AACR Annual Meeting News: Read the latest session previews and recaps from the official news website.
Select "Patients / Caregivers / Public" or "Researchers / Professionals" to filter your results. To further refine your search, toggle appropriate sections on or off.
Home > About the AACR > Newsroom > Meeting News > A New Era in Targeting KRAS
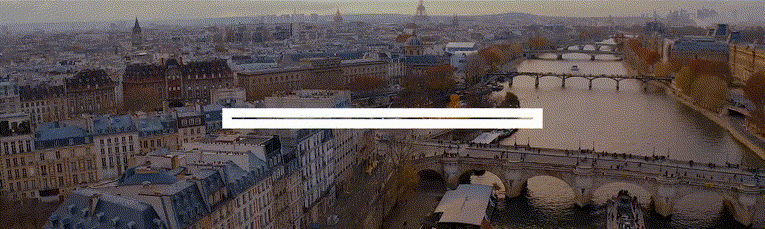
A New Era in Targeting KRAS
Researchers discuss recent progress in targeting a so-called “undruggable” protein at American Association for Cancer Research meetings.
In 1982, dubbed “The Year of the Oncogene” by Nature , Mariano Barbacid, PhD, FAACR , and others identified and isolated a family of cancer-causing genes from human cells for the first time—first HRAS, then KRAS.
Fast forward to March 2023, when Dr. Barbacid opened the AACR Special Conference: Targeting RAS by commemorating the 10 th anniversary of a seminal 2013 paper by Kevan Shokat, PhD, FAACR , that refuted KRAS’s status as an “undruggable” protein. Dr. Shokat discussed this research in an interview with AACR Stories.
As a result of Dr. Shokat’s research, Dr. Barbacid explained, two direct inhibitors of the KRAS G12C mutant protein are now approved to treat patients with lung cancer, illustrating the immense progress made in the 40-plus years since The Year of the Oncogene.
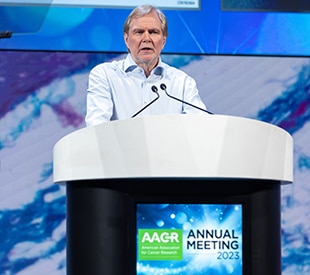
Dr. Barbacid then turned his attention to the future, asking, “What’s next?”
He predicted that the future of treating KRAS-mutated cancers would involve targeting additional KRAS mutants, either directly through the development of new inhibitors against these other mutations, such as those common in pancreatic cancers, or indirectly by blocking the downstream cancer-promoting effects that are activated by KRAS mutants.
“This is not the end of the story,” he contended. “I think that a new era in KRAS, in targeting KRAS, is in front of us.”
Fellow KRAS researchers Frank McCormick, PhD, FAACR, and an AACR Past President ; and Dafna Bar-Sagi, PhD, FAACR , averred that a chief priority for the field is understanding and overcoming treatment resistance, which is common among patients treated with KRAS inhibitors. They suggested that targeting signaling downstream of KRAS or harnessing the immune system might be effective approaches to prevent or circumvent resistance.
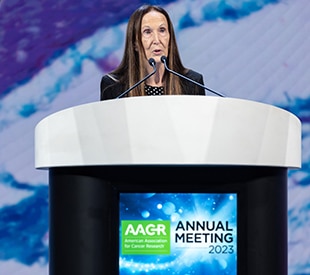
“RAS has a very profound effect on the immune microenvironment,” said Dr. Bar-Sagi, adding that future efforts to target KRAS and overcome resistance should account for these effects.
As researchers continue to address key questions, such as how to design inhibitors against different KRAS mutants and how to improve treatment responses, collaboration will be key, noted AACR Past President David A. Tuveson, MD, PhD, FAACR .
“I’m really pleased to see … biopharma collaborating with academia to ask these questions because it will be essential to getting to the next level of combinations,” he said.
At the Targeting RAS conference, Rising Star Andrew Aguirre, MD, PhD , agreed. “It is incumbent on all of us to work together … to work with pharmaceutical companies to develop appropriate trials, to share data early and often.”
AACR’s array of scientific conferences provides important avenues for researchers to form collaborations and share progress in their research. The first plenary session of the AACR Annual Meeting 2023, “ Beating KRAS: A 30-year Overnight Sensation,” highlights progress in targeting cancers driven by mutations in KRAS.
Visit the official AACR blog, Cancer Research Catalyst , for an overview of research presented at recent AACR conferences:
- Beating KRAS highlights the first plenary session of the AACR Annual Meeting 2023 .
- Driving Progress Against RAS-driven Cancers summarizes research presented by Drs. Barbacid, Aguirre, and Tuveson during the AACR Special Conference: Targeting RAS.
- KRAS, A History outlines key advances from the past four decades of KRAS research, which were discussed at the AACR Annual Meeting 2022.
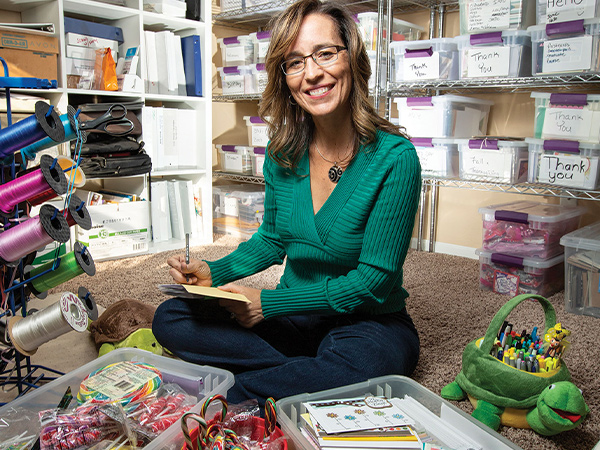
Brain cancer survivor Lanette Veres started the Gray Matters Foundation, a nonprofit that provides encouragement to brain tumor patients through gift packages and cards. Read Lanette's story and the stories of other cancer survivors.
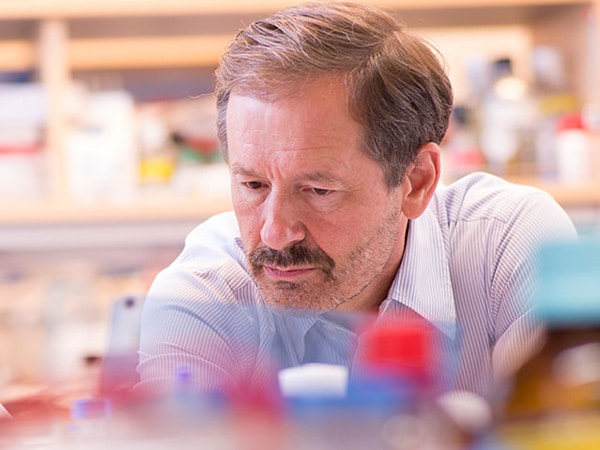
Learn about physician-scientists and cancer researchers like Fellow of the AACR Academy Lewis C. Cantley, PhD, who are driving progress against cancer.
- Annual Meeting 2022: KRAS, A History
- Kevan M. Shokat, PhD: Drugging the Elusive KRAS
- Targeting “Undruggable” Proteins That Drive Cancer
The Therapeutic Landscape for KRAS -Mutated Colorectal Cancers
Affiliations.
- 1 Conjoint Gastroenterology Laboratory, QIMR Berghofer Medical Research Institute, Herston, QLD 4006, Australia.
- 2 School of Medicine, The University of Queensland, Herston, QLD 4029, Australia.
- 3 Department of Medical Oncology, Cancer Care Services, The Royal Brisbane and Women's Hospital, Herston, QLD 4029, Australia.
- 4 Department of Medical Oncology, The Prince Charles Hospital, Chermside, QLD 4032, Australia.
- 5 Conjoint Internal Medicine Laboratory, Pathology Queensland, Queensland Health, Brisbane, QLD 4006, Australia.
- PMID: 37190303
- PMCID: PMC10136970
- DOI: 10.3390/cancers15082375
Colorectal cancer is one of the world's most prevalent and lethal cancers. Mutations of the KRAS gene occur in ~40% of metastatic colorectal cancers. While this cohort has historically been difficult to manage, the last few years have shown exponential growth in the development of selective inhibitors targeting KRAS mutations. Their foremost mechanism of action utilizes the Switch II binding pocket and Cys12 residue of GDP-bound KRAS proteins in G12C mutants, confining them to their inactive state. Sotorasib and Adagrasib, both FDA-approved for the treatment of non-small cell lung cancer (NSCLC), have been pivotal in paving the way for KRAS G12C inhibitors in the clinical setting. Other KRAS inhibitors in development include a multi-targeting KRAS-mutant drug and a G12D mutant drug. Treatment resistance remains an issue with combination treatment regimens including indirect pathway inhibition and immunotherapy providing possible ways to combat this. While KRAS-mutant selective therapy has come a long way, more work is required to make this an effective and viable option for patients with colorectal cancer.
Keywords: GTPase-activating protein (GAP); Kirsten rat sarcoma virus (KRAS); guanine diphosphate (GDP); guanine nucleotide exchange factor (GEF); guanine triphosphate (GTP); mitogen-activated pathway kinase (MAPK).
Publication types
Grants and funding.
- RSP-248-18/19/Tour de Cure
The KRAS tour: Studying metabolic reprogramming in isogenic pancreatic cancer organoids.
Using an isogenic organoid platform to model pancreatic cancer, Duan et al. establish an important link between mutant KRAS and cholesterol metabolism and identify perhexiline maleate as a possible therapeutic to target this relationship.
Duke Scholars
Altmetric Attention Stats
Dimensions citation stats, published in, publication date, start / end page, related subject headings.
- Proto-Oncogene Proteins p21(ras)
- Pancreatic Neoplasms
- Metabolic Reprogramming
- Lipid Metabolism
- Developmental Biology
- 32 Biomedical and clinical sciences
- 31 Biological sciences
- 11 Medical and Health Sciences
RASDak Ride Across South Dakota
Ride across south dakota • june 2-7, 2024 what is rasdak.
RASDak stands for Ride Across South Dakota.
RASDak is an annual bicycle ride to enjoy the beautiful scenery and hospitality of South Dakota, the “Land of Infinite Variety.”
Our state is home to a variety of landscapes from rolling prairie to the Black Hills. The best way to experience these landscapes and South Dakota is by bike!
While the route changes every year, we focus on showcasing all of South Dakota’s charm and small town hospitality. Proceeds from the ride support organizations that help make RASDak possible. Many local church/school/youth organizations provide the rest stops, lunches, and dinners along the route and at our overnight locations. We also provide a $750 scholarship to one student at each of our overnight locations.
Search this Site
Friends of rasdak.
Organised By

- Stages Stage 1 02-19 Burjeel Holdings Stage 141 km Stage 2 02-20 Presight Stage 12.1 km Stage 3 02-21 Ras Al Khaimah Stage 176 km Stage 4 02-22 Dubai Stage 168 km Stage 5 02-23 Fujairah Stage 182 km Stage 6 02-24 Aldar Stage 138 km Stage 7 02-25 International Holding Company Stage 161 km
- Photo & Video
- The UAE Tour Ed. 2023
- The UAE Tour Ed. 2022
- The UAE Tour Ed. 2021
- The UAE Tour Ed. 2020
- The UAE Tour Ed. 2019
Lennert Van Eetvelt has turned the tables
- Press Conference
LENNERT VAN EETVELT HAS TURNED THE TABLES
Lennert van eetvelt wins the uae tour 2024, good morning from stage 7 of the uae tour, tim merlier breaks records in breakwater.
Jersey Wearers
Strive for glory
السعي للمجد, general time, lennert van eetvelt, lotto dstny, 22h 31' 18'', general points, tim merlier, soudal quick-step, intermediate sprint, mark stewart, discover the route, اكتشف المسار, burjeel holdings stage.
From Al Dhafra Walk
Presight Stage
From Hudayriyat Island
To Hudayriyat Island
Ras Al Khaimah Stage
From Al Marjan Island
To Jebel Jais
Dubai Stage
From Dubai Police Officer's Club
To Dubai Harbour
Fujairah Stage
From Al Aqah
To Umm Al Quwain
Aldar Stage
From Louvre Abu Dhabi
To Abu Dhabi Breakwater
International Holding Company Stage
From Bait Mohammed Bin Khalifa
To Jebel Hafeet
Don’t lose a moment
وسائل الإعلام
لا تضيع لحظة
كتاب السباق, photo gallery, video gallery, معرض الفيديو.
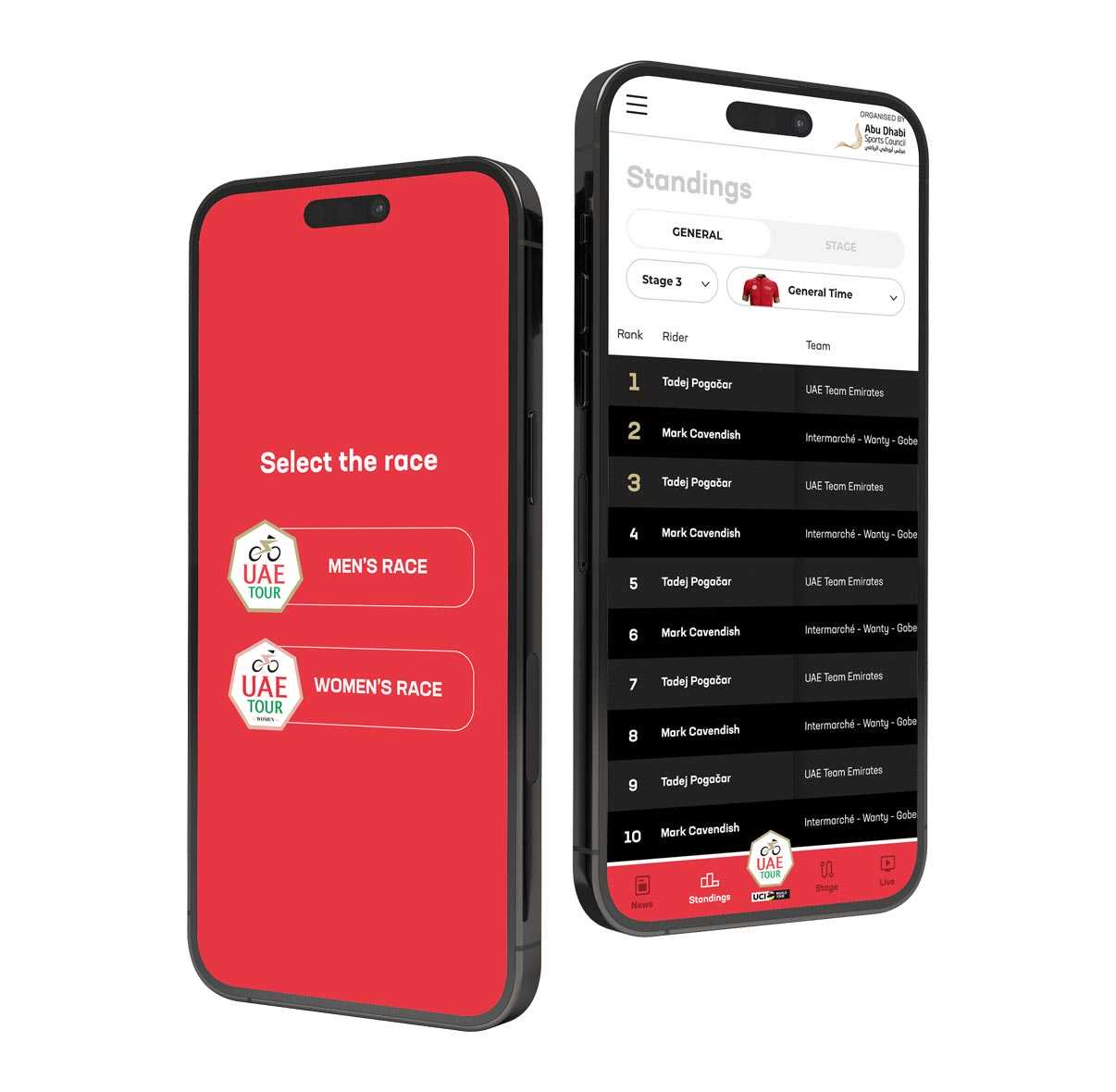
Official app
Download the official app of the uae tour.
This application for cycling fans makes it possible to view exclusive content: live, stages description, rankings, photos, videos and much more.
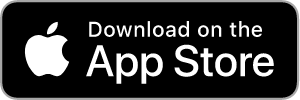
التطبيق الرسمي
نزل التطبيق الرسمي الخاص بطواف الإمارات.
يتيح هذا التطبيق لهواة رياضة ركوب الدراجات إمكانية مشاهدة المحتوى الحصري كالبث المباشر ووصف المراحل والترتيب والصور ومقاطع الفيديو وغيرها الكثير.
Tour de France 2023 route: Every stage of the 110th edition in detail
This year's race has kicked off in Bilbao, in Spain's Basque Country. It looks like it'll be a Tour for the climbers, with the Puy de Dôme returning and 56,400 metres of climbing in all
- Sign up to our newsletter Newsletter
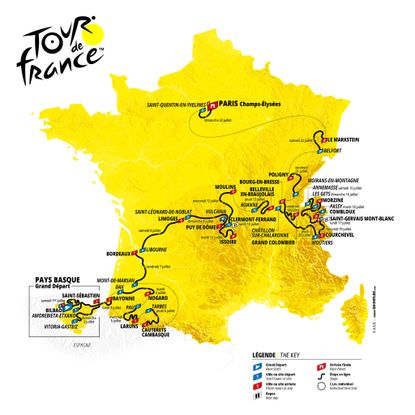
- Stage summary
- The stages in-depth
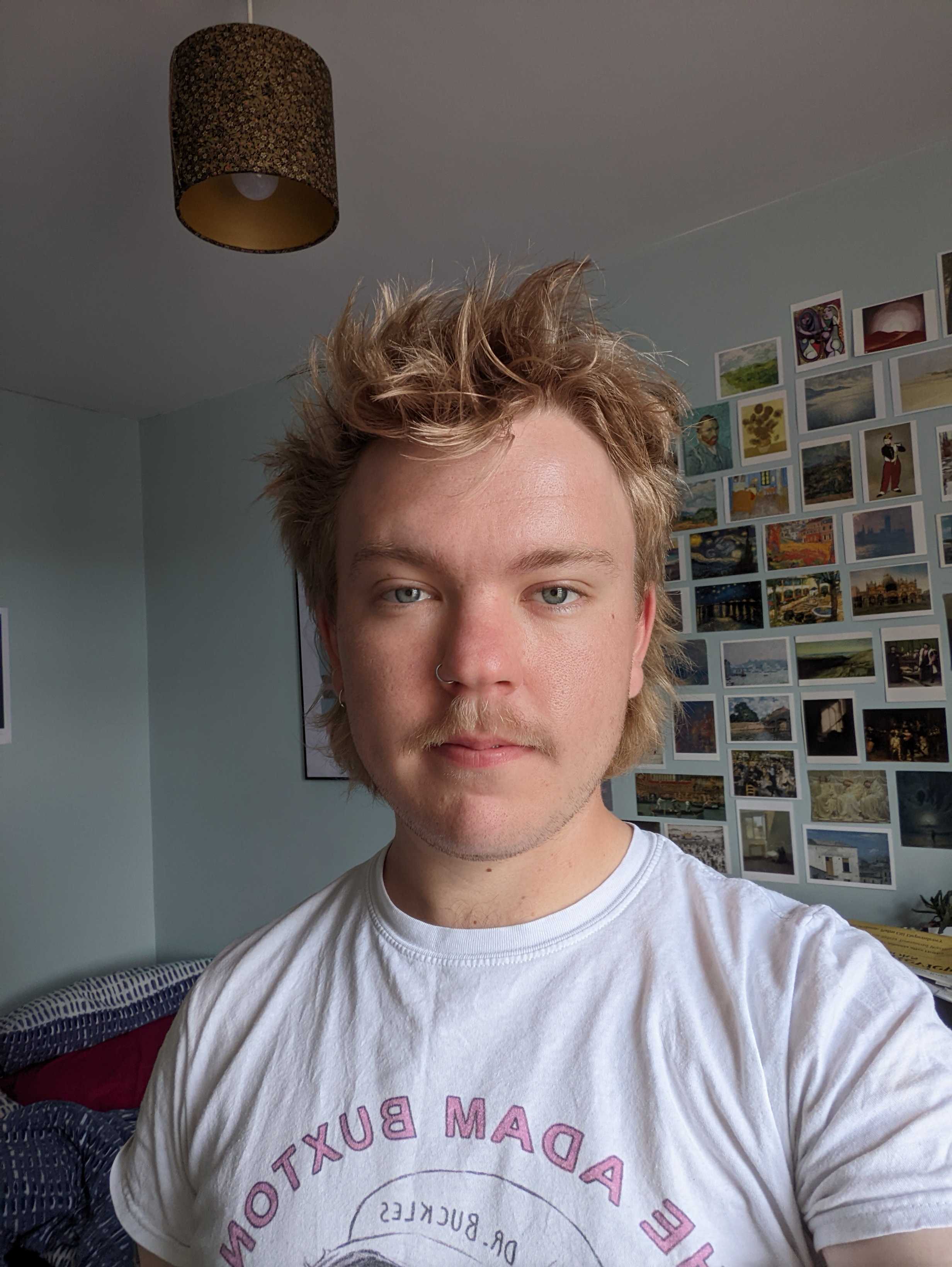
The 2023 men's Tour de France began in Bilbao, Spain on Saturday, July 1, with a route that looks set to be one for the climbers. It features four summit finishes, including a return for the iconic Puy de Dôme climb for the first time since 1988.
There is just one time trial across the three-week event, a short uphill race against the clock from Passy to Combloux over 22km. There are also returns for other epic climbs like the Col de la Loze and the Grand Colombier, with 56,400 metres of climbing on the Tour de France 2023 route.
The race started on foreign soil for the second year in a row, with a Grand Départ in the Spanish Basque Country , the setting for the race's 120th anniversary. There were two hilly stages in Spain, before the peloton crossed the border into France for a stage finish in Bayonne on day three.
After visiting Pau for the 74th time on stage five, the race's first real mountain test came on stage six, leaving Tarbes and cresting the Col d’Aspin and Col du Tourmalet before a summit finish in Cauterets.
On stage seven, the Tour’s second most visited city, Bordeaux, will welcome its first stage finish since 2010, when Mark Cavendish claimed his 14th of a record 34 stage wins. Leaving nearby Libourne the next day, stage eight will head east on a 201km slog to Limoges.
Before the first rest day, the riders will wind up to the summit of the Puy de Dôme, a dormant lava dome which hasn’t featured in the Tour for 35 years. They’ll then enjoy a well-earned day off in Clermont-Ferrand before continuing their passage through the Massif Central.
France’s national holiday, 14 July, will be celebrated next year with a summit finish on the Grand Colombier, the site of Tadej Pogačar ’s second stage win back in 2020. From there, the mountains keep coming. The riders will climb over the Col de Joux Plaine to Morzine on stage 14, before another mountaintop test in Saint-Gervais Mont-Blanc the next day.
Get The Leadout Newsletter
The latest race content, interviews, features, reviews and expert buying guides, direct to your inbox!
The sole individual time trial of the Tour de Franc route comes on stage 16, when a hilly 22km dash from Passy to Combloux will give the GC contenders a chance to force time gaps. The following day will bring the stage with the highest elevation gain, counting 5000m of climbing en route to the Courchevel altiport, via the Cormet de Roselend and the monstrous Col de la Loze.
On stages 18 and 19, the sprinters are expected to come to the fore, with flat finishes in Bourg-en-Bresse and Poligny.
The penultimate stage will play out in the country’s most easterly region, ascending the Petit Ballon, Col du Platzerwasel and finishing in Le Markstein, as the Tour de France Femmes did last year.
The riders will then undertake a 500km transfer to the outskirts of Paris for the curtain-closing stage. The final day will start at France’s national velodrome in Saint-Quentin-en-Yvelines, the track cycling venue for the 2024 Olympics, and will conclude with the customary laps of the capital’s Champs-Elysées.
The 2023 Tour de France will begin on 1 July, with the winner crowned in Paris on 23 July.
2023 Tour de France stage table
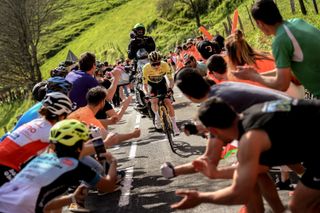
Jonas Vingegaard raced in the Basque Country this year
Tour de France route week summary
Tour de france week one.
The race began in Bilbao, starting in the Basque Country for the first time since 1992, when the Tour started in San Sebastian. The first two stages are packed full of climbs, with ten classified hills in over the opening couple of days, meaning there will be a fierce battle for the polka-dot jersey. Watch out for Basque fans going crazy on the roadside.
Stage three saw the race cross into France, which it will not leave for the rest of the 18 days. As expected we saw a sprint finish in Bayonne, even after four categorised climbs en-route. Nothing is easy this year.
The fourth day was another sprint, on a motor racing circuit in Nogaro, as the race moved, ominously, towards the Pyrenees. The Hors Categorie Col de Soudet on stage five was the first proper mountain of the race, and was followed by the Col de Marie Blanque, which has tough gradients. A GC day early on, although they are all GC days, really.
Stage five was a mountain top finish in Cauterets-Cambasque, but its gradients didn't catch too many out; it is the Col d'Aspin and Col du Tourmalet that will put people through it.
The seventh day of the race was a chance for the riders to relax their legs as the race headed northwest to an almost nailed-on sprint finish, before another opportunity for the the remaining fast men presented itself on stage eight - after two category four climbs towards the end, and an uphill finish.
The long first week of the race - which will have felt longer because last year had a bonus rest day - ended with the mythical Puy de Dôme.
Tour de France week two
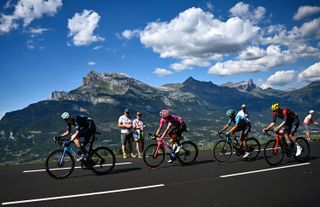
Magnus Cort in the breakaway on stage 10 of the Tour de France 2022
The second week begins with a lumpy road stage around Clermont-Ferrand, starting from a volcano-themed theme park. This will surely be a day for the break. The next day could also be one if the sprint teams fail to get their act together, with two early categorised climbs potential ambush points.
Back into the medium mountains on stage 12, with a finish in the wine making heartland of the Beaujolais, Belleville. Another day for the break, probably, but none of the five categorised climbs are easy.
The following day, stage 13, is France's national holiday, 14 Juillet. The Grand Colombier at the end of the day is the big attraction, with its slopes expected to cause shifts on the GC. Stage 14 is yet another mountain stage as the Tour really gets serious, with the Col de la Ramaz followed by the Col de Joux Plane. The latter, 11.6km at 8.5%, will be a real test for a reduced peloton, before a downhill finish into Morzine.
The final day of week two, stage 15, is yet another day in the Alps before a rest day in Saint-Gervais-Mont-Blanc. There is nothing as fearsome as the previous days, but 4527m of climbing should still be feared.
Tour de France week three
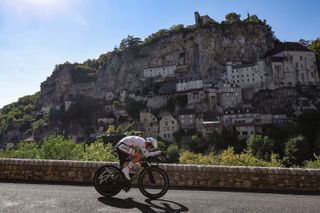
Tadej Pogačar in the final time trial at the 2022 Tour de France
The third and final week begins with the race's only time trial, 22km long and with a lot of uphill. It is not a mountain event, but it is certainly not one for the pure rouleurs .
Stage 17 looks like the race's Queen Stage, with the final climb up to the Col de la Loze looking incredibly tough on paper, and in real life. That follows the Col de Saisies, the Cormet de Roselend and the Côte de Longefoy, adding up to 5,100m of climbing. The race might be decided on this day.
After that, there is a nice day for the sprinters on stage 18, with a flat finish in Bourg-en-Bresse surely one for the fast men. The next day, stage 19 could be a breakaway day or a sprint finish, depending on how desperate teams are feeling, or how powerful the remaining leadout trains are.
The final mountainous day comes on the penultimate stage, with the men following the Femmes lead and finishing in Le Markstein. However, there's no Grand Ballon, just the Petit Ballon, and so unless something chaotic happens, there should not be great time switches on this stage.
Then, at last, there is the usual finish on the Champs-Élysées in Paris, after the race heads out of Saint-Quentin-en-Yvelines, which has a long-term deal to host the start of Paris-Nice too. ASO country.
Remember, this will be the last time Paris hosts the Tour de France until 2025. So, be prepared.
Tour de France 2023: The stages
Stage one: Bilbao to Bilbao (182km)
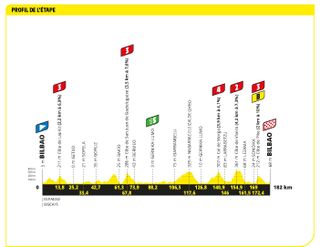
The opening stage is very lumpy
There was no easing into the Tour de France for the peloton this year, with a tough, punchy day in the Basque Country. Adam Yates took the first yellow jersey of the 2023 Tour de France after a scintillating stage in the Basque Country that saw the overall battle for the Tour take shape at the earliest opportunity.
The Briton emerged clear over the top of the final climb of the stage, the short and steep Côte de Pike, with his twin brother Simon a few seconds behind him. The pair worked well together to stay clear of the chasing bunch of GC contenders before Adam rode his brother off his wheel inside the final few hundred metres to claim victory.
Stage two: Vitoria-Gasteiz to Saint Sebastian (208.9km)
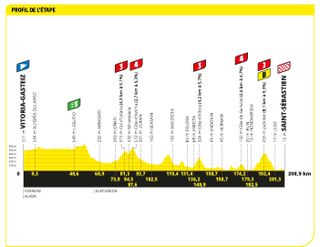
Still in the Basque Country, there is a Klasikoa theme to stage two
This was the longest stage of the Tour, surprisingly. Five more categorised climbs meant it was unlikely to be a sprint stage, including the Jaizkibel, famous from the Clasica San Sebastian, tackled on its eastern side 20km from the finish. This second stage from Vitoria Gasteiz to San Sebastian on the Basque coast followed many of the roads of the San Sebastian Classic, held here every summer.
An early break was soon established in the first 50km and established a three-minute advantage. However, the break was reeled in and a group, including the yellow jersey Adam Yates, pressed towards the finish with Wout Van Aert (Jumbo-Visma) clearly hoping it would finish in a sprint.
Victor Lafay (Cofidis) had other ideas however, and with all and sundry already having attacked Van Aert, Lafay finally made it stick with a kilometre to go, holding off the reduced bunch all the way to the line.
Stage three: Amorebiata-Etxano to Bayonne (187.4km)
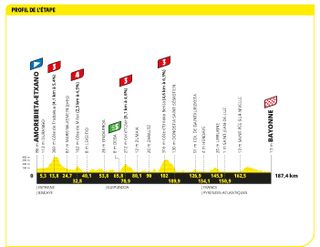
Still some hills, but this should be a sprint stage
The third stage took the riders from Amorebieta-Etxano in the Basque Country and back into France, finishing at Bayonne in what was always tipped to be a bunch sprint. Ultimately, despite a very strong showing in the leadout by Fabio Jakobsen's Soudal-Quick Step team, it was Jasper Philipsen who triumphed , having benefited from a deluxe leadout by team-mate Mathieu Van Der Poel.
Mark Cavendish, who is hunting for a record 35th stage win in what will be his final Tour de France, was sixth.
Stage four: Dax to Nogaro (181.8km)
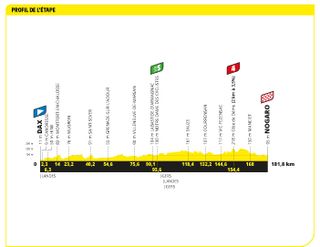
A nailed on bunch sprint, surely. Surely!
Now this one was always going to be a sprint finish, right? It finished on a motor racing circuit in Nogaro, meaning teams have a long old time to sort their leadout trains. After a sleepy day out all hell broke lose on the finishing circuit with a series of high speed crashes. Jasper Philipsen was one of the few sprinters to still have a lead-out man at his disposal and when that lead-out man is of the quality of Mathieu van der Poel he was always going to be very difficult to beat. So it proved with Australian Caleb Ewan chasing him down hard but unable to come around him. Philipsen's win handed him the green jersey too .
Stage five: Pau to Laruns (162.7km)
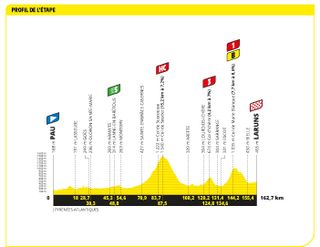
The first proper mountain, and the first sorting out, as early as stage five
The first Hors Categorie climb of the race came on stage five, the Col de Soudet, which is 15.2km at 7.2%, before the Col de Marie-Blanque and its steep gradients. It certainly ignited the GC battle!
A break that at one point contained 37 riders was never allowed more than a few minutes, but that proved unwise for Jonas Vingegaard and Tadej Pogačar behind. Ultimately, with the break already splintering on the final big climb – the Col de Marie-Blanque – Jai Hindley (Bora-Hansgrohe), riding his first Tour de France, attacked.
With Hindley time trialling the largely downhill 18km to the finish, Vingegaard attempted to chase him down – and put time into Pogačar as he did so.
Picking up strays from the early break on the way, Vingegaard got to within 34 seconds of Hindley, but it wasn't enough to stop the Australian from taking the stage win, and the yellow jersey .
Stage six: Tarbes to Cauterets-Cambasque (144.9km)
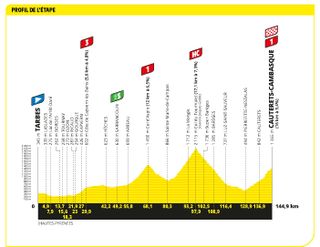
While in the Pyrenees, why not tackle a few more mountains?
A day of aggressive racing in the Pyrenees towards the first summit finish saw Jonas Vingegaard (Jumbo-Visma) take the yellow jersey but Tadej Pogačar (UAE Emirates) win the stage .
Having had his team set a blistering pace on the Col du Tourmalet, Vingegaard attacked with 4km until the summit. Only Pogačar could follow him as yellow jersey holder Jai Hindley dropped back to the peloton
Having joined up with super domestique Wout van Aert over the top, the group of favourites were towed up the first half of the final climb before Vingegaard attacked. Once again Pogačar followed and with two kilometers to go the Slovenian counter-attacked.
He clawed back nearly half a minute by the line, making the race for yellow a three horse race between those two and Hindley in the process.
Stage seven: Mont-de-Marsan to Bordeaux (169.9km)
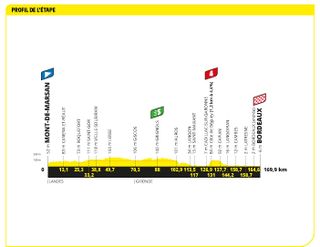
Bordeaux is always a sprint finish
Renowned as a sprint finish town, Bordeaux didn't disappoint the hopeful fastmen –except perhaps for Mark Cavendish, who had to concede victory to hat-trick man Jasper Philipsen, despite a very strong charge for the line from the Manxman .
With Cavendish hunting that elusive 35th record stage win, and having won here last time the Tour came visiting in 2010, many eyes were on the Astana Qazaqstan rider, with on-form Philipsen (Alpecin-Deceuninck) who has won twice already, starting as favourite.
The day began with Arkéa-Samsic's Simon Gugliemi forging what turned out to be a solo break that lasted 130 kilometres. He was joined by Pierre Latour (TotalEnergies) and Nans Peters (Ag2r-Citroën) halfway through the stage, the trio forming a purposeful triumvirate of home riders.
However, with the sprinters and their teams on the hunt and few places to hide on what was a hot day crammed with long, straight roads, the break served only as a placeholder for the day's main action in Bordeaux.
A technical finish with roundabouts aplenty, first Jumbo-Visma (in the service of GC leader Jonas Vingegaard) and then Alpecin-Deceuninck took the race by the scruff of the neck in the final. Philipsen enjoyed a marquee leadout from team-mate Mathieu Van Der Poel, but when Cavendish turned on the afterburners at around 150m and leapt forward, the whole cycling world held its breath.
That 35th stage win had to wait for another day though, with Philipsen sweeping past in what was yet another command performance from the Belgian.
Stage eight: Libourne to Limoges (200.7km)
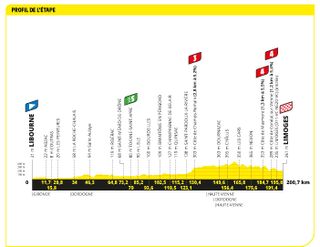
Three categorised climbs in the final 70km could catch people out
Mads Pedersen powered to victory up a punchy finish on stage eight of the Tour de France , managing to hold off green jersey Jasper Philipsen in the process.
Pedersen, the Lidl-Trek rider, now has two Tour stage wins to his name, in a finish which mixed pure sprinters and punchier riders. Alpecin-Deceuninck's Philipsen was third, with Wout van Aert (Jumbo-Visma) in third. To prove how mixed the top ten was, however, Tadej Pogačar (UAE Team Emirates) finished behind the likes of Corbin Strong (Israel-Premier Tech) and Bryan Coquard (Cofidis).
On a day which could have been one for the breakaway, the race was controlled expertly by Jumbo, Trek and Alpecin for their options, and so the escapees were never allowed much time. Sadly, stage eight turned out to Mark Cavendish's last - the Astana-Qazaqstan rider crashed heavily and was forced to abandon .
Stage nine: Saint-Léonard-de-Noblat to Puy de Dôme (184km)
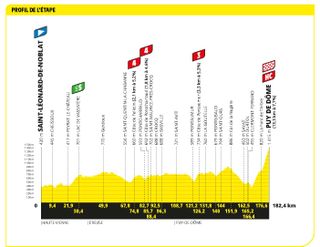
The Puy de Dôme is back, and is vicious
In a north American showdown it was Canada that came out on top as Michael Woods beat American rival Matteo Jorgenson to the win atop the legendary Puy de Dôme.
Jorgenson had gone solo form a breakaway with 40km left to race. However, on the slopes of the Puy de Dôme where the gradient remains over 105 for more than four kilometres, Woods closed the gap and came around Jorgenson with just 600m left to go.
In the final kilometre, of what had been a blisteringly hot day with temperatures north of 30 degree Celsius, Tadej Pogačar managed to drop Jonas Vingegaard but the Jumbo-Visma captain dug deep to minimise his losses and came across the line eight seconds down.
Stage 10: Vulcania to Issoire (162.7km)
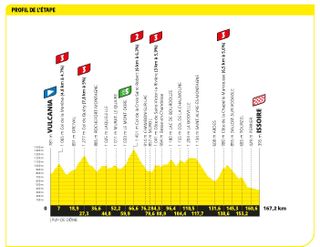
Five categorised climbs over this Volcanic stage
The breakaway had its day in Issoire, as Pello Bilbao (Bahrain Victorious) won beneath the scorching sun in the Auvergne-Rhône-Alpes region.
After a frantic start, the mood finally settled and a 14-rider move went clear. Krists Neilands (Israel Premier Tech) launched a solo bid with around 30km remaining, but was caught in the closing moments by a chasing group led by Bilbao. The Spaniard then policed attacks in the finale, before sprinting to his team's first victory at this year's race.
"For Gino," Bilbao said afterwards, dedicating his win to his late teammate, Gino Mäder .
Stage 11: Clermont-Ferrand to Moulins (179.8km)
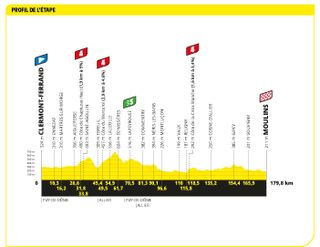
The flat finalé hints at a sprint, but it could be a break day
After a difficult previous day that was hot and hilly, the bunch allowed the break to go very quickly, with Andrey Amador, Matis Louvel and Daniel Oss quickly gaining three minutes. They were kept on a tight leash though, with the sprinters' teams eyeing a bunch finish. And this they delivered, with Jasper Philipsen winning a fourth stage after a tricky finale.
Stage 12: Roanne to Belleville-en-Beaujolais (168.8km)
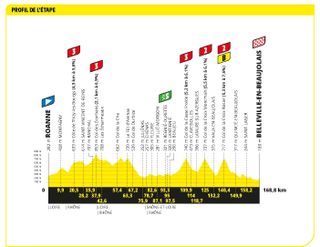
Hills return, with some steep, punchy ones towards the end
Just like stage ten, Thursday's stage 12 was a fast and frenetic affair on the road to Belleville-en-Beaujolais. A strong group of puncheur type riders eventually got up the road after the breakaway took more than 80 kilometres to form. Ion Izagirre (Cofidis) came out on top at the finish, soloing to the line after a big attack on the final climb of the day.
Stage 13: Châtillon-Sur-Chalaronne to Grand Colombier (138km)
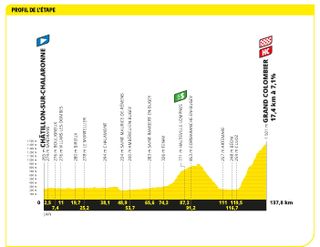
Welcome to the Alps, here's an hors categorie climb
Michał Kwiatkowski took an impressive solo victory on the summit finish of the Grand Colombier. The Polish rider caught and passed the remnants of the day's breakaway which included Great Britain's James Shaw to grab his second-ever Tour stage win. Behind the Ineos rider, Tadej Pogačar attacked and took eight seconds back on Jonas Vingegaard in the fight for the yellow jersey.
Stage 14: Annemasse to Morzine Les Portes du Soleil (151.8km)
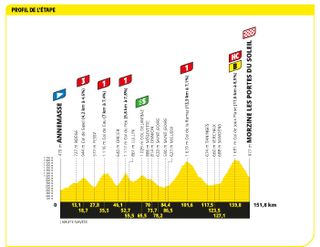
Five categorised climbs, four of which are one and above. Ouch.
Carlos Rodríguez announced himself on his Tour de France debut on stage 14 with a career-defining victory in Morzine. While all eyes were on Jonas Vingegaard and Tadej Pogačar, the Spaniard broke free on the descent of the Col de Joux Plane and descended as if on rails to the finish.
Stage 15: Les Gets Les Portes du Soleil to Saint-Gervais-Mont-Blanc (179km)
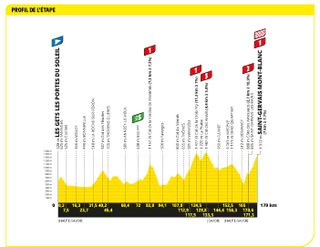
Back to a summit finish, there is no escape at this Tour
The breakaway had its day at the summit of Saint-Gervais Mont Blanc. After dedicating his career to domestique duties, the victory went to Wout Poels (Bahrain Victorious), who launched a late attack on the steepest slopes and held off Wout van Aert (Jumbo-Visma) to the line.
Stage 16: Passy to Combloux ITT (22.4km)
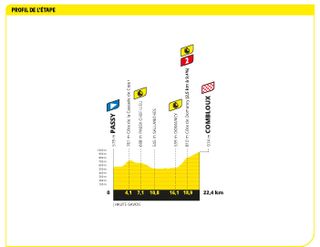
A time trial! But not a flat one
Stage 16 brought the fewest time trial kilometres at the Tour de France in 90 years. On the uphill test to Combloux, Jonas Vingegaard proved the strongest , and by quite a way, too. The Dane's winning margin of 1-38 over Tadej Pogačar left him in the driving seat to taking his second Tour title.
Stage 17: Saint-Gervais-Mont-Blanc to Courchevel (165.7km)
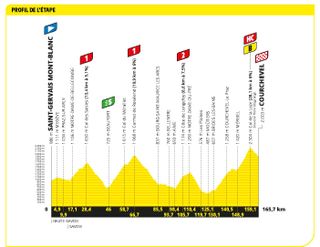
Back to the proper mountains, and there will be no let up on the final Wednesday
The Queen stage brought a career-defining victory for Austrian Felix Gall (AG2R Citroën), but all eyes were on the GC battle, and the demise of Tadej Pogačar. The UAE Team Emirates rider cracked on the slopes of the Col de la Loze, losing almost six minutes to Jonas Vingegaard, and slipping to 7-35 in the overall standings.
Stage 18: Moûtiers to Bourg-en-Bresse (184.9km)
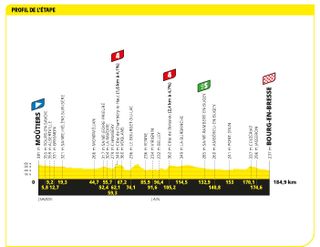
Two category four climbs on the road to a chicken-themed sprint
Denmark's Kasper Asgreen put in one of the best performances of the race to grab his first-ever Tour victory . The Soudal Quick-Step rider was part of a four man breakaway that managed to hold on all the way to the line by just a handful of seconds ahead of the peloton.
Stage 19: Moirans-en-Montagne to Poligny (172.8km)
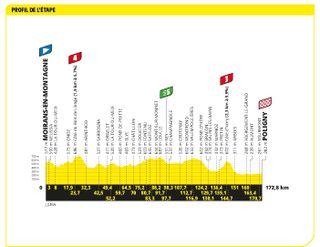
Another sprint, maybe, or a heartbreaking chase which fails to bring the breakaway back
Matej Mohorič of Bahrain Victorious took an emotional victory in Poligny after a chaotic day of racing. The Slovenian rider launched an attack with Kasper Asgreen and Ben O'Connor on the final climb of the hilly stage before beating his breakaway compatriots in a three-up sprint for the line. It was Mohorič's third-ever Tour victory.
Stage 20: Belfort to Le Markstein Fellering (133.5km)
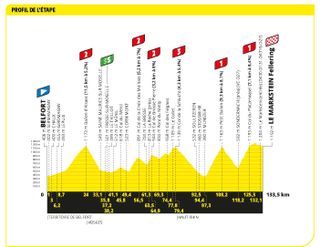
One last chance. Six categorised climbs, will it shake up the GC?
The race might be very near Germany at this point, but Belfort remained French after the Franco-Prussian War, unlike the territory the penultimate stage travels into.
This is the last chance saloon for all teams and riders who aren’t sprinters, especially those with GC ambitions. However, it is not quite the task of the previous Alpine days, with the six categorised climbs not the most testing. Still, there will be a lot of people trying to make things happen.
Stage 21: Saint-Quentin-en-Yvelines to Paris (115.1km)
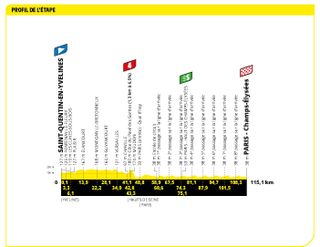
The classic Parisian sprint. Lovely.
This will be the last time the Tour heads to Paris until at least 2025, so make the most of those shots of the Arc de Triomphe and the Champs-Élysées. The classic procession will happen for the first 55km until the race hits the Champs for the first time 60km in. From that point on, anything goes, although that anything will probably be a bunch sprint.
Thank you for reading 20 articles this month* Join now for unlimited access
Enjoy your first month for just £1 / $1 / €1
*Read 5 free articles per month without a subscription
Join now for unlimited access
Try first month for just £1 / $1 / €1
Adam is Cycling Weekly ’s news editor – his greatest love is road racing but as long as he is cycling on tarmac, he's happy. Before joining Cycling Weekly he spent two years writing for Procycling, where he interviewed riders and wrote about racing. He's usually out and about on the roads of Bristol and its surrounds. Before cycling took over his professional life, he covered ecclesiastical matters at the world’s largest Anglican newspaper and politics at Business Insider. Don't ask how that is related to cycling.
- Tom Thewlis
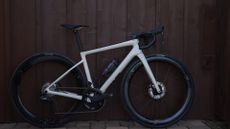
Like a race bike but more capable and comfortable, the Fray is the kind of road bike most of us ought to be riding
By Anne-Marije Rook Published 11 April 24
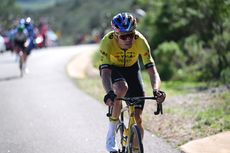
The Visma-Lease a Bike rider was set to ride the Italian Grand Tour for the first time
By Adam Becket Published 11 April 24
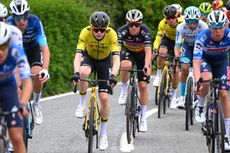
With Jonas Vingegaard, Remco Evenepoel and Primož Roglič hitting the deck at Itzulia Basque Country, all three now face battle to get their seasons back on track
By Adam Becket Published 9 April 24
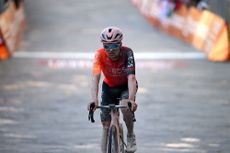
British rider crashed during recon of opening stage time trial last weekend and injured his right hip
By Tom Thewlis Published 3 April 24
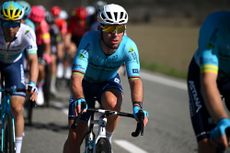
Cavendish will ride Presidential Cycling Tour of Türkiye later this month, Astana Qazaqstan confirms
By Tom Thewlis Published 2 April 24
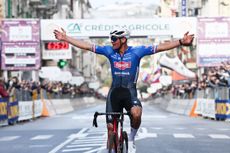
Dutchman starts his 2024 road season at Italian Monument on Saturday
By Tom Thewlis Published 15 March 24
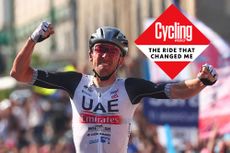
US star grabbed his first ever Grand Tour win at last year’s Giro d’Italia
By Tom Thewlis Published 8 March 24
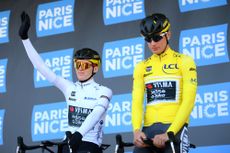
The American could step into the leaders yellow jersey on Tuesday evening after stage three’s team time trial in Auxerre
By Tom Thewlis Published 5 March 24
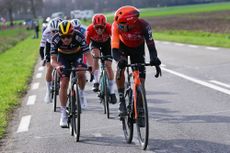
Colombian snapped up key bonus seconds in the general classification battle on run in to Les Mureaux
By Tom Thewlis Published 3 March 24
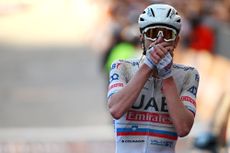
'He can still surprise us all the time’ João Almeida on Pogačar's performance in Tuscany
Useful links
- Tour de France
- Giro d'Italia
- Vuelta a España
Buyer's Guides
- Best road bikes
- Best gravel bikes
- Best smart turbo trainers
- Best cycling computers
- Editor's Choice
- Bike Reviews
- Component Reviews
- Clothing Reviews
- Contact Future's experts
- Terms and conditions
- Privacy policy
- Cookies policy
- Advertise with us
Cycling Weekly is part of Future plc, an international media group and leading digital publisher. Visit our corporate site . © Future Publishing Limited Quay House, The Ambury, Bath BA1 1UA. All rights reserved. England and Wales company registration number 2008885.
LIV Golf Masters Leaderboard: updates, scores for LIV tour as DeChambeau dominates
The Masters are here.
The first major tournament of the year started on Thursday, and nearly 90 players were at the Augusta National Golf Club in Augusta, Georgia to contend for a highly sought-after green jacket. Thirteen of them are LIV golfers.
Spanish golfer, LIV participant and 2023 Masters champion Jon Rahm will be looking to become the fourth golfer in Masters history to win back-to-back jackets and the first since Tiger Woods in 2001-02.
From NFL plays to college sports scores, all the top sports news you need to know every day.
Several other LIV golfers likely will be in contention for a Masters win by the end of the weekend as well, with Brooks Koepka, Dustin Johnson and up-and-comer Joaquín Niemann also among favorites.
Though it's red-hot American (and non-LIV golfer) Scottie Scheffler who wass the early favorite after winning his second consecutive Players Championship last month. He also won this year's Arnold Palmer Invitational just one week before his Players win. Scheffler played remarkably well on Day 1 as well, shooting 6-under on Day 1. Still, he sits in second place heading into Friday as LIV's Bryson DeChambeau leads all golfers at 7-under after Thursday.
Tiger Woods live: Updates, score and tracker for golf icon at Augusta on Day 1 Thursday
LIV Golfers Leaderboard at the Masters
- 1 - Bryson DeChambeau, -7
- T6 - Tyrell Hatton, -3
- T9 - Joaquín Niemann, -2
- T17 - Cameron Smith, -1
- T9 - Patrick Reed, -2
- T32 - Sergio García, E
- T32 - Brooks Koepka, E
- T42 - Phil Mickelson, +1
- T42 - Jon Rahm, +1
- T42 - Bubba Watson, +1
- T42 - Dustin Johnson, +1
- T54 - Charl Schwartzel, +2
- T85 - Adrian Meronk, +6
Masters Leaderboard: Full updated scores for Augusta leaders
Weather in Augusta, Georgia delays Masters start
The start of the Masters' first round was delayed Thursday morning due to rainy and windy conditions.
Thunderstorms are likely to end around 10 a.m. ET, and the first round is now scheduled to begin at 10:30 a.m. ET.
LIV Golfer standings after Day 1 of The Masters
- Bryson DeChambeau, -7
- Tyrell Hatton, -3
- Joaquin Niemann, -2
- Patrick Reed, -2
- Cameron Smith, -1
- Brooks Koepka, E
- Sergio Garcia, E
- Phil Mickelson, +1
- Jon Rahm, +1
- Bubba Watson, +1
- Dustin Johnson, +1
- Charl Schwartzel, +2
- Adrian Meronk, +6
LIV Golfer odds to win Masters 2024
Odds courtesy of BetMGM .
- 1. Jon Rahm (+1200)
- 2. Brooks Koepka (+1800)
- 3. Joaquín Niemann (+2800)
- 4. Bryson DeChambeau (+3300)
- T-5. Dustin Johnson, Cameron Smith (+4000)
- T-7. Tyrell Hatton, Patrick Reed (+6600)
- 9. Sergio García (+10000)
- T-10. Adrian Meronk, Phil Mickelson (+20000)
- 12. Bubba Watson (+25000)
- 13. Charl Schwartzel (+35000)
LIV Golfers who have won the Masters before
- Jon Rahm (2023)
- Dustin Johnson (2020)
- Patrick Reed (2018)
- Sergio García (2017)
- Bubba Watson (2012, 2014)
- Charl Schwartzel (2011)
- Phil Mickelson (2004, 2006, 2010)
2024 Masters Tournament schedule and how to watch
The Masters begins Thursday, April 11 and run through Sunday, April 14.
Round 1: Thursday, April 11
- Starting at 10:30 a.m. ET
- TV coverage: 3:00-7:30 p.m. ET
- Channel: ESPN
- Streaming: Masters.com (simulcast), CBSSports.com (desktop and mobile), CBS Sports App (desktop and mobile), ESPN+, Paramount+
How to watch: Catch Masters action with an ESPN+ subscription
Round 2: Friday, April 12
- Starting at 8:30 a.m. ET
Round 3: Saturday, April 13
- Starting at 10:00 a.m. ET
- TV coverage: 3:00-7:00 p.m. ET
- Channel: CBS
- Streaming: CBSSports.com (simulcast), Paramount+ (simulcast), CBS Sports app (simulcast)
Round 4: Sunday, April 14
- TV coverage: 2:00-7:00 p.m. ET
We occasionally recommend interesting products and services. If you make a purchase by clicking one of the links, we may earn an affiliate fee. USA TODAY Network newsrooms operate independently, and this doesn’t influence our coverage.
Gannett may earn revenue from sports betting operators for audience referrals to betting services. Sports betting operators have no influence over nor are any such revenues in any way dependent on or linked to the newsrooms or news coverage. Terms apply, see operator site for Terms and Conditions. If you or someone you know has a gambling problem, help is available. Call the National Council on Problem Gambling 24/7 at 1-800-GAMBLER (NJ, OH), 1-800-522-4700 (CO), 1-800-BETS-OFF (IA), 1-800-9-WITH-IT (IN). Must be 21 or older to gamble. Sports betting and gambling are not legal in all locations. Be sure to comply with laws applicable where you reside.
This article originally appeared on USA TODAY: LIV Golf Masters Leaderboard: updates, scores for LIV tour as DeChambeau dominates
Thank you for visiting nature.com. You are using a browser version with limited support for CSS. To obtain the best experience, we recommend you use a more up to date browser (or turn off compatibility mode in Internet Explorer). In the meantime, to ensure continued support, we are displaying the site without styles and JavaScript.
- View all journals
- My Account Login
- Explore content
- About the journal
- Publish with us
- Sign up for alerts
- Open access
- Published: 18 December 2023
The energetic and allosteric landscape for KRAS inhibition
- Chenchun Weng ORCID: orcid.org/0000-0002-5440-3783 1 ,
- Andre J. Faure ORCID: orcid.org/0000-0002-4471-5994 1 ,
- Albert Escobedo ORCID: orcid.org/0000-0001-7295-5111 1 &
- Ben Lehner ORCID: orcid.org/0000-0002-8817-1124 1 , 2 , 3 , 4
Nature volume 626 , pages 643–652 ( 2024 ) Cite this article
18k Accesses
1 Citations
286 Altmetric
Metrics details
- Computational biology and bioinformatics
- Drug discovery
- Systems biology
Thousands of proteins have been validated genetically as therapeutic targets for human diseases 1 . However, very few have been successfully targeted, and many are considered ‘undruggable’. This is particularly true for proteins that function via protein–protein interactions—direct inhibition of binding interfaces is difficult and requires the identification of allosteric sites. However, most proteins have no known allosteric sites, and a comprehensive allosteric map does not exist for any protein. Here we address this shortcoming by charting multiple global atlases of inhibitory allosteric communication in KRAS. We quantified the effects of more than 26,000 mutations on the folding of KRAS and its binding to six interaction partners. Genetic interactions in double mutants enabled us to perform biophysical measurements at scale, inferring more than 22,000 causal free energy changes. These energy landscapes quantify how mutations tune the binding specificity of a signalling protein and map the inhibitory allosteric sites for an important therapeutic target. Allosteric propagation is particularly effective across the central β-sheet of KRAS, and multiple surface pockets are genetically validated as allosterically active, including a distal pocket in the C-terminal lobe of the protein. Allosteric mutations typically inhibit binding to all tested effectors, but they can also change the binding specificity, revealing the regulatory, evolutionary and therapeutic potential to tune pathway activation. Using the approach described here, it should be possible to rapidly and comprehensively identify allosteric target sites in many proteins.
Similar content being viewed by others
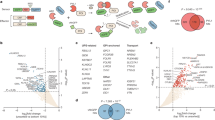
Proteome-scale discovery of protein degradation and stabilization effectors
Juline Poirson, Hanna Cho, … Mikko Taipale
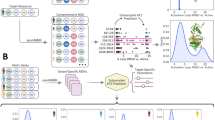
High-throughput prediction of protein conformational distributions with subsampled AlphaFold2
Gabriel Monteiro da Silva, Jennifer Y. Cui, … Brenda M. Rubenstein
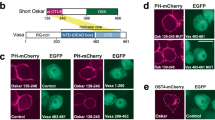
ReLo is a simple and rapid colocalization assay to identify and characterize direct protein–protein interactions
Harpreet Kaur Salgania, Jutta Metz & Mandy Jeske
The GTPase KRAS is somatically mutated in around 10% of all cancers, including about 90% of pancreatic adenocarcinoma, 40% of colorectal adenocarcinoma, 35% of lung adenocarcinoma and 20% of multiple myeloma 2 . KRAS functions as an archetypal molecular switch, cycling between inactive GDP-bound and active GTP-bound states. The altered conformation and activity of KRAS upon GTP binding is an example of allostery, the long-range transmission of information from one site to another in a protein 3 . Many structures of KRAS have been determined, revealing major (but variable) rearrangements in the switch-I and switch-II regions that allow binding to effector proteins in GTP-bound states 4 . KRAS effectors include the RAF proto-oncogene serine/threonine protein kinase (RAF1 (also known as CRAF)), phosphatidylinositol 4,5-bisphosphate 3-kinase catalytic subunit-γ isoform (PIK3CG) and the signalling protein RAL guanine nucleotide dissociation stimulator (RALGDS). Guanine nucleotide exchange factors such as SOS1 catalyse the release of GDP to activate KRAS whereas GTPase-activating proteins (GAPs) catalyse GTP hydrolysis to complete the cycle back to the inactive states. Cancer driver mutations interfere with this cycle, increasing the abundance of active GTP-bound effector-binding states 5 , 6 .
Despite its identification as an oncoprotein more than 40 years ago 7 , tens of thousands of scientific publications, and more than 300 published structures of KRAS 4 , only recently have inhibitors of KRAS been approved for clinical use, pioneered by sotorasib, a covalent binder of the driver mutation KRAS(G12C) 8 , 9 , 10 . Sotorasib is an allosteric inhibitor that binds outside of the nucleotide and effector binding sites to lock KRAS(G12C) in inactive GDP-bound states, reducing effector binding and clinically validating the efficacy of allosteric KRAS inhibition 8 , 10 . Similar to many other medically important proteins, the development of therapeutic agents that target KRAS is limited by the lack of information about inhibitory allosteric sites to target. Indeed, a comprehensive map of allosteric sites has not been generated for any oncoprotein or indeed for any disease target protein or any complete protein in any species.
Atlases of allosteric sites have the potential to greatly accelerate drug development, especially for the many human proteins considered undruggable because of the lack of an appropriate active site or because they function via difficult-to-inhibit protein–protein interaction interfaces. In addition, among other benefits, allosteric drugs often have higher specificity than orthosteric drugs that target conserved active sites 11 , 12 .
KRAS biophysics at scale
To comprehensively map inhibitory allosteric communication in KRAS, we applied a multidimensional deep mutational scanning approach 13 . We used two rounds of nicking mutagenesis 14 to construct three libraries of KRAS variants in which every possible single amino acid substitution is present not only in the wild-type KRAS (4B isoform, amino acids 1–188) but also in KRAS variants with a range of reduced activities (median of ten genetic backgrounds for each single mutant; Fig. 1a–d ). Quantifying the effects of the same mutations in different genetic backgrounds (here double amino acid substitutions) provides sufficient data to resolve biophysical ambiguities 15 and infer the causal biophysical effects of each mutation (see below). In total, the library consists of more than 26,500 variants of KRAS, including more than 3,200 single amino acid substitutions and more than 23,300 double amino acid substitutions.
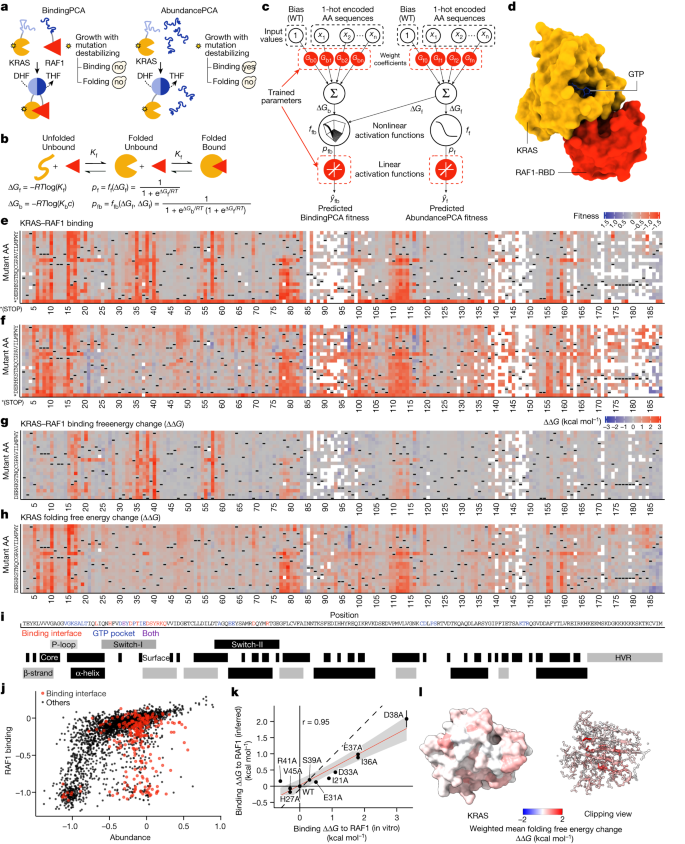
a , Overview of ddPCA selections. Yes, yeast growth; no, yeast growth defect; DHF, dihydrofolate; THF, tetrahydrofolate. b , Three-state equilibrium and corresponding thermodynamic model. ∆ G f , Gibbs free energy of folding; ∆ G b , Gibbs free energy of binding; K f , folding equilibrium constant; K b , binding equilibrium constant; c , binding partner concentration; p f , fraction folded; p fb , fraction folded and bound; f f , nonlinear function of ∆ G f ; f fb , nonlinear function of ∆ G f and ∆ G b ; R , gas constant; T , temperature in Kelvin. c , Neural network architecture used to fit thermodynamic models to the ddPCA data (bottom, target and output data), thereby inferring the causal changes in free energy of folding and binding associated with single amino acid substitutions (top, input values). AA, amino acid; WT, wild type. d , 3D structure of KRAS bound to the RAF1 RBD (RAF1-RBD) (Protein Data Bank (PDB) ID: 6VJJ ). e , f , Heat maps of fitness effects of single amino acid substitutions for KRAS–RAF1 from BindingPCA ( e ) and AbundancePCA ( f ) assays. White spaces indicate missing values; dashes are wild-type amino acids; asterisk indicates a stop codon. g , h , Heat maps showing inferred changes in free energies of binding ( g ) and folding ( h ). i , Sequence and annotation of KRAS. Binding interface is defined by RAF1 distance <5 Å; GTP pocket is defined by GTP or Mg 2+ distance <5 Å; core is defined by relative accessible surface area < 0.25; based on PDB ID: 6VJJ . P-loop, residue numbers 10–17; switch-I: 25–40; switch-II: 58–76; α-helix 1: 15–24; α-helix 2: 67–73; α-helix 3: 87–104; α-helix 4: 127–136; α-helix 5: 148–166; β-strand 1: 3–9; β-strand 2: 38–44; β-strand 3: 51–57; β-strand 4: 77–84; β-strand 5: 109–115; β-strand 6: 139–143. j , Scatter plot comparing abundance and binding fitness of single amino acid substitutions. Substitutions in the binding interface are indicated in red. k , Comparisons of model-inferred free energy changes to in vitro measurements 40 . Error bars indicate 95% confidence intervals from a Monte Carlo simulation approach ( n = 10 experiments). Linear regression fit and its 95% confidence interval are shown as a red solid line and a grey shaded area, respectively. Pearson’s r is shown. Black dashed line indicates y = x . l , 3D structure (left) and clipping view (right) of KRAS with residues coloured by the weighted mean folding free energy change.
We first quantified the binding of these KRAS variants to the RAS-binding domain (RBD) of the oncoprotein effector RAF1. Binding was quantified using a protein-fragment complementation assay 13 , 16 , 17 (BindingPCA). Binding fitness was highly correlated among three independent replicate selections (Pearson’s r > 0.9; Extended Data Fig. 1a ) to previous data that used a different binding assay in a different cellular context 18 , 19 (Pearson’s r = 0.82; Extended Data Fig. 1c ) and to individual growth measurements (Pearson’s r = 0.94; Extended Data Fig. 1d ).
As expected, mutations in the RAF1-binding interface strongly inhibit binding, as do variants in the nucleotide-binding pocket (Fig. 1e,i ). However, 2,019 out of 3,231 single amino acid substitutions reduce binding to RAF1 (false discovery rate (FDR) = 0.05, two-sided z -test), including many outside of the interface and in the hydrophobic core of the protein (Extended Data Fig. 1e ). This strongly suggests that many changes in binding to RAF1 are caused by changes in the abundance of folded KRAS and not by altered binding affinity 13 , 20 .
From phenotypes to free energy changes
To disentangle the effects of mutations on KRAS folding and binding, we used a second selection assay, AbundancePCA 13 , 21 , to quantify the cellular abundance of the KRAS variants. We refer to this combined approach of BindingPCA and AbundancePCA as ‘doubledeepPCA’ 13 (ddPCA). Plotting the RAF1 binding of each variant against its cellular abundance shows that many changes in binding can indeed be explained by reduced KRAS abundance (Fig. 1j ). However, inspection of Fig. 1j also reveals that a substantial number of variants have effects on binding that are much larger than can be accounted for by their reduced abundance, including many variants in the binding interface (red dots in Fig. 1j ).
Protein folding and binding relate to changes in the free energies of folding (∆ G f ) and binding (∆ G b ) by nonlinear functions derived from the Boltzmann distribution 13 , 20 (Fig. 1b ). Typically, many different combinations of folding and binding energy changes could underlie a measured change in binding due to a mutation. ddPCA is an efficient experimental design to generate sufficient data to infer en masse the causal biophysical effects of mutations. There are three key principles of the approach. First, mutational effects are quantified for multiple phenotypes—here the binding of KRAS to RAF1 and the abundance of KRAS in the absence of RAF1. Second, mutational effects are not only quantified in wild-type proteins but also in genetic backgrounds with altered folding and/or binding energies—here our libraries contain a median of ten double mutants for each single amino acid substitution in KRAS. Third, the data are used to fit a thermodynamic model in which free energy changes due to mutations combine additively in energy space (but not additively for the measured molecular phenotypes; Methods ).
We biased the choice of genetic backgrounds in our KRAS library to mutations with weak detrimental effects and used MoCHI, a substantially improved flexible package to fit user-defined mechanistic models to deep mutational scanning data using neural networks 22 , to fit a three-state (unfolded KRAS, folded KRAS and bound KRAS) thermodynamic model to the data (Fig. 1c , Extended Data Fig. 1f–k and Methods). The fitted model predicts the double amino acid mutant data held out during training very well (abundance median R 2 = 0.74, binding median R 2 = 0.91; Extended Data Fig. 1f,g,i,j ) strongly supporting the assumption that most free energy changes combine additively in doubles and these inferred free energy changes are highly correlated with in vitro measurements (Pearson’s r = 0.95; Fig. 1k ). Evaluating model performance on a held out test replicate gave similar results (abundance median R 2 = 0.54, binding median R 2 = 0.87; Extended Data Fig. 1h,k ).
The RAF1-binding interface
In total, 2,241 out of 3,453 single amino acid substitutions are detrimental to folding and 843 out of 3,301 are detrimental to binding (FDR = 0.05; Fig. 1g,h ). Mutations detrimental to folding are enriched in the hydrophobic core of the protein (odds ratio (OR) = 1.92, P < 10 −16 ; Fig. 1h,l , two-sided Fisher’s exact test; Supplementary Video 1 ). By contrast, mutations that increase the binding free energy are strongly enriched in the binding interface (OR = 6.02, P < 10 −16 ; Figs. 1g and 2a ), with the mean absolute binding free energy changes upon mutation at each site identifying the binding interface (Fig. 2b,c and Supplementary Video 2 , receiver operating curve area under curve (ROC-AUC) = 0.8 compared with ROC-AUC = 0.65 when using the mean absolute binding fitness).
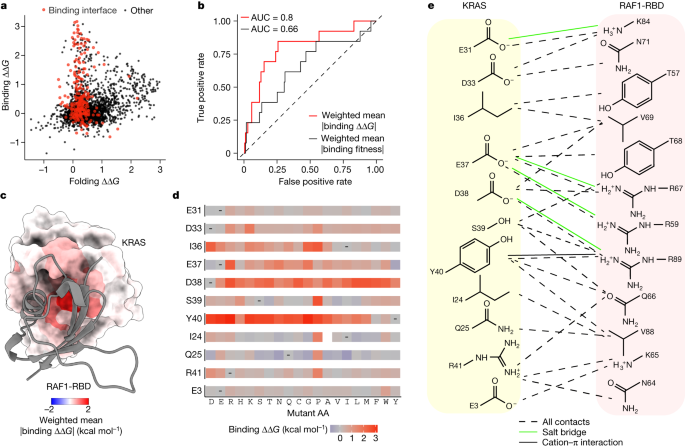
a , Scatter plot comparing binding and folding free energy changes of single amino acid substitutions. b , Receiver operating curves (ROCs) for predicting binding interface residues (RAF1 distance < 5 Å) using weighted mean absolute binding ∆∆ G (red) or using weighted mean absolute binding fitness (black). AUC, area under the curve. Dashed line at y = x indicates performance of a random predictor. c , 3D structure of KRAS bound to RAF1 in which residue atoms are coloured by the position-wise weighted mean absolute change in the free energy of binding to RAF1. RAF1-RBD is shown in grey ribbon. d , Heat maps of binding free energy changes in RAF1-binding interface residues. e , Direct contacts between KRAS and RAF1.
The interface residues that are most important for RAF1 binding include a mixture of charged (E37 and D38) and hydrophobic (I36 and Y40) residues. D38 cannot be changed to any other amino acid without detrimental effects on binding affinity, revealing a requirement for both negative charge and a particular side chain length at this site (Fig. 2d,e ). By contrast, E37 can be replaced by D (shortening the side chain but retaining the negative charge) and also by Y, F or H, suggesting that the salt bridge to RAF1 R67 can be replaced by an alternative interaction involving an aromatic side chain. Y40 can only be replaced by F, revealing the importance of the aromatic side chain which makes a cation–π interaction with RAF1 R89. I36 makes two hydrophobic contacts with RAF1, and whereas polar mutations at this position are detrimental, multiple hydrophobic substitutions are tolerated. Mutations at other residues that contact RAF1 are much better tolerated, indicating that these sites are less important for binding. For example, mutations at D33 tend to be mildly detrimental, with only charge-reversing mutations to R and K and mutation to P strongly inhibiting binding. Similarly, charge-reversing mutations and mutation to P are also most detrimental at R41, whereas mutations at the other two charged sites (E31 and E3) at the edge of the interface generally have little effect on the binding free energy.
Allosteric landscape for RAF1 binding
We next considered mutations outside of the binding interface. In total, there are 361 distal mutations in 74 residues that increase the binding free energy to a greater extent than the average effect of mutations in the RAF1-binding interface (∆∆ G b greater than the weighted mean absolute binding free energy change of substitutions in binding interface residues, FDR = 0.05; Fig. 3a ). Allosteric mutations defined in this manner are highly enriched in the physiological allosteric site of KRAS, the nucleotide-binding pocket (157 mutations in 13 residues, OR = 7.68, P < 10 −16 , two-sided Fisher’s exact test).
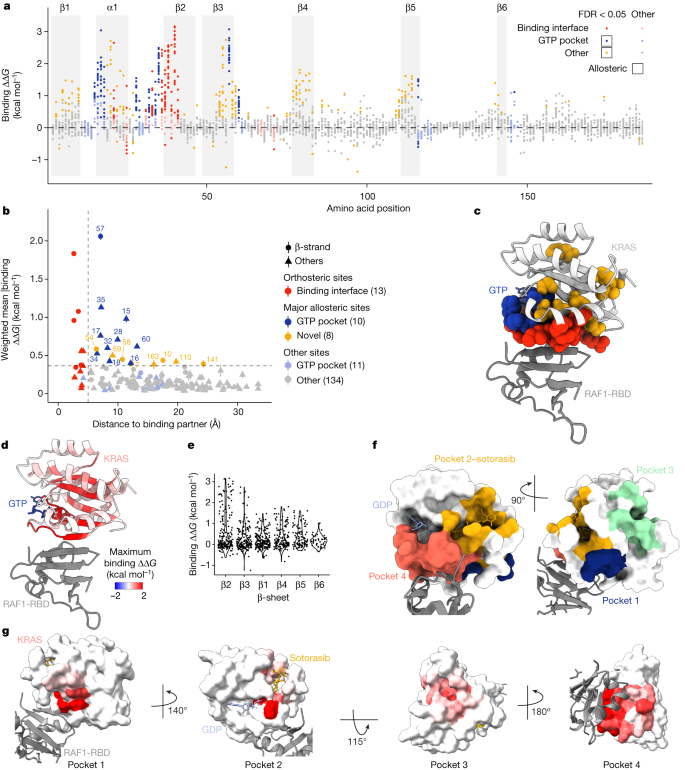
a , Manhattan plot showing the binding free energy changes of all single amino acid substitutions. Dots are coloured according to residue position and whether the corresponding binding ∆∆ G is significantly greater than the weighted mean absolute binding ∆∆ G of all mutations in the RAF1-binding interface (two-sided z -test, FDR = 0.05). b , Relationship between the position-wise average absolute change in free energy of binding to RAF1 and the minimal side chain heavy atom distance to RAF1. Major allosteric sites are defined as non-binding-interface residues with weighted mean absolute change in free energy of binding higher than the average of binding-interface residue mutations (horizontal dashed line). Error bars indicate 95% confidence intervals ( n ≥ 10). c , 3D structure (PDB ID: 6VJJ ) of KRAS bound to RAF1 (grey) with binding interface and major allosteric site residue atoms of KRAS coloured as in b . d , Similar to c , except KRAS residues are coloured by maximum binding ∆∆ G . e , Violin plot showing the decay of binding free energy change across successive strands in the β-sheet. β-strands are ordered by increasing distance to RAF1 in the 3D structure. f , 3D structure alignment (PDB IDs: 6OIM and 6VJJ ) of KRAS bound to GDP (blue), sotorasib (yellow) and RAF1 (grey) with KRAS surface coloured according to previously described pockets in KRAS. Pocket 2, sotorasib distance < 5 Å; pockets 1, 3 and 4 (ref. 23 ); pocket 1, residues 5–7, 39, 54–56 and 70–75; pocket 2, residues 61–65 and 90–94; pocket 3, residues 97, 101, 107–111, 136–140 and 161–166; pocket 4, residues 17, 21, 24–40 and 57. g , Similar to f except KRAS pockets are coloured by maximum binding ∆∆ G .
Enhanced allosteric communication
We first focused on residues in which many different mutations have strong allosteric effects. Defining major allosteric sites as residues where the mean absolute change in binding free energy upon mutation is equal to or greater than that in binding interface residues identifies a total of 18 sites (Fig. 3b,c ). Ten of these major allosteric sites are located in the physiological allosteric site—the nucleotide-binding pocket (Fig. 3b,c ). The additional eight major allosteric sites are residues V7, G10, D54, T58, A59, P110, F141 and I163 (Fig. 3b ). Three of these residues are close to the binding interface, with D54 being adjacent to the binding interface and T58 and A59 connecting the binding interface to the nucleotide-binding pocket (Fig. 3c and Supplementary Video 3 ).
Notably, 5 of the 8 novel major allosteric residues are located in the central (and only) 6-stranded β-sheet of KRAS (Fig. 3b,c , OR = 5.24, P = 2.8 × 10 −2 , two-sided Fisher’s exact test). Within the β-sheet, the binding free energy changes are largest for mutations in residues in the first strand that contacts RAF1 and they progressively decrease in each subsequent strand of the sheet (Fig. 3d,e , Extended Data Fig. 2a–c and Supplementary Video 4 ). This decay of the strength of allosteric effects across the sheet is consistent with local energetic propagations that underlie allosteric communication. A similar, yet weaker, distance-dependent decay is observed for residues outside of the β-sheet (Extended Data Fig. 2c ). Propagation appears more efficient across the sheet than along the backbone within a strand, with residues in the first strand that do not contact RAF1 being depleted for allosteric mutations (Fig. 3a and Extended Data Fig. 2b , OR = 0.16, P = 10 −3 , two-sided Fisher’s exact test). Allosteric communication therefore seems to be particularly effective across the central β-sheet of KRAS.
KRAS has four active surface pockets
We next considered the effects of mutations in the surface residues of KRAS, focusing on the four previously described structural pockets in addition to the nucleotide-binding pocket 23 (Fig. 3f and Supplementary Video 5 ).
Pocket 1 (also called the switch-I/II pocket) is located behind switch-II between the central β-sheet and α-helix 2 and is the binding site for multiple inhibitors that are effective in pre-clinical models 24 , 25 . Many mutations in pocket 1 allosterically inhibit RAF1 binding (57 mutations in 10 residues, FDR = 0.05; Fig. 3f and Extended Data Fig. 2d ), consistent with the demonstrated ability of pocket 1 engagement to inhibit effector binding.
Pocket 2 (also called the switch-II pocket) is located between switch-II and α-helix 3 and is the binding site of sotorasib and other clinically approved allosteric inhibitors of KRAS(G12C) 26 . Seventy-one mutations in nine residues that contact sotorasib allosterically inhibit RAF1 binding (Fig. 3g and Extended Data Fig. 2e ). Thus, mutations and small molecules binding to pocket 1 and pocket 2 can allosterically inhibit KRAS activity.
Pocket 3 of KRAS is located in the C-terminal lobe of the protein and is the most distant pocket from the RAF1-binding interface (Fig. 3f,g ). The effects of pocket 3 engagement are not well described 23 and pocket 3 has received little attention for therapeutic development 24 . However, our data reveal that pocket 3 is allosterically active, with 20 mutations in 6 residues in pocket 3 inhibiting binding to RAF1 (Fig. 3g and Extended Data Fig. 2f ). The effects of mutations located in pocket 3 were validated in individual growth assays (Pearson’s r = 0.94; Extended Data Fig. 1d ). We also validated the effects on in vitro binding to RAF1 of an allosteric mutation in pocket 3 (P110F), as well as a mutation in an additional newly discovered major allosteric site (A59R) (Extended Data Fig. 2h ). Despite its distance from the effector-binding interface, our data show that pocket 3 should be prioritized as a site for the development of KRAS inhibitors.
Finally, pocket 4, which is located immediately behind the flexible effector-binding loop, contains 105 allosteric mutations in 9 residues that do not contact RAF1 (Fig. 3g and Extended Data Fig. 2g ). Our data therefore validate all four surface pockets of KRAS as allosterically active, with perturbations in all pockets having large inhibitory effects on RAF1 binding. This is a strong argument for the development of molecules targeting all four pockets as potential KRAS inhibitors.
Energetic maps for six KRAS interactions
Similar to most oncoproteins, KRAS binds many different proteins as part of its physiological and disease-relevant functions 3 . Many of these interaction partners bind a common surface of KRAS—the effector-binding interface—making KRAS an interesting model of multispecificity in molecular recognition 3 . To our knowledge, the effects of mutations on binding energies for multiple interaction partners have not been comprehensively profiled for any protein. Moreover, quantifying KRAS binding to multiple interaction partners provides an opportunity to quantify the conservation and specificity of allosteric effects in a signalling hub (Fig. 4a ).
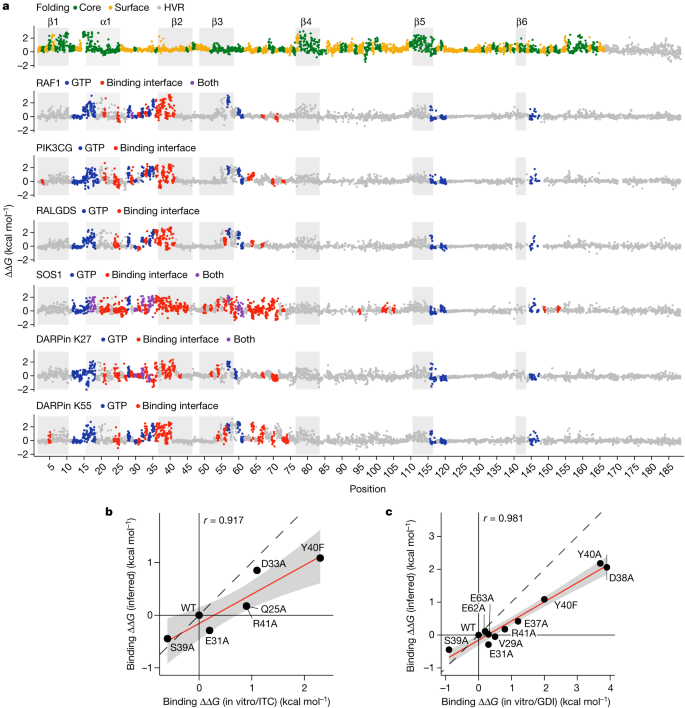
a , Manhattan plots showing the folding and binding free energy changes of all single amino acid substitutions. Dark grey rectangles indicate β-strands, light grey rectangles indicate α-helix 1. HVR, hypervariable region. Binding interface is defined by indicated binding partner distance < 5 Å. PDB IDs: RAF1, 6VJJ ; PIK3CG, 1HE8 ; RALGDS, 1LFD ; SOS1, 1NVW ; DARPin K27, 5O2S ; DARPin K55, 5O2T . b , c , Comparisons of binding free energy changes to in vitro measurements by isothermal titration calorimetry (ITC) ( b ) and guanine nucleotide dissociation inhibition assay (GDI) ( c ). Linear regression fit and its 95% confidence interval are shown as a red solid line and a grey shaded area, respectively. Pearson’s r is shown. Black dashed line indicates y = x . Error bars indicate 95% confidence intervals from a Monte Carlo simulation approach ( n = 10 experiments).
We quantified the binding of the more than 26,000 KRAS variants to six interaction partners: the three KRAS effector proteins RAF1, PIK3CG and RALGDS, the guanine nucleotide exchange factor SOS1, and two DARPins, K27 and K55 (synthetic antibody-like molecules selected to bind GDP-bound KRAS and GTP-bound KRAS, respectively). The structures of all six complexes have been determined 27 , 28 , 29 , 30 , 31 .
The data for all six binding selections were highly reproducible (Extended Data Figs. 1a and 3a ), and we used MoCHI to simultaneously fit a thermodynamic model to the molecular phenotypes of the variants in all seven experimental datasets (Extended Data Fig. 3b,c and Methods). Each single amino acid change in KRAS therefore has seven associated free energy changes: six binding energies and one folding energy (Fig. 4a and Extended Data Fig. 4a ). As for RAF1 (Fig. 1k ), the MoCHI binding energies for RALGDS correlate extremely well with independent in vitro measurements (Fig. 4b,c ). The binding energies identify the known binding surfaces on KRAS, including the two known interfaces for SOS1 (ref. 31 ) (Fig. 2b and Extended Data Fig. 4b , median ROC-AUC = 0.80, range = 0.68–0.89 for weighted mean binding energies and median ROC-AUC = 0.64, range = 0.54-0.75 for weighted mean binding fitness measurements).
These seven free energy landscapes constitute more than 22,000 thermodynamic measurements, which is similar in scale to the number of measurements made for proteins in the entire scientific literature 32 .
Specificity in binding interfaces
We first considered how mutations in the binding interfaces alter binding to the six interaction partners. All six proteins bind KRAS through an overlapping set of contacts (Fig. 5a–c ). This sharing of contacts is particularly pronounced for the three effector proteins, RAF1, PIK3CG and RALGDS (Fig. 5a ). Comparing the mutational effects reveals that whereas some residues are critically important for binding to all three proteins, many substitutions alter the binding specificity (Fig. 5d ). For example, many mutations in the negatively charged residues D33 and D38 and the hydrophobic residues I36 and Y40 strongly inhibit binding to all three effectors. However, a subset of hydrophobic substitutions at I36 inhibits binding to PIK3CG and RALGDS but not to RAF1 and substitution of L56 to negatively charged residues specifically increases binding to RAF1 while retaining binding to PIK3CG but inhibiting binding to RALGDS (Fig. 5d ). By contrast, many substitutions at E37 inhibit binding to RAF1 and RALGDS but increase binding to PIK3CG. Mutating Y64 inhibits binding to PIK3CG and RALGDS but allows binding to RAF1. At S39 a subset of hydrophobic mutations inhibit binding to PIK3CG and RAF1 but not to RALGDS. Comparing the binding free energies for all six binding partners reveals a high diversity of specificity changes that can be reached through single amino acid substitutions (Extended Data Fig. 5 ).
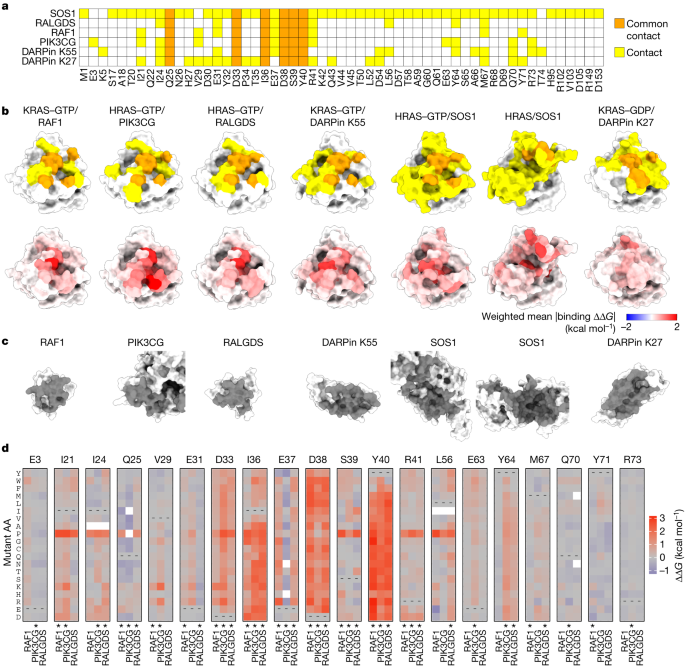
a , Common and unique structural contacts between KRAS and the indicated binding partner. b , 3D structures of KRAS indicating binding partner contacts (top row, coloured as in a ) and weighted mean absolute binding free energy change (bottom row). c , 3D structures of binding partners with binding interface indicated in grey. PDB IDs: RAF1, 6VJJ ; PIK3CG, 1HE8 ; RALGDS, 1LFD ; DARPin K55, 5O2T ; SOS1, 1NVW ; DARPin K27, 5O2S . d , Heat maps of binding free energy changes in interface residues contacting at least one of the three effectors (RAF1, PIK3CG and RALGDS). Asterisks indicate binding interface residues for each partner.
Allosteric maps of six KRAS interactions
We next considered the specificity of mutational effects outside of the binding interfaces. We first focused on the positions that are most enriched for allosteric mutations for each interaction, defining the major allosteric sites for each interaction as those in which the average absolute binding free energy change is as large or greater than the average across mutations in all the binding interfaces (Fig. 6a ). Novel major allosteric sites were identified for all six binding partners, with a median of 9 major allosteric sites in the nucleotide-binding pocket and a median of 5.5 additional major allosteric sites for each interaction (Fig. 6a ).
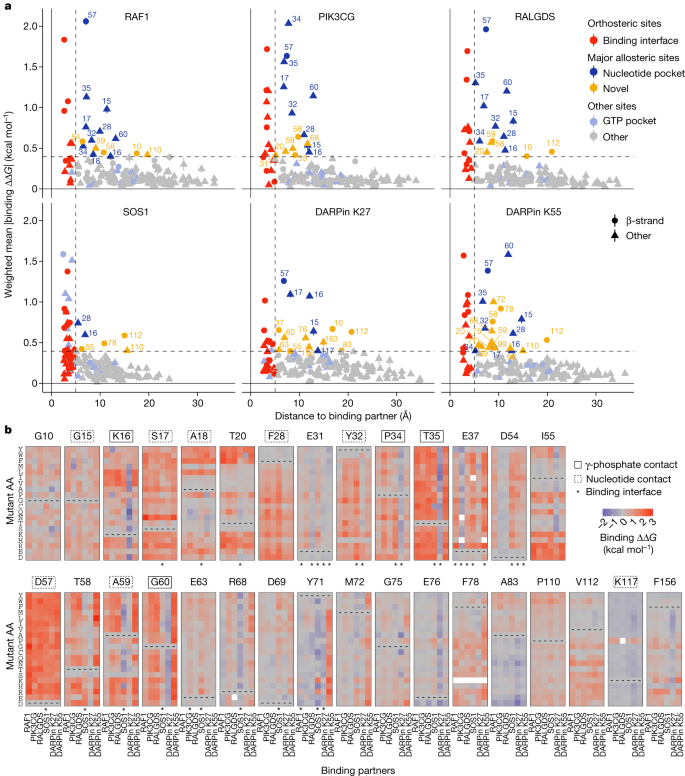
a , Relationship between the weighted mean absolute change in free energy of binding and the distance to each corresponding binding partner (minimal side chain heavy atom distance). Major allosteric sites are defined as non-binding-interface residues with weighted mean absolute change in free energy of binding higher than the average of binding-interface-residue mutations across all binding partners (horizontal dashed line). Error bars indicate 95% confidence interval ( n ≥ 10). b , Heat maps of binding free energy changes in all major allosteric sites. Nucleotide pocket and γ-phosphate-contacting residues are indicated.
We then compared the binding free energy changes between all six interaction partners for all mutations in these positions (Fig. 6b ). Many substitutions at G10, G15, S17, D57, F78, P110 and V112 inhibit all six interactions (Fig. 6b and Extended Data Fig. 6a ). Substitutions of F28 to non-aromatic amino acids inhibit all six interactions, as do many changes to charged amino acids at I55 and to hydrophobic amino acids at A18 and A83 (Fig. 6b ). Substitutions to P at I55, A59, R68, K117 and F156 inhibit at least five interactions (Fig. 6b and Extended Data Fig. 6a ). Considering all mutations outside of the binding interface, allosteric mutations are enriched at G, P, F and T residues for four out of six partners and depleted at charged residues for six out of six partners. Allosteric mutations are also enriched for substitutions to P for six out of six partners and to R for five out of six partners (Extended Data Fig. 6b ). The enrichment for allosteric mutations at G residues and for substitutions to P is also observed in three small protein domains 13 .
Allosteric control of binding specificity
That multiple mutations at many of the allosteric sites inhibit binding to all interaction partners suggests that engagement of these sites is likely to generally inhibit KRAS function. However inspection of Fig. 6b also reveals sets of mutations in the major allosteric sites that have more specific allosteric effects. Particularly notable examples are many mutations in residues K16, I55, G60 and F156 that allosterically inhibit binding to most KRAS interaction partners but allosterically increase binding to the DARPin K27 (Fig. 6b ). The DARPin K27 specifically recognizes inactive GDP-bound KRAS, and so mutations at these sites are likely to favour GDP-binding states. Consistent with this, K16 and G60 directly contact the γ-phosphate of GTP. Many substitutions of E76 also increase binding to DARPin K27 but with little effect on the other interactions. Additional examples include mutations at Y71 and M72 that specifically inhibit binding to DARPin K55 and mutations at D54 that inhibit four interactions but retain or enhance binding to PIK3CG and RALGDS (Fig. 6b ). In addition, outside of these major allosteric sites there are many other mutations that allosterically alter both the binding affinity and specificity of KRAS (Extended Data Fig. 7 ).
Here we presented a global map of inhibitory allosteric sites for KRAS and a comprehensive comparative map of the effects of mutations on the free energies of binding of KRAS to multiple interaction partners. The dataset constitutes more than 22,000 free energy measurements, a rich resource for protein biophysics and computational biology.
KRAS is one of the most frequently mutated genes in cancer and one of the most sought after and valuable therapeutic targets. Our results reveal a number of principles concerning allosteric communication in KRAS. First, KRAS has many inhibitory allosteric sites. Second, most allosteric mutations inhibit binding to all three KRAS effectors, revealing the potential to broadly inhibit KRAS activity. Third, allosteric mutations are enriched close to binding sites, suggesting local energetic propagation as the main allosteric mechanism. Fourth, allosteric communication is anisotropic, with communication being particularly effective across the central β-sheet of KRAS. Fifth, mutations can also allosterically control binding specificity, suggesting the potential for regulatory, evolutionary and therapeutic modulation of signalling bias. Sixth, all four surface pockets of KRAS are allosterically active, with particularly notable effects of mutations in the distal pocket 3. The comprehensive allosteric map therefore genetically validates all four pockets as suitable for therapeutic targeting and focuses attention on the largely ignored pocket 3.
The KRAS effector interface—similar to many protein surfaces—has to recognize structurally diverse proteins. Comprehensive mutagenesis of this surface shows that its evolution is constrained by fitness trade-offs, with mutations that increase binding to one protein typically having antagonistic pleiotropic effects on binding to others. However, the binding specificity of KRAS is highly evolvable, with single amino acid substitutions causing a diversity of specificity changes. These altered binding profiles can be useful experimental tools, providing ‘edgetic’ perturbations 33 to test the functions of individual molecular interactions and their combinations 33 , 34 .
In our experiments, we quantified mutational effects in wild-type KRAS. To test how well these effects are conserved in KRAS carrying oncogenic driver mutations, we reconstituted activation of RAF1 binding by driver mutations in yeast by co-expressing the catalytic domain of a human GAP, RASA1 (Extended Data Fig. 8a–i ). Mutational effects in oncogenic KRAS were highly correlated to those in wild-type KRAS in the absence and presence of human GAP co-expression (for example, Pearson’s r between wild-type KRAS and KRAS(G12C) in the presence of human GAP co-expression is 0.93, n = 776; Extended Data Fig. 8 ). A second potential caveat of our experiments was that we quantified binding of KRAS to isolated RBDs and, in general, mutations that have allosteric effects in isolated domains may have different effects or directly participate in binding in full-length proteins. However we found that changes in binding to full-length RAF1 were highly correlated to those to the RAF1 RBD (Pearson’s r = 0.94, n = 1,186 genotypes), as were the inferred binding free energy changes (Pearson’s r = 0.89, n = 1,195; Extended Data Fig. 9 ). Finally, we note that there are likely to be multiple molecular mechanisms that mediate the allosteric effects, including shifts in conformational equilibria, altered nucleotide binding or hydrolysis, and propagated structural and dynamic perturbations in the binding interfaces. Further experiments will be needed to disentangle the mechanistic causes of allostery.
The accelerated pace of human genetics research means we now know the proteins to therapeutically target in hundreds of human diseases 35 . However, effective therapies have been developed against a small minority of these genetically validated targets. In short, the protein targets for many diseases are known, but we do not know how to target them. For most proteins, the location of the ‘switches’ to target with drugs to turn activity off or on remain unknown. If we could find these switches, we would be able to develop drugs to control their activity.
The data presented here and in other recent studies 13 , 36 , 37 , 38 , 39 have revealed that allosteric sites are much more prevalent than is widely appreciated. Moreover, the approach that we have applied here to KRAS is quite general and can be used to identify allosteric sites in many different proteins. We believe that this general strategy can be used to systematically map regulatory sites that can be used to target many important proteins. Mapping of allosteric sites is likely to have an increasingly important role in drug development, laying the foundations for therapeutically targeting proteins that were previously considered to be undruggable.
Media and buffers
The following media and buffers were used and prepared as follows. Luria-Bertani (LB) medium: 10 g l −1 Bacto-tryptone, 5 g l −1 yeast extract, 10 g l −1 NaCl; autoclaved 20 min at 120 °C. Yeast peptone dextrose adenine (YPDA): 20 g l −1 glucose, 20 g l −1 peptone, 10 g l −1 yeast extract, 40 mg l −1 adenine sulfate; autoclaved 20 min at 120 °C. Sorbitol medium (SORB): 1 M sorbitol, 100 mM lithium acetate, 10 mM Tris pH 8.0, 1 mM EDTA; filter sterilized (0.2 mm nylon membrane, Thermo Scientific). Plate mixture: 40% PEG3350, 100 mM lithium acetate, 10 mM Tris-HCl pH 8.0, 1 mM EDTA pH 8.0; filter sterilized. Recovery medium: yeast peptone dextrose (20 g l −1 glucose, 20 g l −1 peptone, 10 g l −1 yeast extract), 0.5 M sorbitol. Filter sterilized. Synthetic complete medium without uracil (SC-URA): 6.7 g l −1 yeast nitrogen base without amino acid, 20 g l −1 glucose, 0.77 g l −1 complete supplement mixture drop-out without uracil; filter sterilized. Synthetic complete medium without uracil, methionine and adenine (SC-URA/MET/ADE): 6.7 g l −1 yeast nitrogen base without amino acid, 20 g l −1 glucose, 0.74 g l −1 complete supplement mixture drop-out without uracil, adenine and methionine; filter sterilized. Competition medium: SC-URA/MET/ADE + 200 μg ml −1 methotrexate (BioShop Canada), 2% DMSO. DNA extraction buffer: 2% Triton X-100, 1% SDS, 100 mM NaCl, 10 mM Tris-HCl pH 8, 1 mM EDTA pH 8.
Plasmid construction
Two generic plasmids were constructed to be able to assay any protein of interest by BindingPCA or AbundancePCA: the BindingPCA plasmid (pGJJ161) and the AbundancePCA plasmid (pGJJ162).
The BindingPCA plasmid (pGJJ161) and AbundancePCA plasmid (pGJJ162) were derived from the previous BindingPCA plasmid (pGJJ001) and the previous AbundancePCA plasmid (pGJJ045) 13 . The C-terminus (GGGGS)4 linker of DHFR3 were changed to the N terminus, which allowed us to fuse the protein of interest to the N terminus of the DHFR3 fragment in both abundance and BindingPCA assays.
One KRAS AbundancePCA plasmid, 7 BindingPCA plasmids, one BindingPCA co-expression RASGAP (the catalytic domain of human RASA1) plasmid and one KRAS mutagenesis plasmid are used in this paper. To construct the KRAS AbundancePCA plasmid (pGJJ271), the sequence of full-length KRAS (188 amino acids) was amplified from a plasmid, a gift from the L. Serrano laboratory, using primer pair oGJJ231/oGJJ232 (Supplementary Table 1 ). This primer pair also introduced the HindIII and NheI restriction sites. The PCR product was digested by HindIII and NheI, then was cloned into the digested pGJJ162 plasmid using T4 Ligase (NEB). To construct 7 KRAS BindingPCA plasmids, a common KRAS BindingPCA plasmid (pGJJ317) was constructed by ligating full-length KRAS sequence digested by HindIII and NheI to digested BindingPCA plasmid. 7 BindingPCA plasmids are constructed by ligating each binding partners PCR product which was digested by BamHI and SpeI to digested pGJJ317 using T4 Ligase (NEB). To construct RAF1 BindingPCA plasmid (pGJJ336), the sequence of RAF1-RBD (52–131) was amplified from the cDNA of 293 T cell line using primer pair oGJJ74/oGJJ307 which also introduced the BamHI and SpeI restriction sites. To construct PI3KCG BindingPCA plasmid (pGJJ565), the sequence of PIK3CG RBD (203–312) was amplified from R777-E169 Hs.PIK3CG (Addgene) using primer pair oWCC169/oWCC170. To construct RALGDS BindingPCA plasmid (pGJJ400), the sequence of RALGDS RBD (778–864) was amplified from R777-E169 Hs.PIK3CG (Addgene) using primer pair oWCC28/oWCC29. To construct SOS1 BindingPCA plasmid (pGJJ541), the sequence of SOS1 (564–1049) was amplified from plasmid R777-E317 Hs.SOS1 (Addgene) using primer pair oWCC149/oWCC150. To construct DARPin K27 BindingPCA plasmid (pGJJ553), the sequence of DARPin K27 was amplified from plasmid pCASP-SptP120-K27-HilA (Addgene) using primer pair oWCC157/oWCC158. To construct DARPin K55 BindingPCA plasmid (pGJJ554), the sequence of DARPin K55 was amplified from plasmid pCASP-SptP120-K55-HilA (Addgene) using primer pair oWCC159/oWCC160. To construct full-length RAF1 BindingPCA plasmid (pGJJ623), the sequence of full-length RAF1 (amino acids 1 to 648) was amplified from a gene block synthesized by IDT (Integrated DNA Technologies) using primer pair oWCC252/oWCC253. To construct the BindingPCA co-expression RASGAP plasmid, the cyc1 promoter-driven RASGAP cassette was amplified in four fragments, cyc1 promoter from AbundancePCA plasmid (pGJJ271) using primer pair oWCC182/oWCC183, two fragments of RASGAP (amino acids 714 to 1047) were amplified from ORFeome plasmid (81020C02, Protein Technologies Unit, CRG) using primer pairs oWCC184/oWCC97, and oWCC96/oWCC129, cyc1 terminator was amplified from AbundancePCA plasmid (pGJJ271) using primer pair oWCC128/oWCC140, which were then assembled by Gibson reaction (Protein Technologies Unit, CRG) at 50 °C for 1 h with RAF1-RBD BindingPCA plasmid (pGJJ336) which was digested by NgoMIV. To construct the KRAS mutagenesis plasmid (pGJJ380), pGJJ191 plasmid was constructed firstly which contained a streptomycin resistance gene cassette. The pGJJ191 plasmid was amplified in two fragments: one ori cassette which also contained AvrII and HindIII restriction sites using primer pair oGJJ308/oGJJ309, the other streptomycin resistance gene cassette using primer pair oGJJ310/oGJJ311, which were then assembled by Gibson reaction at 50 °C for 1 h. The KRAS sequence was digested by AvrII and HindIII from AbundancePCA plasmid and ligated into digested pGJJ191. Then a BbvCI restriction site was introduced using primer pair oWCC51/oWCC52.
Mutagenesis library construction
The plasmid-based one-pot saturation (nicking) mutagenesis protocol was used in this study 14 . KRAS are divided to three blocks in order to be fully sequenced by Illumina paired-end 150 NextSeq pipeline.
An initial single round of nicking mutagenesis using equimolar mixes of degenerate KRAS primers (Supplementary Table 2 ) was obtained for two reasons: (1) To obtain random single mutants to use as template for another round of nicking mutagenesis (by randomly selecting single colonies and verified by Sanger sequencing); and (2) to quantify the degenerate primer positional bias and compensate for it in the shallow double mutant libraries.
To construct three final KRAS libraries, an equimolar pool of single mutants of each block and wild type were used as the plasmid template for a round of nicking mutagenesis. The mutants were chosen based on their varying binding affinities to RAF1 (refs. 18 , 19 ), ensuring a range of affinities within the mutant pools (block 1: T2K, V14S, L6H, E37G, Y40A, D38C, L19P, Q61L, E63V; block 2: I84L, F82S, L113F, Y71F, K101R, A66P, M72G, F78W, E63V, V112N; block 3: K176C, R149V, L133A, Y137K, L159A and A146F). Additionally, the mutants of interest (G12C, G12D, G12V, S17N and T35S) were also included in block 1. To compensate for the extreme positional biases, each mutagenic primer was mixed in the pool inversely to the mean read counts per position from these first-round nicking libraries.
The libraries midi preps were digested with HindIII and NheI restriction enzymes and the insert containing the mutated protein was gel purified (MinElute Gel Extraction Kit, QIAGEN) to be later cloned into the AbundancePCA plasmid and BindingPCA plasmids by temperature-cycle ligation. The AbundancePCA plasmid and BindingPCA plasmids were all digested by HindIII and NheI enzymes and purified using the QIAquick Gel Extraction Kit (QIAGEN). The assembly of AbundancePCA libraries and BindingPCA libraries were done overnight by temperature-cycle ligation using T4 ligase (New England Biolabs) according to the manufacturer’s protocol, 67 fmol of backbone and 200 fmol of insert in a 33.3 μl reaction. The ligation was desalted by dialysis using membrane filters for 1 h and later concentrated 3.3× using a SpeedVac concentrator (Thermo Scientific).
All concentrated assembled libraries were transformed into NEB 10β High-efficiency Electrocompetent Escherichia coli cells according to the manufacturer’s protocol (volumes used in each library specified in Supplementary Table 3 ). Cells were allowed to recover in SOC medium (NEB 10β Stable Outgrowth Medium) for 30 min and later transferred to 200 ml LB medium with ampicillin 4× overnight. The total number of estimated transformants for each library can be found in Supplementary Table 3 . One-hundred millilitres of each saturated E. coli culture were collected next morning to extract the plasmid library using the QIAfilter Plasmid Midi Kit (QIAGEN).
Methotrexate selection assays
The methotrexate selection assay protocol was described in a previous study 13 . The high-efficiency yeast transformation protocol was scaled in volume depending on the targeted number of transformants of each library. The transformation protocol described below (adjusted to a pre-culture of 175 ml of YPDA) was scaled up or down in volume as reported in Supplementary Table 3 .
For each of the selection assays (3 blocks × 6 BindingPCA + 3 blocks × 1 AbundancePCA), 3 independent pre-cultures of BY4742 were grown in 20 ml standard YPDA at 30 °C overnight. The next morning, the cultures were diluted into 175 ml of pre-warmed YPDA at an optical density at 600 nm (OD 600 ) of 0.3. The cultures were incubated at 30 °C for 4 h. After growth, the cells were collected and centrifuged for 5 min at 3,000 g , washed with sterile water and later with SORB medium (100 mM lithium acetate, 10 mM Tris pH 8.0, 1 mM EDTA, 1 M sorbitol). The cells were resuspended in 8.6 ml of SORB and incubated at room temperature for 30 min. After incubation, 175 μl of 10 mg ml −1 boiled salmon sperm DNA (Agilent Genomics) was added to each tube of cells, as well as 3.5 μg of plasmid library. After gentle mixing, 35 ml of plate mixture was added to each tube to be incubated at room temperature for a further 30 min. DMSO (3.5 ml) was added to each tube and the cells were then heat shocked at 42 °C for 20 min (inverting tubes from time to time to ensure homogeneous heat transfer). After heat shock, cells were centrifuged and resuspended in ~50 ml of recovery medium and allowed to recover for 1 h at 30 °C. Next, cells were again centrifuged, washed with SC-URA medium and resuspended in SC-URA (volume used in each library found in Supplementary Table 3 ). After homogenization by stirring, 10 μl were plated on SC-URA petri dishes and incubated for ~48 h at 30 °C to measure the transformation efficiency. The independent liquid cultures were grown at 30 °C for ~48 h until saturation. The number of yeast transformants obtained in each library assay can be found in Supplementary Table 3 .
For each of the BindingPCA or AbundancePCA assays, each of the growth competitions was performed right after yeast transformation. After the first cycle of post-transformation plasmid selection, a second plasmid selection cycle (input) was performed by inoculating SC-URA/MET/ADE at a starting OD 600 = 0.1 with the saturated culture (volume of each experiment specified in Supplementary Table 3 ). Cells were grown for 4 generations at 30 °C under constant agitation at 200 rpm (selection time of each experiment specified in Supplementary Table 3 ). This allowed the pool of mutants to be amplified and enter the exponential growth phase. The competition cycle (output) was then started by inoculating cells from the input cycle into the competition medium (SC-URA/MET/ADE + 200 μg ml −1 methotrexate) so that the starting OD 600 was 0.05. For that, the adequate volume of cells was collected, centrifuged at 3,000 rpm for 5 min and resuspended in the pre-warmed output medium. Meanwhile, each input replicate culture was split in two and collected by centrifugation for 5 min at 5,000 g at 4 °C. Yeast cells were washed with water, pelleted and stored at −20 °C for later DNA extraction. After ~4 generations of competition cycles, each output replicate culture was split in two and collected by centrifugation for 5 min at 5,000 g at 4 °C, washed twice with water and pelleted to be stored at −20 °C.
DNA extractions and plasmid quantification
The DNA extraction protocol used was described previously 13 . A 50 ml collected culture of OD 600 ≈ 1.6 is described below. Cell pellets (one for each experiment input or output replicate) were resuspended in 1 ml of DNA extraction buffer, frozen by dry ice-ethanol bath and incubated at 62 °C water bath twice. Subsequently, 1 ml of phenol:chloroform:isoamyl alcohol 25:24:1 (equilibrated in 10 mM Tris-HCl, 1 mM EDTA, pH 8) was added, together with 1 g of acid-washed glass beads (Sigma Aldrich) and the samples were vortexed for 10 min. Samples were centrifuged at room temperature for 30 min at 4,000 rpm and the aqueous phase was transferred into new tubes. The same step was repeated twice. Three molar sodium acetate (0.1 ml) and 2.2 ml of pre-chilled absolute ethanol were added to the aqueous phase. The samples were gently mixed and incubated at −20 °C for 30 min. After that, they were centrifuged for 30 min at full speed at 4 °C to precipitate the DNA. The ethanol was removed and the DNA pellet was allowed to dry overnight at room temperature. DNA pellets were resuspended in 0.6 ml TE 1× and treated with 5 μl of RNaseA (10 mg ml −1 , Thermo Scientific) for 30 min at 37 °C. To desalt and concentrate the DNA solutions, QIAEX II Gel Extraction Kit was used (50 µl of QIAEX II beads). The samples were washed twice with PE buffer and eluted twice by 125 µl of 10 mM Tris-HCI buffer, pH 8.5 and then the two elutions were combined. Finally, plasmid concentrations in the total DNA extract (that also contained yeast genomic DNA) were quantified by quantitative PCR using the primer pair oGJJ152/oGJJ153, that binds to the ori region of the plasmids.
Sequencing library preparation
The sequencing library preparation protocol was described previously 13 . The sequencing libraries were constructed in two consecutive PCR reactions. The first PCR (PCR1) was designed to amplify the mutated protein of interest and to increase the nucleotide complexity of the first sequenced bases by introducing frame-shift bases between the adapters and the sequencing region of interest. The second PCR (PCR2) was necessary to add the remainder of the Illumina adapter and demultiplexing indexes.
To avoid PCR biases, PCR1 of each independent sample (input/output replicates of any of the yeast assays) was run with an excess of plasmid template 20–50 times higher than the number of expected sequencing reads per sample. Each reaction started with a maximum of 1.25 × 10 7 template plasmid molecules per microlitre of PCR1, avoiding introducing more yeast genomic DNA that interfered with the efficiency of the PCR reaction. For this reason, PCR1s were scaled up in volume as specified in Supplementary Table 3 . The PCR1 reactions were run using Q5 Hot Start High-Fidelity DNA Polymerase (New England Biolabs) according to the manufacturer’s protocol, with 25 pmol of pooled frame-shift primers as specified in Supplementary Table 1 for different blocks (forward and reverse primers were independently pooled according to the nucleotide diversity of each oligonucleotide, Supplementary Table 1 ). The PCR reactions were set to 60 °C annealing temperature, 10 s of extension time and run for 15 cycles. Excess primers were removed by adding 0.04 μl of ExoSAP-IT (Affymetrix) per microlitre of PCR1 reaction and incubated for 20 min at 37 °C followed by an inactivation for 15 min at 80 °C. The PCRs of each sample were then pooled and purified using the MinElute PCR Purification Kit (QIAGEN) according to the manufacturer’s protocol. DNA was eluted in EB to a volume six times lower than the total volume of PCR1.
PCR2 reactions were run for each sample independently using Hot Start High-Fidelity DNA Polymerase. The total reaction of PCR2 was reduced to half of PCR1, using 0.05 μl of the previous purified PCR1 per microlitre of PCR2. In this second PCR the remaining parts of the Illumina adapters were added to the library amplicon. The forward primer (5′ P5 Illumina adapter) was the same for all samples, while the reverse primer (3′ P7 Illumina adapter) differed by the barcode index (oligonucleotide sequences in Supplementary Table 1 ), to be subsequently pooled together and demultiplex them after deep sequencing (indexes used in each replicate of each sequencing run found in Supplementary Table 3 ). Eight cycles of PCR2s were run at 62 °C of annealing temperature and 10 s of extension time. All reactions from the same sample were pooled together and an aliquot was run on a 2% agarose gel to be quantified. All samples were purified using the QIAEX II Gel Extraction Kit. The purified amplicon library pools were subjected to Illumina 150 bp paired-end NextSeq sequencing at the CRG Genomics Core Facility.
Sequencing data processing
FastQ files from paired-end sequencing of all BindingPCA and AbundancePCA experiments were processed with DiMSum v1.2.9 (ref. 41 ) ( https://github.com/lehner-lab/DiMSum ) using default settings with minor adjustments. Supplementary Table 4 contains DiMSum fitness estimates and associated errors for all experiments. Experimental design files and command-line options required for running DiMSum on these datasets are available on GitHub ( https://github.com/lehner-lab/krasddpcams ). In all cases, adaptive minimum Input read count thresholds based on the corresponding number of nucleotide substitutions (‘fitnessMinInputCountAny’ option) were selected in order to minimize the fraction of reads per variant related to sequencing error-induced ‘variant flow’ from lower order mutants.
Variant counts associated with all samples (output from DiMSum stage 4) were further filtered using a custom script to retain only those variants with single amino acid substitutions including a G/T in the third codon position (encoded by NNK) or amino acid substitutions representing high confidence backgrounds. The latter were defined as single amino acid substitutions observed at least 200 times (in different double amino acid variants) in at least five (out of a total of seven) BindingPCA/AbundancePCA experiments. For double amino acid variants, we required one of the constituent single amino acid variants to be a high confidence background mutation. All read counts associated with remaining single or double amino acid variants (probably the result of PCR and sequencing errors) were discarded. Finally, fitness estimates and associated errors were then obtained from the resulting filtered variant counts with DiMSum (countPath option).
Thermodynamic model fitting with MoCHI
We used MoCHI v0.9 ( https://github.com/lehner-lab/MoCHI ) 22 to fit a global mechanistic model to all 21 ddPCA datasets (7 phenotypes × 3 blocks) simultaneously. The software is based on our previously described genotype–phenotype modelling approach 13 with additional functionality and improvements for ease of use and flexibility.
In brief, we model individual KRAS PPIs as an equilibrium between three states: unfolded and unbound (uu), folded and unbound (fu), and folded and bound (fb). We assume that the probability of the unfolded and bound state (ub) is negligible and free energies of folding and binding are additive—that is, the total binding and folding free energy changes of an arbitrary variant relative to the wild-type sequence is simply the sum over residue-specific energies corresponding to all constituent single amino acid substitutions. Furthermore, we assume binding energies are specific for each binding partner whereas folding energies are shared or intrinsic to KRAS—that is, unaffected by the identity, presence or expression of a given binding partner. We also assume that mutation effects on abundance level predominantly arise from folding free energy changes. However, protein abundance can be influenced by factors beyond folding, such as degradation or cellular processes, which may skew the free energy estimates.
We configured MoCHI parameters to specify a neural network architecture consisting of seven additive trait layers (free energies)—that is, one for each biophysical trait to be inferred (6 binding and 1 folding), as well as one linear transformation layer per experiment (3 AbundancePCA and 18 BindingPCA fitness). The specified nonlinear transformations ‘TwoStateFractionFolded’ and ‘ThreeStateFractionBound’ derived from the Boltzmann distribution function relate energies to proportions of folded and bound molecules respectively. The target (output) data to fit the neural network comprises fitness scores for wild-type, single and double amino acid substitution variants from all 21 ddPCA datasets.
A random 30% of double amino acid substitution variants was held out during model training, with 20% representing the validation data and 10% representing the test data. Validation data were used to evaluate training progress and optimize hyperparameters (batch size). Optimal hyperparameters were defined as those resulting in the smallest validation loss after 100 training epochs. Test data were used to assess final model performance.
MoCHI optimizes the parameters θ of the neural network using stochastic gradient descent on a loss function L [ θ ] based on a weighted and regularized form of mean absolute error:
where y n and σ n are the observed fitness score and associated standard error respectively for variant n , \({\widehat{y}}_{n}\) is the predicted fitness score, N is the batch size and λ 2 is the L 2 regularization penalty. In order to penalize very large free energy changes (typically associated with extreme fitness scores) we set λ 2 to 10 −6 representing light regularization. The mean absolute error is weighted by the inverse of the fitness error \(({\sigma }_{n}^{-1})\) in order to downweight the contribution of less confidently estimated fitness scores to the loss. Furthermore, in order to capture the uncertainty in ddPCA fitness estimates, the training data were replaced with a random sample from the fitness error distribution of each variant. The validation and test data were left unaltered.
Models were trained with default settings—that is, for a maximum of 1,000 epochs using the Adam optimization algorithm with an initial learning rate of 0.05. MoCHI reduces the learning rate exponentially ( γ = 0.98) if the validation loss has not improved in the most recent ten epochs compared to the preceding ten epochs. In addition, MoCHI stops model training early if the wild-type free energy terms over the most recent ten epochs have stabilized (standard deviation ≤10 −3 ).
Free energies are calculated directly from model parameters as follows: ∆ G b = θbRT and ∆ G f = θfRT , where T = 303 K and R = 0.001987 kcal K −1 mol −1 . We estimated the confidence intervals of model-inferred free energies using a Monte Carlo simulation approach. The variability of inferred free energy changes was calculated between ten separate models fit using data from (1) independent random training–validation–test splits; and (2) independent random samples of fitness estimates from their underlying error distributions. Confident inferred free energy changes are defined as those with Monte Carlo simulation derived 95% confidence intervals of less than 1 kcal mol −1 . Supplementary Table 5 contains inferred binding and folding free energy changes of mutations for all binding partners.
Recombinant protein sample preparation
KRAS residues 1–169 fused to an N-terminal His 6 tag and a TEV protease cleavage site was cloned into a pCoofy31 vector, and variants were generated by using the Q5 site-directed mutagenesis kit (New England Biolabs). Vectors were transformed into E. coli BL21 competent cells (NEB), and single colonies were picked to grow overnight pre-cultures to saturation in Luria-Bertani broth (LB) containing 33 μg ml −1 kanamycin. Ten millilitres of the pre-cultures were used to inoculate antibiotic-supplemented 1 l LB cultures, which were grown at 24 °C to OD 600 ≈ 0.4, then at 18 °C to OD 600 ≈ 0.6. Protein expression was induced with 0.5 mM isopropyl β- d -1-thiogalactopyranoside (IPTG), and induced cultures were grown at 18 °C overnight. Cells were collected by centrifugation (15 min, 3,000g, 4 °C), resuspended in KRAS lysis buffer (20 mM Tris, 500 mM NaCl, 25 mM imidazole, 5 mM MgCl2, 2 mM β-mercaptoethanol, pH 8) supplemented with one tablet of Pierce protease inhibitor tablets, 0.5 mg ml −1 PMSF (both from ThermoFisher), 0.1 mg ml −1 bovine pancreas DNAse I and 1.5 mg ml −1 chicken egg white lysozyme (both from Sigma Aldrich), and lysed in an Emulsiflex-C5 homogenizer (Avestin) at a maximum pressure of 1,500 psi. Cell debris was removed by ultracentrifugation (20 min, 40,000 g , 4 °C) and the cleared lysate was loaded on a His-Trap Fast Flow column mounted on an Äkta Pure chromatography system (both from Cytiva). Column-bound recombinant KRAS variants were washed with KRAS lysis buffer containing 1 M KCl and eluted over a 15-column-volume gradient with lysis buffer containing 0.5 M imidazole. Collected 0.25 ml fractions were analysed by SDS–PAGE, pooled based on purity and concentrated using Amicon 10 kDa MWCO centrifugal filters (Merck Millipore).
Nucleotide exchange to load the non-hydrolysable GTP analogue guanosine 5′-[β,γ-imido]triphosphate (GppNHp, Sigma Aldrich) was achieved by adapting a previously detailed method 42 . In brief, concentrated KRAS variants were diluted to a concentration of 1.8 mg ml −1 and a final volume of 2.5 ml in GppNHp loading buffer (50 mM Tris, 200 mM (NH 4 ) 2 SO 4 , 2 mM β-mercaptoethanol, pH 8) containing 3 mg of GppNHp. After 1 h incubation at 4 °C in a rotating wheel, samples were passed through a PD-10 column and eluted with 3.5 ml of GppNHp loading buffer. 30 units (6 μl) of QuickCIP (NEB) were added along with 2 mg of GppNHp, and samples were incubated for an additional 1 h on a rotating wheel at 4 °C. Subsequently, MgCl 2 was added to a concentration of 30 mM.
Both GDP and GppNHp-loaded samples were concentrated down to 0.5 ml and injected to a Superdex 75 10/300 GL column (Cytiva) equilibrated with SPR buffer (20 mM HEPES, 150 mM NaCl, 1 mM TCEP, pH 7.4) and mounted on an Äkta Pure system for size-exclusion chromatography. 0.5 ml fraction purity was assessed by SDS–PAGE, and fractions with ≥95% purity were flash-frozen in liquid nitrogen and stored at −80 °C until required for SPR measurement.
RAF1 residues 56–131, as well as DARPin K55 residues 1–156, fused to an N-terminal Twin-Strep tag and a 3 C HRV protease cleavage site were also cloned into pCoofy31. Inoculated cultures were grown at 37 °C to OD 600 ≈ 0.6, induced with 1 mM IPTG, and collected after 3 h growth at 37 °C. Cleared lysates in ligand lysis buffer (100 mM Tris, 150 mM NaCl, 1 mM EDTA, pH 8) were loaded on a StrepTrap XT prepacked chromatography column mounted on an Äkta Pure system (Cytiva). Bound protein was step-eluted with ligand lysis buffer containing 50 mM biotin, fractions were pooled based on SDS–PAGE-assessed purity and concentrated using 10 kDa MWCO centrifugal filters. Size-exclusion chromatography in SPR buffer and storage were performed in an analogous manner as described above for KRAS.
Surface plasmon resonance
Samples were thawed on ice, centrifuged at 13,000 g for 10 min, transferred to a new tube, and quantified using a NanoDrop One (ThermoFisher). Binding kinetics and affinity of KRAS variants for RAS or K55 were evaluated by surface plasmon resonance on a BIAcore T200 instrument (Cytiva) with SPR running buffer (10 mM HEPES, 150 mM NaCl, 0.05% Tween 20, pH 7.2). The assay format involved a Series S CM5 chip functionalized with Streptactin (50 μg ml −1 ). In brief, amine coupling was used to create a Streptactin surface (Strep-Tactin XT) following instructions provided with the Twin-Strep-tag capture kit (IBA Lifesciences). Twin-Strep-tagged RAS or K55 protein constructs were captured on flow cell 4, leaving flow cell 3 as a subtractive reference. Capture levels of RAS or K55 were targeted between 50 and 100 resonance units, after which increasingly concentrated samples of KRAS variants were flowed over immobilized RAS or K55 (50 μl min −1 for 1 min) and allowed to dissociate up to 3 min. A concentration series of each KRAS variant ranging from 0.74 nM to 60 nM was used to analyse binding to RAS or K55. The capture surface was regenerated with a 60 s injection of 3 M guanidine hydrochloride (50 μl min −1 for 1 min). All sensograms were analysed using a 1:1 Langmuir binding model.
Reporting summary
Further information on research design is available in the Nature Portfolio Reporting Summary linked to this article.
Data availability
All DNA sequencing data have been deposited in the Sequence Read Archive (SRA) under BioProject PRJNA907205 . All fitness measurements and free energies are provided in Supplementary Tables 4 and 5 and released on MAVEdb (MAVEdb accession: urn:mavedb:00000115 ).
Code availability
Source code for fitting thermodynamic models (MoCHI) is available at https://github.com/lehner-lab/MoCHI . Source code for all downstream analyses and to reproduce all figures described here is available at https://github.com/lehner-lab/krasddpcams .
Amberger, J. S., Bocchini, C. A., Scott, A. F. & Hamosh, A. OMIM.org: leveraging knowledge across phenotype–gene relationships. Nucleic Acids Res. 47 , D1038–D1043 (2019).
Article CAS PubMed Google Scholar
Cook, J. H., Melloni, G. E. M., Gulhan, D. C., Park, P. J. & Haigis, K. M. The origins and genetic interactions of KRAS mutations are allele- and tissue-specific. Nat. Commun. 12 , 1808 (2021).
Article CAS PubMed PubMed Central ADS Google Scholar
Lu, S. et al. Ras conformational ensembles, allostery, and signaling. Chem. Rev. 116 , 6607–6665 (2016).
Parker, M. I., Meyer, J. E., Golemis, E. A. & Dunbrack, R. L. Delineating the RAS conformational landscape. Cancer Res. 82 , 2485–2498 (2022).
Article CAS PubMed PubMed Central Google Scholar
Hofmann, M. H., Gerlach, D., Misale, S., Petronczki, M. & Kraut, N. Expanding the reach of precision oncology by drugging all KRAS mutants. Cancer Discov. 12 , 924–937 (2022).
Pantsar, T. The current understanding of KRAS protein structure and dynamics. Comput. Struct. Biotechnol. J. 18 , 189–198 (2020).
Tsuchida, N., Ryder, T. & Ohtsubo, E. Nucleotide sequence of the oncogene encoding the p21 transforming protein of Kirsten murine sarcoma virus. Science 217 , 937–939 (1982).
Article CAS PubMed ADS Google Scholar
Ostrem, J. M., Peters, U., Sos, M. L., Wells, J. A. & Shokat, K. M. K-Ras(G12C) inhibitors allosterically control GTP affinity and effector interactions. Nature 503 , 548–551 (2013).
Skoulidis, F. et al. Sotorasib for lung cancers with p.G12C mutation. N. Engl. J. Med. 384 , 2371–2381 (2021).
Lanman, B. A. et al. Discovery of a covalent inhibitor of KRAS (AMG 510) for the treatment of solid tumors. J. Med. Chem. 63 , 52–65 (2020).
Changeux, J.-P. The concept of allosteric modulation: an overview. Drug Discov. Today Technol. 10 , e223–e228 (2013).
Article PubMed ADS Google Scholar
Fenton, A. W. Allostery: an illustrated definition for the ‘second secret of life’. Trends Biochem. Sci. 33 , 420–425 (2008).
Faure, A. J. et al. Mapping the energetic and allosteric landscapes of protein binding domains. Nature 604 , 175–183 (2022).
Wrenbeck, E. E. et al. Plasmid-based one-pot saturation mutagenesis. Nat. Methods 13 , 928–930 (2016).
Li, X. & Lehner, B. Biophysical ambiguities prevent accurate genetic prediction. Nat. Commun. 11 , 4923 (2020).
Tarassov, K. et al. An in vivo map of the yeast protein interactome. Science 320 , 1465–1470 (2008).
Freschi, L., Torres-Quiroz, F., Dubé, A. K. & Landry, C. R. qPCA: a scalable assay to measure the perturbation of protein-protein interactions in living cells. Mol. Biosyst. 9 , 36–43 (2013).
Bandaru, P. et al. Deconstruction of the Ras switching cycle through saturation mutagenesis. eLife 6 , e27810 (2017).
Article PubMed PubMed Central Google Scholar
Hidalgo, F. et al. A saturation-mutagenesis analysis of the interplay between stability and activation in Ras. eLife 11 , e76595 (2022).
Otwinowski, J. Biophysical inference of epistasis and the effects of mutations on protein stability and function. Mol. Biol. Evol. 35 , 2345–2354 (2018).
Levy, E. D., Kowarzyk, J. & Michnick, S. W. High-resolution mapping of protein concentration reveals principles of proteome architecture and adaptation. Cell Rep. 7 , 1333–1340 (2014).
Faure, A. J. & Lehner, B. MoCHI: neural networks to fit interpretable models and quantify energies, energetic couplings, epistasis and allostery from deep mutational scanning data. bioRxiv https://doi.org/10.1101/2024.01.21.575681 (2024).
Grant, B. J. et al. Novel allosteric sites on Ras for lead generation. PLoS ONE 6 , e25711 (2011).
Ostrem, J. M. L. & Shokat, K. M. Direct small-molecule inhibitors of KRAS: from structural insights to mechanism-based design. Nat. Rev. Drug Discov. 15 , 771–785 (2016).
Kessler, D. et al. Drugging an undruggable pocket on KRAS. Proc. Natl Acad. Sci. USA 116 , 15823–15829 (2019).
Canon, J. et al. The clinical KRAS(G12C) inhibitor AMG 510 drives anti-tumour immunity. Nature 575 , 217–223 (2019).
Guillard, S. et al. Structural and functional characterization of a DARPin which inhibits Ras nucleotide exchange. Nat. Commun. 8 , 16111 (2017).
Tran, T. H. et al. KRAS interaction with RAF1 RAS-binding domain and cysteine-rich domain provides insights into RAS-mediated RAF activation. Nat. Commun. 12 , 1176 (2021).
Pacold, M. E. et al. Crystal structure and functional analysis of Ras binding to its effector phosphoinositide 3-kinase γ. Cell 103 , 931–943 (2000).
Huang, L., Hofer, F., Martin, G. S. & Kim, S. H. Structural basis for the interaction of Ras with RalGDS. Nat. Struct. Biol. 5 , 422–426 (1998).
Margarit, S. M. et al. Structural evidence for feedback activation by Ras.GTP of the Ras-specific nucleotide exchange factor SOS. Cell 112 , 685–695 (2003).
Nikam, R., Kulandaisamy, A., Harini, K., Sharma, D. & Gromiha, M. M. ProThermDB: thermodynamic database for proteins and mutants revisited after 15 years. Nucleic Acids Res. 49 , D420–D424 (2021).
Zhong, Q. et al. Edgetic perturbation models of human inherited disorders. Mol. Syst. Biol. 5 , 321 (2009).
Buday, L. & Downward, J. Many faces of Ras activation. Biochim. Biophys. Acta 1786 , 178–187 (2008).
CAS PubMed Google Scholar
Koscielny, G. et al. Open Targets: a platform for therapeutic target identification and validation. Nucleic Acids Res. 45 , D985–D994 (2017).
Perica, T. et al. Systems-level effects of allosteric perturbations to a model molecular switch. Nature 599 , 152–157 (2021).
Mathy, C. J. P. et al. A complete allosteric map of a GTPase switch in its native network. Cell Syst. 14 , 237–246.e7 (2023).
Tack, D. S. et al. The genotype-phenotype landscape of an allosteric protein. Mol. Syst. Biol. 17 , e10847 (2021).
Leander, M., Yuan, Y., Meger, A., Cui, Q. & Raman, S. Functional plasticity and evolutionary adaptation of allosteric regulation. Proc. Natl Acad. Sci. USA 117 , 25445–25454 (2020).
Kiel, C., Serrano, L. & Herrmann, C. A detailed thermodynamic analysis of Ras/effector complex interfaces. J. Mol. Biol. 340 , 1039–1058 (2004).
Faure, A. J., Schmiedel, J. M., Baeza-Centurion, P. & Lehner, B. DiMSum: an error model and pipeline for analyzing deep mutational scanning data and diagnosing common experimental pathologies. Genome Biol. 21 , 207 (2020).
Gentile, D. R. et al. Ras binder induces a modified switch-II pocket in GTP and GDP states. Cell Chem. Biol. 24 , 1455–1466.e14 (2017).
Download references
Acknowledgements
This work was funded by European Research Council (ERC) Advanced grant (883742), the Spanish Ministry of Science and Innovation (LCF/PR/HR21/52410004, EMBL Partnership, Severo Ochoa Centre of Excellence), the Bettencourt Schueller Foundation, the AXA Research Fund, Agencia de Gestio d’Ajuts Universitaris i de Recerca (AGAUR, 2017 SGR 1322), and the CERCA Program/Generalitat de Catalunya. C.W. was funded by an EMBO long-term fellowship (ALTF 881-2020). A.J.F. was funded by a Ramón y Cajal fellowship (RYC2021-033375-I) financed by the Spanish Ministry of Science and Innovation (MCIN/AEI/10.13039/501100011033) and the European Union (NextGenerationEU/PRTR). We thank all members of the Lehner laboratory for helpful discussions and suggestions. We thank the CRG Genomics Unit for sequencing and the Protein Technologies Unit for assistance with the SPR measurements.
Author information
Authors and affiliations.
Centre for Genomic Regulation (CRG), The Barcelona Institute of Science and Technology, Barcelona, Spain
Chenchun Weng, Andre J. Faure, Albert Escobedo & Ben Lehner
University Pompeu Fabra (UPF), Barcelona, Spain
Institució Catalana de Recerca i Estudis Avançats (ICREA), Barcelona, Spain
Wellcome Sanger Institute, Wellcome Genome Campus, Hinxton, UK
You can also search for this author in PubMed Google Scholar
Contributions
B.L. and C.W. conceived the project and designed the experiments. C.W. and A.E. performed the experiments. C.W. and A.J.F. performed the data analysis. B.L., C.W., A.J.F. and A.E. wrote the manuscript.
Corresponding author
Correspondence to Ben Lehner .
Ethics declarations
Competing interests.
A.J.F. and B.L. are founders, employees and shareholders of ALLOX.
Peer review
Peer review information.
Nature thanks the anonymous reviewer(s) for their contribution to the peer review of this work. Peer review reports are available.
Additional information
Publisher’s note Springer Nature remains neutral with regard to jurisdictional claims in published maps and institutional affiliations.
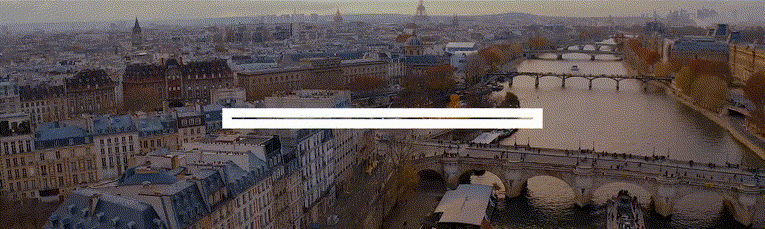
Extended data figures and tables
Extended data fig. 1 experimental reproducibility and thermodynamic model fitting..
a , b , Scatter plots showing the reproducibility of each block’s RAF1 BindingPCA ( a ) and AbundancePCA ( b ) fitness estimates from ddPCA. Pearson’s r indicated on the top right corner. c , Comparison of RAF1 BindingPCA fitness to previously reported KRAS-RAF1 binding E score 19 . Pearson’s r = 0.82. d , Comparison of individually measured growth rates to their corresponding fitness from deep sequencing for KRAS. The red line corresponds to a linear regression model. Pearson’s r is shown. e , Single mutation fitness density distributions. f , 2D density plots showing non-linear relationships (global epistasis) between observed AbundancePCA fitness and changes in free energy of folding. g , 2D density plots comparing model predictions and observations of AbundancePCA fitness for held out test data (comprising 10% of double aa substitution variants held out during model training). h , Same as ( g ) except the model was trained using data from replicates 1 and 2 and evaluated using data from replicate 3. i , Non-linear relationships (global epistasis) between observed BindingPCA fitness and both free energies of binding and folding. j , 2D density plots comparing model predictions and observations of BindingPCA fitness for held out test data. k , Same as ( j ) except the model was trained using data from replicates 1 and 2 and evaluated using data from replicate 3. The three columns in panels ( g - k ) indicate data corresponding to the three mutagenesis library blocks (block 1, left; block 2, middle; block 3, right).
Extended Data Fig. 2 Allosteric mutations in the KRAS beta sheet and surface pockets.
a , Heat maps of binding free energy changes of residues in the beta sheet. GTP indicates the location of GTP in the 3D structure. b , Number of allosteric mutations in each secondary structure element. *, odds ratio > 1, and two-sided Fisher’s Exact Test P < 0.05. c , Scatter plot showing the binding free energy changes of all mutations and the distance to the binding partner. Residues in beta sheet and GTP binding sites (minimal side chain heavy atom distance to GTP < 5 Å) are coloured as indicated. d , e , f , g , Scatter plot showing the binding free energy changes of all mutations and the distance to the binding partner. Residues in each pocket are coloured as indicated. h , Surface plasmon resonance (SPR) single-cycle kinetics (SCK) measurement of the in vitro binding of a KRAS major allosteric site variant, A59R, and a pocket 3 KRAS variant P110F, both in the active state (GppNHp-loaded), to the RAF1 RBD (raw data as colour scatter plot, 2 replicates per measurement, data fit as solid black line). Analogous measurements for WT KRAS in the active (GppNHp-loaded) and inactive (GDP-loaded) states are shown for reference.
Extended Data Fig. 3 Experimental reproducibility and thermodynamic model fitting for five additional interaction partners.
a , Scatter plots showing the reproducibility of each block’s BindingPCA fitness estimates from ddPCA. Pearson’s r indicated on the top right corner. b , Performance of models fit to ddPCA data. 2D density plots comparing model predictions and observations of BindingPCA fitness for held out test data (comprising 10% of double aa substitution variants held out during model training). c , Same as ( b ) except the model was trained using data from replicates 1 and 2 and evaluated using data from replicate 3.
Extended Data Fig. 4 Seven KRAS free energy landscapes.
a , Heat maps of folding and binding free energy changes. b , ROC curves for predicting binding interface residues (distance to binding partner <5 Å) using weighted mean absolute binding free energy changes (∆∆G) in red or using weighted mean absolute binding fitness in black. AUC = Area Under the Curve.
Extended Data Fig. 5 Binding interface specificity for all interactions.
Heat maps of binding free energy changes of all binding partners (RAF1, PIK3CG, RALGDS, SOS1, DARPin K27, DARPin K55) for binding interface residues. * indicates the binding interface residues of each binding partner.
Extended Data Fig. 6 Allosteric regulation for six binding partners.
a , Surface plasmon resonance (SPR) single-cycle kinetics (SCK) measurements of the in vitro binding of a KRAS major allosteric site variant, A59R, and a pocket 3 KRAS variant P110F, both in the active state (GppNHp-loaded) to the DARPin K55 (raw data as colour scatter plot, 2 replicates per measurement, data fit as solid black line). An analogous measurement for WT KRAS in the active state (GppNHp-loaded) is shown for reference. b , Enrichments are quantified for changes from each WT aa and for changes to each aa. Enrichments are also quantified for changes from and to amino acids with particular physicochemical properties: hydrophobic (A, V, I, L, M, F, Y, W) and charged (R, H, K, D, E). Results are shown for all residues outside the binding interface.
Extended Data Fig. 7 Binding energy and allosteric landscapes for all six binding partners.
Manhattan plots showing the binding free energy changes of all mutations coloured according to residue position and whether the free energy change is larger than the weighted mean of binding free energy changes in the binding-interfaces of all six proteins. Two-sided z test, FDR < 0.05.
Extended Data Fig. 8 KRAS-RAF1RBD BindingPCA with RASGAP co-expression.
a , Heat maps of KRAS-RAF1 BindingPCA for single aa substitutions with RASGAP co-expression. White, missing values; -, WT aa; *, STOP codon. b , 2D density plots showing the reproducibility of binding fitness of block1 estimates from bindingPCA with RASGAP co-expression. Pearson’s r indicated on the top right corner. c , Single mutation growth rates density distributions. Cancer driver mutation (G12C, G12D, G12V, G13D, Q61H, Q61L) growth rates are indicated with dashed lines. WT growth rates are indicated with solid lines. KRAS-RAF1RBD BindingPCA growth rates are coloured by blue, co-expression RASGAP KRAS-RAF1RBD BindingPCA growth rates are coloured by red. d - i , Comparisons of binding fitness of single aa substitutions in WT background or G12C (left), G12D (middle), G12V (right) background for KRAS-RAF1RBD BindingPCA in the absence (top) and presence (bottom) of human GAP co-expression. Points are coloured as in Fig. 3a according to residue position and whether the corresponding binding ∆∆G is significantly greater than the weighted mean absolute binding ∆∆G of RAF1RBD BindingPCA of all mutations in the RAF1 binding interface (two-sided z -test, FDR = 0.05). Pearson’s r for all mutations are indicated in black, allosteric mutations are indicated in orange.
Extended Data Fig. 9 KRAS-full RAF1 BindingPCA.
a , Heat maps of fitness effects of single aa substitutions for KRAS-full RAF1 from BindingPCA. White, missing values; -, WT aa; *, STOP codon. b , 2D density plots showing the reproducibility of binding fitness of block1 for KRAS-full RAF1 from BindingPCA. Pearson’s r indicated on the top right corner. c , Comparisons of binding fitness of single aa substitutions for KRAS-RAF1RBD and for KRAS-full RAF1 from BindingPCA. Points are coloured as in Fig. 3a according to residue position and whether the corresponding binding ∆∆G is significantly greater than the weighted mean absolute binding ∆∆G of RAF1RBD BindingPCA of all mutations in the RAF1 binding interface (two-sided z-test, FDR = 0.05). Pearson’s r is shown. d , Comparisons of model-inferred binding free energy changes of single aa substitutions for KRAS-RAF1RBD and for KRAS-full RAF1. Points are coloured as in ( c ). Pearson’s r for all mutations is indicated in black, allosteric mutations is indicated in orange.
Supplementary information
Reporting summary, supplementary table 1.
Primers used in this study.
Supplementary Table 2
Degenerate NNK oligonucleotides used for the KRAS nicking mutagenesis libraries.
Supplementary Table 3
Experimental details and numbers of the mutagenesis libraries in this study.
Supplementary Table 4
Fitness estimates for KRAS abundance and binding to six binding partners.
Supplementary Table 5
Inferred folding and binding free energy changes for KRAS.
Supplementary Video 1 3D structure of KRAS the weighted mean folding free energy changes.
3D structure of KRAS in which residue atoms are coloured by the weighted mean folding free energy change. (Video of Fig. 1i).
Supplementary Video 2 3D structure of KRAS bound to RAF1.
3D structure of KRAS bound to RAF1 in which residue atoms are coloured by the position-wise weighted mean absolute change in the free energy of binding to RAF1. (Video of Fig. 2c).
Supplementary Video 3 3D structure of allosteric sites of KRAS bound to RAF1.
3D structure of KRAS bound to RAF1 (grey) with binding interface (red), GTP pocket residue atoms (blue) and major allosteric site residue atoms (orange) of KRAS. (Video of Fig. 3c).
Supplementary Video 4 3D structure of maximum KRAS binding free energy change to RAF1.
3D structure of KRAS bound to RAF1 (grey) with residues coloured by maximum binding ∆∆ G . (Video of Fig. 3d).
Supplementary Video 5 3D structure of KRAS pockets.
3D structure alignment (PDB:6OIM, 6VJJ) of KRAS bound to GDP (blue), sotorasib (yellow) and RAF1 (grey) with KRAS surface coloured according to previously described pockets in KRAS. (Video of Fig. 3f).
Peer Review File
Rights and permissions.
Open Access This article is licensed under a Creative Commons Attribution 4.0 International License, which permits use, sharing, adaptation, distribution and reproduction in any medium or format, as long as you give appropriate credit to the original author(s) and the source, provide a link to the Creative Commons licence, and indicate if changes were made. The images or other third party material in this article are included in the article’s Creative Commons licence, unless indicated otherwise in a credit line to the material. If material is not included in the article’s Creative Commons licence and your intended use is not permitted by statutory regulation or exceeds the permitted use, you will need to obtain permission directly from the copyright holder. To view a copy of this licence, visit http://creativecommons.org/licenses/by/4.0/ .
Reprints and permissions
About this article
Cite this article.
Weng, C., Faure, A.J., Escobedo, A. et al. The energetic and allosteric landscape for KRAS inhibition. Nature 626 , 643–652 (2024). https://doi.org/10.1038/s41586-023-06954-0
Download citation
Received : 06 December 2022
Accepted : 07 December 2023
Published : 18 December 2023
Issue Date : 15 February 2024
DOI : https://doi.org/10.1038/s41586-023-06954-0
Share this article
Anyone you share the following link with will be able to read this content:
Sorry, a shareable link is not currently available for this article.
Provided by the Springer Nature SharedIt content-sharing initiative
This article is cited by
Mutational scanning pinpoints distinct binding sites of key atgl regulators in lipolysis.
- Johanna M. Kohlmayr
- Gernot F. Grabner
- Ulrich Stelzl
Nature Communications (2024)
By submitting a comment you agree to abide by our Terms and Community Guidelines . If you find something abusive or that does not comply with our terms or guidelines please flag it as inappropriate.
Quick links
- Explore articles by subject
- Guide to authors
- Editorial policies
Sign up for the Nature Briefing: Translational Research newsletter — top stories in biotechnology, drug discovery and pharma.

Tour de kras
- Pohled do historie
- Fotogalerie 2018 - 2019
- Fotogalerie 2016 - 2017
- Fotogalerie 2014 - 2015
- Fotogalerie 2012 - 2013
- Fotogalerie 2011
- Fotogalerie 2006 - 2010
- Fotogalerie 2001 - 2005
- Fotogalerie 1992 - 2000
Spanilá jízda?
Stále v jednom kole!
Pitný režim?
Další ročník se uskuteční 23.7.-24.7.2022
Jedná se o recesní akci, která spočívá v jízdě na kolech po Moravském krasu a restauračních zařízeních. V roce 1992 se uskutečnila první spanilá jízda cyklistů a velocipedistů . Největší počet účastníků byl v roce 2011. Tento 20-tý ročník podpořilo svou účastí rovných 100 "závodníků".
Náhodně vybrané fotografie
Moscow Metro Tour
- Page active

Description
Moscow metro private tours.
- 2-hour tour $87: 10 Must-See Moscow Metro stations with hotel pick-up and drop-off
- 3-hour tour $137: 20 Must-See Moscow Metro stations with Russian lunch in beautifully-decorated Metro Diner + hotel pick-up and drop off.
- Metro pass is included in the price of both tours.
Highlight of Metro Tour
- Visit 10 must-see stations of Moscow metro on 2-hr tour and 20 Metro stations on 3-hr tour, including grand Komsomolskaya station with its distinctive Baroque décor, aristocratic Mayakovskaya station with Soviet mosaics, legendary Revolution Square station with 72 bronze sculptures and more!
- Explore Museum of Moscow Metro and learn a ton of technical and historical facts;
- Listen to the secrets about the Metro-2, a secret line supposedly used by the government and KGB;
- Experience a selection of most striking features of Moscow Metro hidden from most tourists and even locals;
- Discover the underground treasure of Russian Soviet past – from mosaics to bronzes, paintings, marble arches, stained glass and even paleontological elements;
- Learn fun stories and myths about Coffee Ring, Zodiac signs of Moscow Metro and more;
- Admire Soviet-era architecture of pre- and post- World War II perious;
- Enjoy panoramic views of Sparrow Hills from Luzhniki Metro Bridge – MetroMost, the only station of Moscow Metro located over water and the highest station above ground level;
- If lucky, catch a unique «Aquarelle Train» – a wheeled picture gallery, brightly painted with images of peony, chrysanthemums, daisies, sunflowers and each car unit is unique;
- Become an expert at navigating the legendary Moscow Metro system;
- Have fun time with a very friendly local;
- + Atmospheric Metro lunch in Moscow’s the only Metro Diner (included in a 3-hr tour)
Hotel Pick-up
Metro stations:.
Komsomolskaya
Novoslobodskaya
Prospekt Mira
Belorusskaya
Mayakovskaya
Novokuznetskaya
Revolution Square
Sparrow Hills
+ for 3-hour tour
Victory Park
Slavic Boulevard
Vystavochnaya
Dostoevskaya
Elektrozavodskaya
Partizanskaya
Museum of Moscow Metro
- Drop-off at your hotel, Novodevichy Convent, Sparrow Hills or any place you wish
- + Russian lunch in Metro Diner with artistic metro-style interior for 3-hour tour
Fun facts from our Moscow Metro Tours:
From the very first days of its existence, the Moscow Metro was the object of civil defense, used as a bomb shelter, and designed as a defense for a possible attack on the Soviet Union.
At a depth of 50 to 120 meters lies the second, the coded system of Metro-2 of Moscow subway, which is equipped with everything you need, from food storage to the nuclear button.
According to some sources, the total length of Metro-2 reaches over 150 kilometers.
The Museum was opened on Sportivnaya metro station on November 6, 1967. It features the most interesting models of trains and stations.
Coffee Ring
The first scheme of Moscow Metro looked like a bunch of separate lines. Listen to a myth about Joseph Stalin and the main brown line of Moscow Metro.
Zodiac Metro
According to some astrologers, each of the 12 stops of the Moscow Ring Line corresponds to a particular sign of the zodiac and divides the city into astrological sector.
Astrologers believe that being in a particular zadiac sector of Moscow for a long time, you attract certain energy and events into your life.
Paleontological finds
Red marble walls of some of the Metro stations hide in themselves petrified inhabitants of ancient seas. Try and find some!
- Every day each car in Moscow metro passes more than 600 km, which is the distance from Moscow to St. Petersburg.
- Moscow subway system is the 5th in the intensity of use (after the subways of Beijing, Tokyo, Seoul and Shanghai).
- The interval in the movement of trains in rush hour is 90 seconds .
What you get:
- + A friend in Moscow.
- + Private & customized Moscow tour.
- + An exciting pastime, not just boring history lessons.
- + An authentic experience of local life.
- + Flexibility during the walking tour: changes can be made at any time to suit individual preferences.
- + Amazing deals for breakfast, lunch, and dinner in the very best cafes & restaurants. Discounts on weekdays (Mon-Fri).
- + A photo session amongst spectacular Moscow scenery that can be treasured for a lifetime.
- + Good value for souvenirs, taxis, and hotels.
- + Expert advice on what to do, where to go, and how to make the most of your time in Moscow.
Write your review
Tour de Romandie past winners
Champions from 1947 to 2023
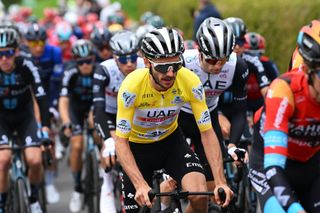
Thank you for reading 5 articles in the past 30 days*
Join now for unlimited access
Enjoy your first month for just £1 / $1 / €1
*Read any 5 articles for free in each 30-day period, this automatically resets
After your trial you will be billed £4.99 $7.99 €5.99 per month, cancel anytime. Or sign up for one year for just £49 $79 €59

Try your first month for just £1 / $1 / €1
Get The Leadout Newsletter
The latest race content, interviews, features, reviews and expert buying guides, direct to your inbox!
Cyclingnews is the world's leader in English-language coverage of professional cycling. Started in 1995 by University of Newcastle professor Bill Mitchell, the site was one of the first to provide breaking news and results over the internet in English. The site was purchased by Knapp Communications in 1999, and owner Gerard Knapp built it into the definitive voice of pro cycling. Since then, major publishing house Future PLC has owned the site and expanded it to include top features, news, results, photos and tech reporting. The site continues to be the most comprehensive and authoritative English voice in professional cycling.
Amstel Gold Race past winners 2024
La Flèche Wallonne Femmes winners
Ashlin Barry pursues family racing tradition, 2028 Olympics already a goal
Most popular.
By Cyclingnews February 22, 2024
By Jackie Tyson February 21, 2024
By Cycling News February 21, 2024
By Cyclingnews February 21, 2024
By Cycling News February 18, 2024
By Cycling News February 04, 2024
By Cycling News February 01, 2024
Latest on Cyclingnews
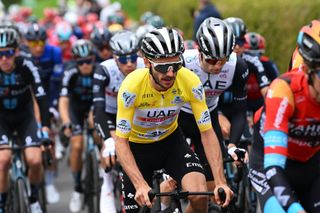
Julian Alaphilippe says he raced with fractured knee through spring campaign
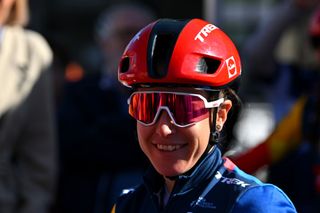
An Ardennes horizon and the easing Olympic obsession – Amanda Spratt
- EUR (€)
- GBP (£)
- Contact us

- Currency :
- US Dollar Euro Pound Sterling Russian Ruble Australian Dollar Canadian Dollar Swiss Franc Danish Krone Japanese Yen Norwegian Krone Swedish Krona
- Toll Free 0800 011 2023
- US and Canada United Kingdom Australia Brazil Netherlands Russia Sweden
- Tours by Region Moscow Saint Petersburg Golden Ring Lake Baikal Murmansk Siberia & Far East Sochi & Southern Russia Amsterdam Berlin Copenhagen Gdansk Georgia Helsinki Kaliningrad Kamchatka Karelia Kazan Klaipeda Nizhny Novgorod Northern Europe Oslo Perm Riga Ryazan Stockholm Tallinn Ulan-Ude Veliky Novgorod Vilnius Vladivostok Volgograd Yakutia Yekaterinburg
- Why Choose our Day Tours
- One Day Tours Two Days Tours Three Day Tours
- About Russian tours Private vs Group Tours
- Tours by Region Moscow & St.Peterburg Moscow Saint Petersburg Golden Ring Lake Baikal Trans-Siberian Siberia & Far East Altai Kaliningrad Kamchatka Karelia Kazan Perm Veliky Novgorod Yakutia Yekaterinburg
- Tour Types Small Group Tours Private Tour Theme Tour
- Moscow events St. Petersburg events Events archive
- Events by type Ballet Opera Concert Show All types
- Top theaters Bolshoi theatre - Historic Stage Bolshoi theatre - Small Stage Mariinsky Theater Mariinsky Theatre - Mariinsky II Mikhailovsky Theater
- Direction Moscow - St.Petersburg St. Petersburg - Moscow
- Ships MS Rostropovich MS Volga Dream MS Anton Chekhov MS General Lavrinenkov MS Georgy Chicherin MS Ivan Bunin MS Konstantin Fedin MS Konstantin Korotkov MS Kronshtadt MS Maxim Gorky MS Nikolay Chernyshevsky MS Nizhny Novgorod MS Rachmaninoff MS River Victoria MS Scenic Tsar MS Tikhy Don (MS Alexander Borodin) MS Vasiliy Kandinsky MS Zosima Shashkov Mustai Karim
- Russian Visa Invitation
- Moscow The Kremlin Red Square Golden Ring Tverskaya Street Grand Kremlin Palace (The Kremlin) Christ the Savior Novodevichiy Convent Moscow Subway The Bolshoi Theater Armoury Chamber (The Kremlin) St. Basil's Cathedral The Pushkin Museum of Fine Arts Yury Gagarin Cosmonauts training center
- Saint Petersburg Hermitage Museum Peterhof Catherine's Palace (Tsarskoe Selo) Pavlovsk St. Isaac Cathedral Peter and Paul Fortress Nevsky Prospect Yusupov Palace Savior on Blood Amber room Bronze Horseman (Senate square) Hermitage Theatre Kazansky Cathedral Kronstadt Palace Square Spit of the Vasilievsky Island
- 1/7th of the World Volga River Siberia Baikal Kazan Sochi Smolensk Nizhny Novgorod Kaliningrad Ulyanovsk Verkhoturova Island
- uVisitRussia Why travel with UVisitRussia Customers' Testimonials Contact details Cancellations & Changes
- Russian Travel News
- Russian travel advice Advices while packing Advices while travelling
Tour of famous Moscow Metro. Explore the Underground World! (2 hours)
- Add to wishlist
On this tour you take in some of Moscow's most important and highly decorated stations. Carrying up to 7 million passengers a day and covering almost the entire city, the Moscow Metro is one of the most extensive mass transit systems in the world. It's famous for the fine examples of social-realism which decorate many of its stations.
Visit some of the most important stations and get the chance to admire spectacular baroque-style ceilings, marble statues, busts of Communist heroes, stained glass windows, and ceiling mosaics depicting the bright Soviet future. Visit the most remarkable stations like Komsomolskaya, Kurskaya, Kievskaya and others, with the experienced guide who will bring you a full insight into their fascinating history.
- Tour of Moscow's Metro system visiting beautifully decorated key stations on the network.
- System that carries more than 7 million passengers per day
- Views of the most opulently designed tunnels & platforms
- Significance to the country—known as the “People's Palace”
- History & stories relayed by an expert local guide
If you wonder why the Moscow metro is considered one the most beautiful in the world, this tour is made for you!
Important info
Ask a travel expert.
- Professional English-speaking guide assistance. Other languages upon request (additional charge may apply)
- Hotel pick-up and drop-off
- Personal expenses
- Gratuities (optional)
- Food and drinks
- Confirmation will be received at time of booking
- Children must be accompanied by an adult
Departure and return point: nearest metro station to your central Moscow hotel
Departure time: flexible
Sights included in program
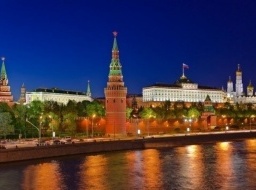
Thanks ! We will reply you in less than 24 hours (usually within 1-2 hours) .
Our flexible payment options allow you to pay 20% of a deposit first and the remaining 80% in 14 days prior to your tour date - payment can be done both online or over the phone. This gives you the peace of mind knowing your spaces are booked on the tours and that you do not miss out on making the most of your time in Russia.
Also you get the best, top-rated and most experienced and knowledgeable hand-picked tour guide appointed on a priority basis. In our experience, exceptional travel experiences are almost always delivered by exceptional people. With that in mind, we utilize a comprehensive approach to select and employ the best tour guides only. Multilingual and well travelled, each possesses deep insight into the diverse attractions and cultural patterns throughout the region. With us guides undergo a rigorous selection process, achieving outstanding knowledge of local culture and language. Rest assured that the best tour guides only will be working on the tours to give you excellent opportunity to explore the best of the sights during both short and long-term stay in Russia.
Once you complete your reservation, we will send you a booking confirmation email. As the day of the tour approaches, our logistics team will provide you with all relevant information for your tour.
Speak to an expert Call us now
+1 (888) 845-8877
- Shore excursions
- The Best of Two Capitals Private
- The Best of Two Capitals Small Group
- The Best of Two Capitals Gold
- Russia's Ancient Kingdoms
- Cradle of Russia
- Explore Moscow
- Explore St. Petersburg
- Read Customer Testimonials
- Advantages of Traveling with Us
US office 3422 Old Capitol Trail Suite 1252 , Wilmington DE, 19808 USA. US toll-free: 1-888-845-8877 Russian office Ligovsky pr. 57, Office 19, 191040, St. Petersburg, Russia
tel: +7-812-309-5339
© 2001 – 2024 by Northern Crown, Ltd. uVisitRussia and uVisitRussia.com are registered trademarks . Terms & Conditions Privacy Policy
Sign in with your social account
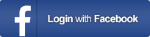
Sign in to our website using your Facebook or Google+ account.
Why Do You Need Our Travel Expert
Contact our experts, and they will help you to plan your best trip to Russia, with attention to every detail!
Our Experts have been in the travel industry for many years, guarantee to offer first class customer service, excellent value for money and unbiased advice. They are standing by to find and build your dream holiday to one of the world's most fascinating destinations - Russia. Your personal Travel Expert will guide you through each stage of the travel process, from choosing a program that fits you best to support during your trip.
Just tell us your e-mail, and we'll take care of everything!
Leave your phone number
Your tour request has been received. Thank you ! We have sent you the confirmation message to [email]
Please make sure that you receive this message (sometimes e-mail messages may go to the spam/junk mail). If you did not get this message, it means you will not get message with the tours' selection as well. If you use a Yahoo!, Gmail, AOL or Hotmail, we recommend to add [email protected] to your address book.
We recommend to leave your phone number. If we will not heard back that you received the e-mail with the tours' selection, we will contact you by phone. And you will not miss the best tour for you.
Thank you ! Your request for Travel Expert assistance has been sent. We will e-mail you within 1 hour.
Sorry, some changes needed
There was a problem with your request.
The Masters 2024: Guide to the DP World Tour members in action
A host of current DP World Tour members are teeing it up at the 2024 Masters Tournament , including four debutants while others are chasing further Major Championship honours.
Here’s our guide, including a synopsis of their form so far this year, to the 21 players with status on golf’s Global Tour who are in action at August National Golf Club. We've listed them in alphabetical order.
Ludvig Åberg
- Birthplace: Eslov, Sweden
- Turned Pro: 2023
- Official World Golf Ranking: 9
- Best Masters finish: N/A
After becoming the first player in history to compete in a Ryder Cup before ever playing a Major last September, Ludvig Åberg is making his Major Championship debut at the 88th Masters. Prior to turning professional in June 2023, the Swede was the top ranked amateur on the World Golf Amateur Rankings and made history as the first player to earn direct access to the PGA TOUR via collegiate merit, finishing first on the 2023 PGA TOUR University Ranking while at Texas Tech. He secured his first win as a professional at the Omega European Masters in September on the DP World Tour. He was also part of the winning European Ryder Cup team in Italy in October and earned his first PGA TOUR win at the RSM Classic in November. Finished runner-up in the AT&T Pebble Beach Pro-Am in February and eighth in his debut at The Players Championship in March, rising inside the top 10 in the Official World Golf Ranking for the first time.
Matt Fitzpatrick
- Birthplace: Sheffield, England
- Turned Pro: 2014
- Official World Golf Ranking: 10
- Best Masters finish: T7 (2016)
The Englishman, who is making his 10th Masters appearance, has finished inside the top 15 in each of the past two years at Augusta National. Like Åberg, he won two global titles in 2023, the first in Hilton Head Island on the PGA TOUR, before winning the Alfred Dunhill Championship at St Andrews in October. He too was part of the winning European Ryder Cup team in Italy. Won the U.S. Open in 2022 to claim his first Major Championship at The Country Club in Brookline, Massachusetts, the same course where he won the 2013 U.S. Amateur. Has top-10 finishes in his last two starts worldwide, The Players Championship and most recently at the Valero Texas Open.
Tommy Fleetwood
- Birthplace: Southport, England
- Turned Pro: 2010
- Official World Golf Ranking: 13
- Best Masters finish: T7 (2022)
Fleetwood has finished inside the top 20 in three of the past six years at Augusta National, with this being his eighth Masters appearance. In 2023, he finished runner-up in both the Canadian Open and the season-ending DP World Tour Championship in Dubai. He recorded top 10s in both the U.S. Open and The Open, along with the clinching point for Team Europe at the 2023 Ryder Cup. The Englishman made a terrific start to 2024, winning the Dubai Invitational in January for his seventh win on the DP World Tour. Two top 10s have since followed on the PGA TOUR, including last week in San Antonio.
- Birthplace: Auckland, New Zealand
- Turned Pro: 2011
- Official World Golf Ranking: 54
- Best Masters finish: T26 (2023)
The New Zealander made his Masters debut last year and ensured a return by maintaining his top-50 ranking at the close of last year. Enjoyed a strong finish to 2023 on the DP World Tour, winning his first Rolex Series title at the BMW PGA Championship and finishing tied second at the Alfred Dunhill Links Championship on his title defence in Scotland. Those performances helped him earn dual membership status with the PGA TOUR as one of the top 10 finishers not already exempt on the season-long Race to Dubai Rankings in Partnership with Rolex. His best result so far this year is a tie for 14th at the DP World Tour’s Dubai Invitational.
Tyrrell Hatton
- Birthplace: High Wycombe, England
- Official World Golf Ranking: 19
- Best Masters finish: T18 (2021)
The Englishman has made the cut on five of his seven visits to date at Augusta National, since his debut in 2017. Last year, he finished runner-up at both the BMW PGA Championship and THE PLAYERS Championship, prestige events on the DP World Tour and PGA TOUR respectively. He additionally made the cut in all four Majors and represented Europe in his third Ryder Cup at Marco Simone Golf & Country Club. Finished in the top 20 in his first two starts of the year on the PGA TOUR, before making the cut in Dubai on the DP World Tour. His most recent victory worldwide came at the Abu Dhabi HSBC Championship in 2021.
Ryo Hisatsune
- Birthplace: Okayama, Japan
- Turned Pro: 2020
- Official World Golf Ranking: 87
The Japanese is making his first Masters appearance and his Major Championship debut after receiving a special invite. Enjoyed a remarkable rookie season on the DP World Tour in 2023, after securing his card at the Qualifying School. Won his first DP World Tour title at the Cazoo Open de France in September, before becoming the first Japanese player to be named the DP World Tour's Sir Henry Cotton Rookie of the Year. He was one of ten players to earn 2024 PGA Tour membership through his performances on the DP World Tour last year. Has two top 20s so far this season on the PGA TOUR, with his best result a tie for 11th at The American Express in January.
Nicolai Højgaard
- Birthplace: Billund, Denmark
- Turned Pro: 2019
- Official World Golf Ranking: 38
The Dane is another first-timer teeing it up this week. Secured his invite to feature in the first men’s Major by breaking into the top 50 of the world with his maiden Rolex Series victory at the DP World Tour Championship at the end of last season, a week after finishing runner-up at the Nedbank Golf Challenge. Finished second at the AT&T Pebble Beach PRO-AM in February, days after finishing in the top 10 at the DP World Tour's Hero Dubai Desert Classic, during an impressive start to his first full season on the PGA TOUR. The three-time DP World Tour winner was a member of the winning European Ryder Cup team last year.
Viktor Hovland
- Birthplace: Oslo, Norway
- Official World Golf Ranking: 6
- Best Masters finish: T7 (2023)
Hovland earned low amateur honours in 2019 as Norway's first Masters participant and has yet to miss a cut at Augusta National in his first four appearances. Last year, he won three times on the PGA TOUR, while he recorded top-20 finishes in each of the four Majors. He also finished in a tie for second at the DP World Tour’s season-ending event in Dubai. Delivered 3.5 points from his five matches at the 2023 Ryder Cup in Italy. His best performance so far in 2024 came at the Genesis Invitational when he finished in a tie for 19th.
Min Woo Lee
- Birthplace: Perth, Australia
- Official World Golf Ranking: 32
- Best Masters finish: T14 (2022)
The Australian is making his third consecutive Masters appearing, having finished in the top 15 on his debut in 2022 after a record-tying 30 on the front nine of his final round. Registered six top 10s, including at The PLAYERS and U.S. Open, along with a victory on the Asian Tour, before getting his 2024 DP World Tour campaign off to a fast start with a title on home soil at the Fortinet Australian PGA Championship. Finished in a tie for second at the Cognizant Classic in the Palm Beaches on the PGA TOUR in March.
Shane Lowry
- Birthplace: Clara, Ireland
- Turned Pro: 2009
- Official World Golf Ranking: 36
- Best Masters finish: T3 (2022)
Boasts an encouraging record at Augusta National, with top 25s in each of the past four years, ahead of his ninth Masters start. His best result of 2023 saw him finish in a tie for third on home ground at the Horizon Irish Open, shortly before he helped Europe regain the Ryder Cup after receiving a Captain’s Pick from Luke Donald. Finished third and then a tie for fourth in back-to-back events on the PGA TOUR in March to climb back into the world’s top 50.
Rory McIlroy
- Birthplace: Holywood, Northern Ireland
- Turned Pro: 2007
- Official World Golf Ranking: 2
- Best Masters finish: 2 (2022)
McIlroy, who is making his 16th Masters appearance, is one win away from joining Gene Sarazen, Ben Hogan, Gary Player, Jack Nicklaus and Tiger Woods as winners of the career Grand Slam. Has top 10s in seven of the past 10 years at Augusta National, including a second place finished behind Scottie Scheffler in 2022. Successfully defended his title at the Hero Dubai Desert Classic in January as he claimed his third Rolex Series title. In 2023, he won in Dubai and Scotland on the DP World Tour as he earned his fifth Harry Vardon Trophy, finished inside the top seven in three majors, and produced a career-best performance to help the European team win the Ryder Cup. Picked up his first top-10 finish of the season on the PGA TOUR at the Valero Texas Open, the week before the Masters.
Adrian Meronk
- Birthplace: Hamburg, Germany
- Turned Pro: 2016
- Official World Golf Ranking: 57
- Best Masters finish: MC (2023)
Missed the cut on his Masters debut 12 months ago as he made history as Poland’s first participant in the tournament. Won twice on the DP World Tour in 2023 to become a four-time winner, and registered three further top-five finishes as he went on to be voted Seve Ballesteros Award after being voted Player of the Year by his peers. He finished runner-up at the Hero Dubai Desert Classic in January.
Joaquín Niemann
- Birthplace: Santiago, Chile
- Turned Pro: 2018
- Official World Golf Ranking: 93
- Best Masters finish: T16 (2023)
Registered his best Major performance at Augusta National 12 months ago, one of three occasions has made the cut in his first four appearances. He has top-five finishes in each of his three DP World Tour starts this season, including winning the ISPS HANDA Australian Open in a play-off last December.
Thorbjørn Olesen
- Birthplace: Hareskovby, Denmark
- Turned Pro: 2008
- Official World Golf Ranking: 72
- Best Masters finish: T16 (2013)
Five years on from his last Masters appearance, Olesen returns to Augusta National for a fourth time after receiving a special invite. The Dane is aiming to maintain his record of making the cut on each occasion, with his best finish coming on debut in 2013 when he finished in a tie for sixth. Victory at the Ras Al Khaimah Championship in February made it three DP World Tour titles in as many seasons, after victories in England in 2022 and Thailand in 2023. The 2018 Ryder Cup winner recorded seven additional top-10 finishes last season to earn 2024 PGA Tour membership.
Matthieu Pavon
- Birthplace: Toulouse, France
- Turned Pro: 2013
- Official World Golf Ranking: 25
One of the debutants at Augusta National this week. The Frenchman has enjoyed a life-changing period in world golf, since winning his maiden DP World Tour title in October during a stellar finish to the Race to Dubai Campaign which saw him record three further top-15 finishes, including at the DP World Tour Championship where he made four consecutive birdies, to earn PGA TOUR membership for 2024. Made history as the first French winner on the PGA TOUR since 1907 with victory at the Farmers Insurance Open in January. Two further global top 10s have since followed, most recently in Singapore on the DP World Tour.
- Birthplace: Barrika, Spain
- Official World Golf Ranking: 3
- Best Masters finish: 1 (2023)
The defending champion is making his eighth Masters appearance, having won his second Major with a four-stroke victory over Brooks Koepka and Phil Mickelson 12 months ago. Prior to his triumph at Augusta National, he had won three PGA TOUR titles earlier in the year and he went on to finish in a tie for second at The Open and register top-5 finishes in both the BMW PGA Championship and DP World Tour Championship. He is a ten-time winner on the DP World Tour, including a record five on the Rolex Series.
Justin Rose
- Birthplace: Johannesburg, South Africa
- Turned Pro: 1998
- Official World Golf Ranking: 58
- Best Masters finish: 2 (2015, 2017)
Boasts an enviable record at the Masters, with top-25 finishes in 14 of his 18 starts. Remarkably, 11 of those come in the past 13 years. Most notably, lost out in a play-off to Sergio Garcia in 2017. In 2023, he won for the 11th time on the PGA TOUR after a four-year wait with victory at the AT&T Pebble Beach Pro-Am. His best finish on the DP World Tour last year was a tie for fourth at the Betfred British Masters hosted by Sir Nick Faldo, while he made his sixth Ryder Cup appearance in Italy. He has missed the cut in his last two starts, with his best result so far this coming at Pebble Beach with a tie for 11th in his title defence.
- Birthplace: Adelaide, Australia
- Turned Pro: 2000
- Official World Golf Ranking: 52
- Best Masters finish: 1 (2013)
One of the past champions in the field, following his play-off triumph against Angel Cabrera in 2013. Now, Australia’s first Green Jacket winner is set for his 23rd Masters appearance. He has only missed the cut on two occasions, and not since 2009. Recorded top-10 finishes in his first three starts of the 2024 DP World Tour season and has since added another at the WM Phoenix Open on the PGA TOUR.
Sepp Straka
- Birthplace: Vienna, Austria
- Official World Golf Ranking: 29
- Best Masters finish: T30 (2022)
The Austrian is making his third consecutive appearance at the Masters. He hit his stride in 2023 after finishing in a tie for seventh at the US PGA Championship, and then won his second PGA TOUR title at the John Deere Classic in early July. Later that month he produced his career-best Major performance with a tie for second at The Open, before ending the PGA TOUR campaign at the Tour Championship in a share of sixth place. Part of the European team that regained the Ryder Cup in Italy. Has two top-20 finishes so far this year on the PGA TOUR, including at The PLAYERS Championship.
Erik van Rooyen
- Birthplace: Bellville, South Africa
- Official World Golf Ranking: 63
The South African is making his third Masters appearance, having missed the cut in both 2020 and 2022. Claimed his second PGA TOUR title at the World Wide Technology Championship in November, dedicating his triumph to a close friend, who died shortly afterwards due to terminal cancer. Finished in a tie for second in Palm Beach Gardens in March and also has a top 10 this season in Mexico. His one win on the DP World Tour so far came in Sweden in 2019.
Danny Willett
- Official World Golf Ranking: 245
- Best Masters finish: 1 (2016)
This marks the Englishman’s 10th Masters appearance. His career highlight so far came in 2016 when he shot a final-round 67 to overturn a three-stroke deficit and become England’s first Masters champion since Sir Nick Faldo won his third Green Jacket in 1996. Among his other prestigious victories are evens both the DP World Tour Championship, BMW PGA Championship and Alfred Dunhill Links Championship. Struggled for form last year, and he has not played since undergoing shoulder surgery following the BMW PGA Championship in September.
Svensson caps off stunning 12 months with inaugural DP World Tour victory
Jesper Svensson became a winner on the DP World Tour for the first time at the Porsche Singapore Classic last month, continuing his remarkable rise up the golfing ranks which began on the Challenge Tour in 2023.
Challenge Tour Partners
Masters 2024 Leaderboard: First round scores for golf leaders Thursday at Augusta
Follow live updates from all the Masters action here or check out our hole-by-hole analysis of Tiger Woods' first round at Augusta National.
The best golfers in the world have once again converged at Augusta National Golf Club in search of the coveted green jacket. This is the 88th edition of the annual Masters Tournament , so will we see a brand new winner or will one of the 18 former winners playing in this year's tournament claim the championship yet again?
Day 1 proved to be exhilarating. Not only did we see the early tournament favorite, Scottie Scheffler , prove why so many people were backing him to win, but a few dark horses emerged as well. LIV's Bryson DeChambeau leads the entire field after Day 1, shooting 7-under par on Thursday. Other golfers who weren't given great odds to start the tournament like Danny Willet (-5) and Nicolai Højgaard (-4) also played some incredible rounds. Even Tiger Woods had some great moments and moves into Day 2 ranking inside the top-20 at 1-under.
Three more days are left in this year's tournament, with one round played each day. The cut will happen after Day 2, and only the top-50 players on the leaderboard will advance into Saturday and Sunday. It's unforgiving, but what else can you expect from a tournament as heralded as The Masters.
Here is everything of not to happen during Day 1 of the 2024 Masters Tournament.
Tiger Woods live: Updates, score and tracker for golf icon at Augusta on Day 1 Thursday
Masters Leaderboard 2024:
- Bryson DeChambeau, -7
- Scottie Scheffler, -6
- Nicolai Højgaard, -5
- Danny Willet, -4
- Max Homa, -4
- Ryan Fox, -3
- Cameron Davis, -3
- Tyrell Hatton, -3
- Corey Conners, -2
- Byeong Hun An, -2
Masters Leaderboard: Full updated scores for Augusta leaders
Weather in Augusta, Georgia delays Masters start
The start of the Masters' first round was delayed Thursday morning due to rainy and windy conditions.
Thunderstorms are likely to end around 10 a.m. ET, and the first round is now scheduled to begin at 10:30 a.m. ET.
2024 Masters Tournament morning tee times: Day 1 Thursday
Note: The start of the Masters' first round was delayed due to poor weather conditions. The following tee times have been updated accordingly.
10:30 a.m.: Erik van Rooyen, Jake Knapp
10:42 a.m.: Jose Maria Olazabal, Taylor Moore, Santiago de la Fuente (A)
10:54 a.m.: Danny Willett, Austin Eckroat, Stephan Jaeger
11:06 a.m.: Charl Schwartzel, Luke List, Christo Lamprecht (A)
11:18 a.m.: Gary Woodland, Thorbjorn Olesen, Bryson DeChambeau
11:30 a.m.: Zach Johnson, Corey Conners, Jasper Stubbs (A)
11:42 a.m.: Sergio Garcia, Chris Kirk, Ryan Fox
11:54 a.m.: Lucas Glover, Byeong Hun An, Harris English
12:06 p.m.: Phil Mickelson, Sepp Straka, Tony Finau
12:18 p.m.: Nick Taylor, Joaquín Niemann, Russell Henley
12:36 p.m.: Patrick Cantlay, Min Woo Lee, Rickie Fowler
12:48 p.m.: Hideki Matsuyama, Will Zalatoris, Justin Thomas
1 p.m.: Jon Rahm, Matt Fitzpatrick, Nick Dunlap
1:12 p.m.: Scottie Scheffler, Rory McIlroy, Xander Schauffele
1:24 p.m.: Wyndham Clark, Viktor Hovland, Cameron Smith
1:36 p.m.: Lee Hodges, Adrian Meronk, Grayson Murray
1:48 p.m. Camilo Villegas, Denny McCarthy, Cameron Davis
2 p.m.: Mike Weir, Ryo Hisatsune, Neal Shipley
2:12 p.m. Vijay Singh, Si Woo Kim, Emiliano Grillo
2:24 p.m.: Fred Couples, Adam Hadwin, Stewart Hagestad
2:42 p.m.: Justin Rose, Eric Cole, Peter Malnati
2:54 p.m.: Akshay Bhatia, J.T. Poston, Shane Lowry
2024 Masters Tournament schedule and how to watch
The Masters begins Thursday, April 11 and run through Sunday, April 14.
Round 1: Thursday, April 11
- Starting at 10:30 a.m. ET
- TV coverage: 3:00-7:30 p.m. ET
- Channel: ESPN
- Streaming: Masters.com (simulcast), CBSSports.com (desktop and mobile), CBS Sports App (desktop and mobile), ESPN+, Paramount+
How to watch: Catch Masters action with an ESPN+ subscription
Round 2: Friday, April 12
- Starting at 8:30 a.m. ET
Round 3: Saturday, April 13
- Starting at 10:00 a.m. ET
- TV coverage: 3:00-7:00 p.m. ET
- Channel: CBS
- Streaming: CBSSports.com (simulcast), Paramount+ (simulcast), CBS Sports app (simulcast)
Round 4: Sunday, April 14
- TV coverage: 2:00-7:00 p.m. ET
We occasionally recommend interesting products and services. If you make a purchase by clicking one of the links, we may earn an affiliate fee. USA TODAY Network newsrooms operate independently, and this doesn’t influence our coverage.
Gannett may earn revenue from sports betting operators for audience referrals to betting services. Sports betting operators have no influence over nor are any such revenues in any way dependent on or linked to the newsrooms or news coverage. Terms apply, see operator site for Terms and Conditions. If you or someone you know has a gambling problem, help is available. Call the National Council on Problem Gambling 24/7 at 1-800-GAMBLER (NJ, OH), 1-800-522-4700 (CO), 1-800-BETS-OFF (IA), 1-800-9-WITH-IT (IN). Must be 21 or older to gamble. Sports betting and gambling are not legal in all locations. Be sure to comply with laws applicable where you reside.
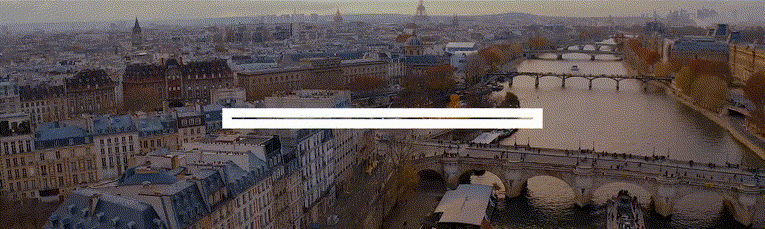
IMAGES
COMMENTS
Pan-KRAS degrader. IND in 2023. NA. Boehringer Ingelheim. Pan-KRAS degrader. Preclinical. NA. ... They published on this tour-de-force effort, and the resulting compound MRTX1133, in 2021 ...
Fast forward to March 2023, when Dr. Barbacid opened the AACR Special Conference: Targeting RAS by commemorating the 10 th anniversary of a seminal 2013 paper by Kevan Shokat, PhD, FAACR, that refuted KRAS's status as an "undruggable" protein. Dr. Shokat discussed this research in an interview with AACR Stories.
They published on this tour-de-force effort, and the resulting compound MRTx1133, in 2021. "There are probably four chemical ... NA Aella Pan-KRAS degade IND in 2023 NA Boehinge Ingelheim Pan-KRAS degade Peclinical NA Boehinge Ingelheim Pan-KRAS inhibio Peclinical New on-state inhibitors
The KRAS tour: Studying metabolic reprogramming in isogenic pancreatic cancer organoids Cell Stem Cell. 2024 Jan 4;31(1):1-2. doi: 10.1016/j.stem.2023.12.010. Authors Shree Bose 1 , Xiling Shen 2 Affiliations 1 Department of Internal Medicine, University of Chicago, Chicago, IL, USA. 2 Terasaki Institute for Biomedical Innovation, Los Angeles ...
The discovery that covalent small molecules could reversibly bind switch-II pocket (SII-P) in KRAS G12C mutants led to the rapid development of several targeted therapies agents including sotorasib (Amgen) and adagrasib (Mirati Therapeutics), both of which were granted accelerated FDA approval for treatment of KRAS G12C-mutated non-small cell ...
The recent design of mutation-selective KRAS inhibitors has led to US Food and Drug Administration approval of two inhibitors of KRAS(G12C), sotorasib and adagrasib. ... 1060-1062 (2023). https ...
Cell Stem Cell. et al. performed one such study, using a new platform of isogenic murine pancreatic organoids that were either wild type (WT) or with canonical PDAC mutations in alone (KC; KRAS KRASG12D), with mutation (KPC; TP53 KRASG12D, Tp53R172H), with loss of (KSC; KRASG12D, SMAD4 / ), SMAD4 or with all three (KSPC; KRASG12D, Tp53R172H ...
One of the most significant breakthroughs in oncology in recent years is the ability to target KRAS. KRAS is the most commonly mutated oncogene in human cancer, with greatest frequency in non-small-cell lung cancer (NSCLC), colorectal cancer, and pancreatic cancer. Since the gene's discovery several decades ago it had been considered an undruggable target with many failed clinical trials.
KRAS mutations and real-world survival on FTD/TPI treatment. We next collected real-world data of 960 patients with mCRC who were treated with FTD/TPI in 36 centers across Italy and the UK ...
Grant support. RSP-248-18/19/Tour de Cure. Colorectal cancer is one of the world's most prevalent and lethal cancers. Mutations of the KRAS gene occur in ~40% of metastatic colorectal cancers. While this cohort has historically been difficult to manage, the last few years have shown exponential growth in the development of selective inhibito ….
DOI: 10.1016/j.stem.2023.12.010 Corpus ID: 266786395; The KRAS tour: Studying metabolic reprogramming in isogenic pancreatic cancer organoids. @article{Bose2024TheKT, title={The KRAS tour: Studying metabolic reprogramming in isogenic pancreatic cancer organoids.}, author={Shree Bose and Xiling Shen}, journal={Cell stem cell}, year={2024}, volume={31 1}, pages={ 1-2 }, url={https://api ...
Using an isogenic organoid platform to model pancreatic cancer, Duan et al. establish an important link between mutant KRAS and cholesterol metabolism and identify perhexiline maleate as a possible therapeutic to target this relationship.
RASDak stands for Ride Across South Dakota. RASDak is an annual bicycle ride to enjoy the beautiful scenery and hospitality of South Dakota, the "Land of Infinite Variety.". Our state is home to a variety of landscapes from rolling prairie to the Black Hills. The best way to experience these landscapes and South Dakota is by bike! While the ...
The UAE Tour Ed. 2023; ... Ras Al Khaimah Stage. 176 km. From Al Marjan Island. To Jebel Jais. Climb More Info. Stage 4. Thu, February 22. Dubai Stage. ... Download the official app of the UAE Tour. This application for cycling fans makes it possible to view exclusive content: live, stages description, rankings, photos, videos and much more. ...
Racing; News Tour de France 2023 route: Every stage of the 110th edition in detail. This year's race has kicked off in Bilbao, in Spain's Basque Country.
James Moultrie is a gold-standard NCTJ journalist who joined Cyclingnews as a News Writer in 2023 after originally contributing as a freelancer for eight months, during which time he also wrote ...
Spanish golfer, LIV participant and 2023 Masters champion Jon Rahm will be looking to become the fourth golfer in Masters history to win back-to-back jackets and the first since Tiger Woods in ...
The GTPase KRAS is somatically mutated in around 10% of all cancers, including about 90% of pancreatic adenocarcinoma, 40% of colorectal adenocarcinoma, 35% of lung adenocarcinoma and 20% of ...
In this issue of Cell Stem Cell, Duan et al. performed one such study, using a new platform of isogenic murine pancreatic organoids that were either wild type (WT) or with canonical PDAC mutations in KRAS alone (KC; KRAS G12D), with TP53 mutation (KPC; KRAS G12D, Tp53 R172H), with loss of SMAD4 (KSC; KRAS G12D, SMAD4 −/−), or with all three (KSPC; KRAS G12D, Tp53 R172H, SMAD4 −/−).
Defending Tour de France champion Jonas Vingegaard is in hospital after suffering a horror crash during stage four of the Tour of the Basque Country on Thursday.. His cycling team, Team Visma ...
Tour de kras Další ročník se uskuteční 23.7.-24.7.2022. Jedná se o recesní akci, která spočívá v jízdě na kolech po Moravském krasu a restauračních zařízeních. V roce 1992 se uskutečnila první spanilá jízda cyklistů a velocipedistů. Největší počet účastníků byl v roce 2011.
Moscow Metro private tours. 2-hour tour $87: 10 Must-See Moscow Metro stations with hotel pick-up and drop-off. 3-hour tour $137: 20 Must-See Moscow Metro stations with Russian lunch in beautifully-decorated Metro Diner + hotel pick-up and drop off. Metro pass is included in the price of both tours.
Champions from 1947 to 2023. Adam Yates (UAE Team Emirates) won the overall at 2023 Tour de Romandie(Image credit: Dario BelingheriGetty Images) Tour de Romandie past winners. Year. Rider Name ...
Toll Free 0800 011 2023; Day tours. Tours by Region. Moscow Saint Petersburg Golden Ring Lake Baikal Murmansk Siberia ... Tour Types. Small Group Tours Private Tour Theme Tour. Theaters. Moscow events St. Petersburg events Events archive; Events by type. Ballet Opera Concert Show All types. Top theaters.
The Moscow Metro system is full of art, but there are hundreds of stations. Eliminate the risk of getting lost in the vast network, or missing the most important stations. On this handy private tour you'll be taken to the most interesting and impressive art and architectural examples, and learn all about their history and cultural significance from your local guide.
Victory at the Ras Al Khaimah Championship in February made it three DP World Tour titles in as many seasons, after victories in England in 2022 and Thailand in 2023. The 2018 Ryder Cup winner recorded seven additional top-10 finishes last season to earn 2024 PGA Tour membership.
The Moscow Metro is famous for its elaborate decorations and beautiful artworks. On this tour of the underground transportation system you'll learn about Russian culture, art, history, technology and more, all in one convenient trip. Your local guide will be able to point out details that you might miss if you were traveling by yourself. This is a fun way to travel around Moscow while ...
Mistral's big torrent file with a mixture of experts. While the capabilities of Mixtral 8x22B remain undisclosed, AI enthusiasts were quick to point out that this is a big release from Mistral ...
2024 Masters Tournament morning tee times: Day 1 Thursday. Note: The start of the Masters' first round was delayed due to poor weather conditions. The following tee times have been updated ...