the Earth through the windows.
So far, several major airlines, including American Airlines, United and Japan Airlines, have placed orders for Overture, signaling industry confidence in the future of supersonic.
We’ll have to be patient, though – Boom plans to commence aircraft production in 2024, conduct test flights in 2027, and achieve certifications to carry passengers as early as 2029.
Eco-fabulous hotel stays
Like air travel, eco-conscious hotels are paving the way for more sustainable travel in the future.
When room2 Chiswick opened in London in 2021, it became the world’s “whole life net-zero” hotel.
In other words, the property has offset its entire carbon footprint, from production and construction, materials, maintenance, electricity and so on.
Compared with a typical UK hotel of a similar size, room2 uses 89% less energy per square meter thanks to ground source heat pumps, solar panels, water-saving fixtures, sustainable procurement policies, a biodiverse green roof and a zero-waste policy.
In the US, Bauhaus-style Hotel Marcel New Haven, Tapestry Collection by Hilton , is on track to become the first net-zero hotel in the country after revitalizing a heritage building designed by Hungarian-born architect Marcel Breuer.
Opened in May 2022, the hotel’s day-to-day operations are fully solar-powered by 1,000 PV panels on the roof, and, as a result, the property emits zero carbon emissions.
It also features triple-glazed windows, an electric shuttle van, and a carport full of electric car chargers, including 12 Tesla Superchargers – enhancements that have earned the property LEED Platinum certification.
Looking ahead, the Six Senses Svart aims to be the world’s first energy-positive hotel when it opens in 2024 within the Arctic Circle in Norway.
With its low-impact overwater design inspired by local fish-drying structures, a solar power system, a zero-waste restaurant and efficient waste and water management systems, the hotel aims to help eco-minded travelers responsibly explore the polar region.
Also coming up in 2024 is Sheybarah Resort , an entirely off-grid resort in a protected marine park in the Red Sea off the coast of Saudi Arabia known for its dense mangroves, pristine coral reefs and sea life.
The LEED-Platinum property plans to minimize its footprint in many ways, from suspending pod-like hotel rooms above the water (so as not to disturb marine life) to installing a solar farm, a solar-powered desalination plant for fresh water and on-site recycling facilities.
Say goodbye to language barriers
There’s nothing wrong with playing charades, but wouldn’t it be amazing to have deeper conversations with the people you meet while traveling?
Suppose language barriers became a thing of the past. In that case, we could connect across cultures, work and live abroad, discover new business opportunities and relate to far-flung family members.
The good news is that real-time translation is growing more sophisticated by the day.
Google’s Pixel Buds can either translate what’s being said directly into your ear or share a transcription so you can follow along.
The company has also been working on wearable augmented-reality glasses that display translated text on the lenses in real-time.
Another company, Mymanu , also offers auto voice translation via its CLIK S earbuds.
Currently used in the hospitality industry and soon to be rolled out to help asylum seekers in the UK , the headphones sync with the company’s MyJuno translation app to help users communicate with people in over 37 languages.
The company says it will soon be launching the world’s first voice-controlled, eSIM-enabled earbuds. Called “Titan,” they can be used like a screen-less phone, and they also offer live translation without the need for a phone app.
The space race rockets forward
We’re well on our way to exploring the final frontier with a flurry of space tourism initiatives available now – or very soon – to the most affluent explorers.
According to SpaceVIP , which bills itself as the only aggregator of space-related experiences, roughly a dozen types of expeditions are available in 2023, with many more to follow.
For starters, Blue Origin offers excursions past the Kármán Line – a boundary 62 miles above sea level that marks the beginning of outer space.
Meanwhile, S paceX has successfully launched commercial orbits around the Earth, missions to the International Space Station (ISS), and plans to take Japanese entrepreneur Yusaku Maezawa and an international crew of artists, actors, musicians and athletes on the first civilian mission around the moon in 2023.
Those seeking a leisurely and luxurious experience can soon travel to the edge of space in a pressurized Spaceship Neptune capsule, propelled by a SpaceBalloon (the same used by NASA), with Space Perspective .
During the six-hour journey, due to launch in 2024, travelers will enjoy panoramic views of the Earth, a gourmet meal and cocktails before a slow descent and water landing.
Virgin Galactic, meanwhile, plans to launch 90-minute joyrides into the upper atmosphere this summer .
If they do go ahead, the $450,000 suborbital flight will reach about 50 miles above the planet, where passengers will have about a minute to enjoy astounding views and experience zero gravity.
Meanwhile, several companies, including Hi-Seas, Space Training Academy, Nastar Center and Air Zero G, provide Earth-bound training programs so you can experience various levels of gravity, learn astronaut maneuvers and enjoy simulator experiences like a moon launch or ISS docking.
Hyperloop coming down the pipeline
US entrepreneur Elon Musk has been talking about hyperloop technology – an ultra-high-speed transport system in a low-pressure vacuum tube – for years.
As the pitch goes, with hyperloop networks, we could travel in pods up to 760 mph from LA to San Francisco in 30 minutes, Beijing to Shanghai in 60, and Paris to Amsterdam in 90.
In addition to saving time, proponents claim hyperloop also has the potential to provide a cheaper, faster, safer and more sustainable alternative to conventional mass transit.
Over the past decade, early movers like Musk’s The Boring Company, Virgin Hyperloop (now Hyperloop One) and Hyperloop Transportation Technologies (HyperloopTT) made some impressive headway but encountered regulatory, funding and infrastructure challenges.
Despite an unsuccessful IPO attempt earlier this year , HyperloopTT is reportedly working on potential projects, including a “Great Lakes” link between Chicago, Cleveland and Pittsburgh, according to Bloomberg .
While thoroughly tested hyperloop transit networks may still be a decade, if not decades, away, many innovative companies are pushing the technology forward.
According to the South China Morning Post , the China Aerospace Science and Industry Corporation completed a few successful hyperloop test runs in Shanxi province in January 2023.
In addition, Hardt Hyperloop in the Netherlands demonstrated its technology at low speeds and is now constructing the European Hyperloop Center for high-speed demonstrations and testing.
Meanwhile, Toronto-based TransPod hopes to bring hyperloop technology to Canada with its eponymous tube-based transportation system powered by renewable energy.
By 2025, the company plans to build a 620-mile-per-hour TransPod link between Calgary and Edmonton, connecting the two cities in 45 minutes.
Maybe it’s a pipedream – or maybe it will transform travel as we know it.
For more CNN news and newsletters create an account at CNN.com
Screen Rant
The clone wars set up a major arc for an iconic revenge of the sith villain - but never used it.
The Clone Wars set up a story arc to explore the backstory of one of its most iconic villains, but the show never capitalized on the chance.
- General Grievous lacked character development in The Clone Wars, remaining one-dimensional and unexplored.
- The show hinted at Grievous' rich backstory with statues in his lair, but failed to capitalize on its potential.
- The Clone Wars missed the opportunity to explore why Grievous hated the Jedi, which could have made him a much better villain.
Star Wars: The Clone Wars provided a wealth of information about some of the best characters in the prequel trilogy, but there's one Revenge of the Sith villain whose backstory was barely explained, despite having a major arc set up for it. The Clone Wars showcased some of Star Wars ' most powerful villains , and it also gave interesting context to many of their stories. Some villains were even completely changed in the show, which made their stories even better, as shown by Darth Maul's essential viewing list . Not every villain got as much chance to stand out, though, and one in particular was almost completely forgotten.
Star Wars has a long history of introducing extremely cool villains and killing them off too quickly. The most notable example is Boba Fett, one of the best bounty hunters in Star Wars . Boba Fett's backstory was explored in the prequel trilogy, and he eventually got his own show that allowed him to become a more developed character. Other villains, like one from Revenge of the Sith , never got such a chance, even though The Clone Wars set up an entire story arc. That story arc could have made them a much more compelling villain, and fixed one of the show's rare mistakes.
Star Wars: The Clone Wars Voice Cast - What The Actors Look Like In Real Life
General grievous never got much character development in the clone wars, grievous never grew out of his cowardly depiction.
Of all the villains featured in The Clone Wars , General Grievous had by far the least development. Throughout the entire show, Grievous was only ever shown as a competent but cowardly warrior who would flee at the first sign of a fight going wrong . While there were moments when his true power shone through, such as his assault on the Nightsisters of Dathomir , very few layers of his character were ever revealed in The Clone Wars . There was one episode that explored Grievous' past, though it didn't go into much detail, and it never became as big as it could have been.
The Statues In Grievous’ Lair Almost Made Him An Even More Compelling Villain
The clone wars hinted at his rich & interesting backstory, but never used it.
In The Clone Wars season 1, episode 10, Jedi Master Kit Fisto and his former Padawan infiltrated General Grievous' lair. As they examined his base ahead of his arrival, they came upon several statues that showed Grievous at various stages of his life and with varying levels of cybernetic implants . Grievous also told his medical droid that he "submits to no one" and that he considered his implants to be improvements. Those moments offered a rare glimpse into Grievous' backstory and some of his more interesting character traits, which would have been a compelling story arc for the show to follow.
Exploring Grievous' history could have made him so much more compelling than the cowardly general he was depicted as, but the opportunity was never capitalized on.
Before Grievous was a cyborg general, he was a Kaleesh warlord. The Kaleesh were a reptilian species whose home planet was located in the Wild Space region of the Star Wars galaxy map . Grievous led a war band across Wild Space to invade and conquer several species, like the Huk, which explains why he was so fearsome during the Clone Wars. The statues in Grievous' lair hinted at his warlord backstory, but it was completely abandoned after that episode . Exploring Grievous' history could have made him so much more compelling than the cowardly general he was depicted as, but the opportunity was never capitalized on.
How Could The Clone Wars Have Used Grievous’ Backstory More?
The show could have explored why grievous hated the jedi so much.
While The Clone Wars couldn't have directly shown Grievous' backstory, as it took place years prior, there were several ways it could have been referenced. In Star Wars canon, the Huk called for help after Grievous' rampage, and the Republic and Jedi allied with them, causing Grievous' hatred of both. A Clone Wars episode could have traveled to the Huk world to see the effects Grievous had on them and explore the effects the Republic's intervention had on the Kaleesh in greater detail . The show also could have seen the Separatists capitalize on the Kaleesh's hatred of the Republic and form an alliance with them.
The Clone Wars also could have addressed his history more indirectly by focusing on some of the personality traits Grievous displayed in that episode. His conversation with the medical droid established the pride Grievous felt in himself and his implants, but it also showed how much he wanted Count Dooku's approval. That could have inspired resentment in Grievous, and it would have been the perfect setup for an episode showing him challenging Dooku . It also would have utilized his statement that he never submitted to anyone, which would have then given Grievous a much more compelling reason to be so angry beyond simple hatred of the Jedi.
Grievous' next on-screen appearance will be in Star Wars: Tales of the Empire , which releases on Disney+ on May 4.
Grievous' character development, or lack thereof, is one of the few mistakes The Clone Wars ever made. As the commander of the droid army, Grievous was one of the show's main villains, but he was also one-dimensional. Exploring his backstory would have made Grievous a much more complex villain, and it would have made The Clone Wars better as a whole. There's still a chance that some future Star Wars story will give Grievous that development, but for now, he remains on the list of the franchise's under-utilized villains.
Star Wars: The Clone Wars
*Availability in US
Not available
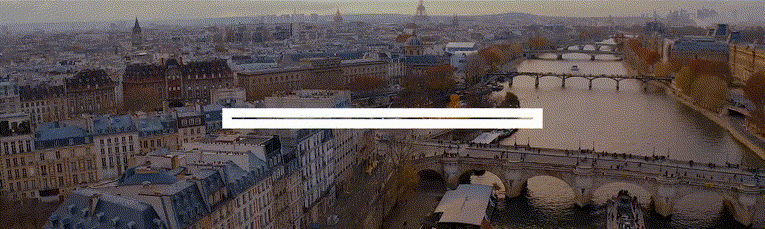
IMAGES
VIDEO
COMMENTS
The first page of The Time Machine published by Heinemann. Time travel is the hypothetical activity of traveling into the past or future.Time travel is a widely recognized concept in philosophy and fiction, particularly science fiction. In fiction, time travel is typically achieved through the use of a hypothetical device known as a time machine.The idea of a time machine was popularized by H ...
The concept of time travel — moving through time the way we move through three-dimensional space — may in fact be hardwired into our perception of time. Linguists have recognized that we are ...
In Summary: Yes, time travel is indeed a real thing. But it's not quite what you've probably seen in the movies. Under certain conditions, it is possible to experience time passing at a different rate than 1 second per second. And there are important reasons why we need to understand this real-world form of time travel.
Time travel is the concept of moving between different points in time, just like you move between different places. In movies, you might have seen characters using special machines, magical ...
The result is a so-called wormhole, a concept that's familiar from sci-fi movies, but actually originates in Einstein's theory of relativity. ... This doesn't prove time travel is impossible, but ...
Time travel is the concept of moving between different points in time, just like you move between different places. In movies, you might have seen characters using special machines, magical ...
Time Travel. Time travel is commonly defined with David Lewis' definition: An object time travels if and only if the difference between its departure and arrival times as measured in the surrounding world does not equal the duration of the journey undergone by the object. ... Also, different metaphysical concepts of time result in different ...
Time Travel. First published Thu Nov 14, 2013; substantive revision Fri Mar 22, 2024. There is an extensive literature on time travel in both philosophy and physics. Part of the great interest of the topic stems from the fact that reasons have been given both for thinking that time travel is physically possible—and for thinking that it is ...
An observer traveling at high velocity will experience time at a slower rate than an observer who isn't speeding through space. While we don't accelerate humans to near-light-speed, we do send ...
Time travel, a longstanding fascination in science fiction, remains a complex and unresolved concept in science. The second law of thermodynamics suggests time can only move forward, while Einstein's theory of relativity shows time's relativity to speed. Theoretical ideas like wormholes offer potential methods, but practical challenges and ...
For centuries, the concept of time travel has captivated people's imaginations. Time travel is the concept of moving between different points in time, just like you move between different places. In movies, you might have seen characters using special machines, magical devices or even hopping into a futuristic car to travel backward or ...
Time travel and parallel timelines almost always go hand-in-hand in science fiction, but now we have proof that they must go hand-in-hand in real science as well. General relativity and quantum ...
10. Time Travel. We are all familiar with time travel stories, and there are few among us who have not imagined traveling back in time to experience some particular period or meet some notable person from the past. But is time travel even possible? One question that is relevant here is whether time travel is permitted by the prevailing laws of ...
Time and Relativity . Though referenced in H.G. Wells' The Time Machine (1895), the actual science of time travel didn't come into being until well into the twentieth century, as a side-effect of Albert Einstein's theory of general relativity (developed in 1915). Relativity describes the physical fabric of the universe in terms of a 4-dimensional spacetime, which includes three spatial ...
Of all time travel's paradoxes, here's the strangest of them all: hop on a TARDIS back to 1894 and the concept didn't even exist. "Time travel is a new idea," explains New York-based author James ...
Time travel has been a staple of science fiction. With the advent of general relativity it has been entertained by serious physicists. But, especially in the philosophy literature, there have been arguments that time travel is inherently paradoxical. The most famous paradox is the grandfather paradox: you travel back in time and kill your ...
Perhaps because of this connection between space and time, the possibility that time can be experienced in different ways and traveled through has surprisingly early roots. One of the first known examples of time travel appears in the Mahabharata, an ancient Sanskrit epic poem compiled around 400 B.C., Lisa Yaszek, a professor of science ...
Some think the concept of time travel is promising. Before we examine time travel theories, we should first visit Albert Einstein's explanation of time. Einstein, Space and Time. The majority of people view time as a constant, linear construct. One that moves forward at a regular pace. After all, we organise our lives around a 24-hour clock ...
Time travel is actually somewhat unique in science fiction. Many core concepts have their origins earlier in history. ... The historical roots of the concept of a 'robot' can be seen in Jewish ...
Time Travel has been a matter of great interest for Science fiction since ages. Whether it's the movies like Planet of the Apes (1968) or modern franchises like "Doctor Who" and "Star Trek"; the concept is grabbing a lot of eyeballs.Not only movies and shows but even some mythological tales like Mahabharata and the Japanese story of Urashima Taro support the evidence that time travel ...
Mallett posits that by twisting time into a loop, one could travel from the future back to the past - and then back to the future. And this is the idea of a wormhole, a sort of tunnel with two ...
Time travel has long been a captivating concept that has intrigued the human imagination. The idea of traversing through time, visiting the past or future, and altering events is a topic that has…
NASA's Perseverance Mars rover captured this image of a sample cored from a rock called "Bunsen Peak" on March 11,… NASA's Curiosity Rover Reaches Gediz Vallis Channel (360 View) 360-degree panorama provided by NASA's Curiosity Mars rover. This view was captured at Gediz Vallis channel ...
On track to start offering commercial flights in 2023, the company plans to let travelers fly the aircraft on short, scenic rides - about 8-15 minutes at a time - during a 25-city roadshow ...
Before Grievous was a cyborg general, he was a Kaleesh warlord. The Kaleesh were a reptilian species whose home planet was located in the Wild Space region of the Star Wars galaxy map.Grievous led a war band across Wild Space to invade and conquer several species, like the Huk, which explains why he was so fearsome during the Clone Wars.
A torrent of rain on Tuesday flooded parts of Dubai, turned streets into rivers and shut down the world's second-busiest airport for a time — a deluge of water that triggered the question: Was ...