- Skip to primary navigation
- Skip to main content
- Skip to primary sidebar
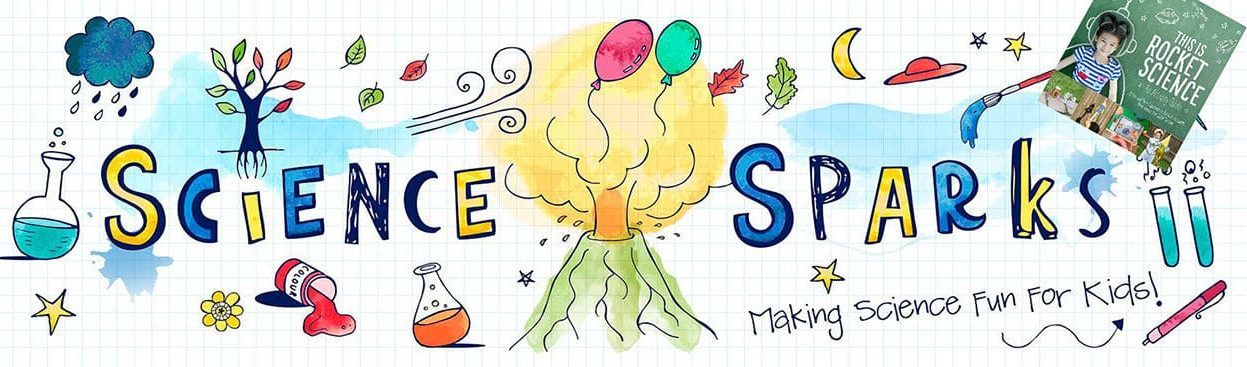
- FREE Experiments
- Kitchen Science
- Climate Change
- Egg Experiments
- Fairy Tale Science
- Edible Science
- Human Health
- Inspirational Women
- Forces and Motion
- Science Fair Projects
- STEM Challenges
- Science Sparks Books
- Contact Science Sparks
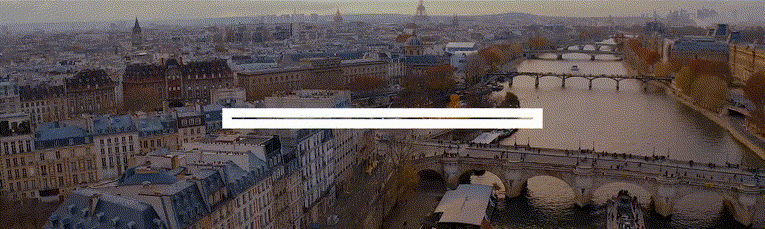
Viscosity Races – investigating the flow of liquids
April 23, 2012 By Emma Vanstone 14 Comments
It is so annoying when getting to the end of a ketchup bottle, and you have to keep banging to get that last bit out. Why is this? Because ketchup is thick and does not flow as easily as other fluids. We call this resistance to flowing viscosity .
The rate at which different fluids flow can vary considerably.
So how about having races to test them?
What you will need:
Stopwatch/tape measure
A ramp – we used a table that we took two legs off.
Different fluids to test
We used whole milk, ketchup, chocolate sauce, orange juice and cooking oil
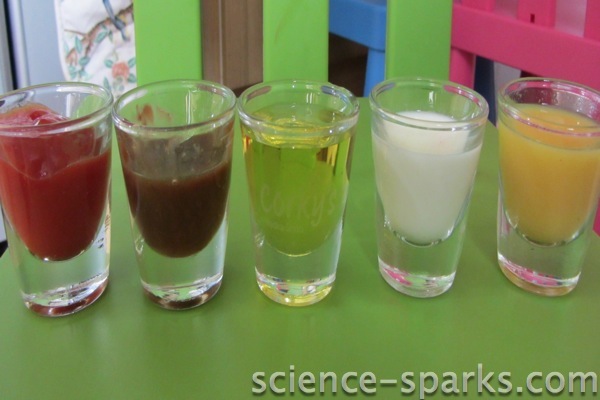
Deciding what to measure
- you could decide to time how long it takes for all five fluid to reach the bottom of the table
- you could decide to measure how far they have travelled after a certain time
How to make the test fair
You need to consider the things that could impact on it not being a fair test – this could include things like
- ensuring the volume use use is the same for all fluids
- ensuring that you time the flow for the same distance
- ensuring that measure after a set time for each.
- ensuring you repeat the investigation 3 times and take a mean result
- The gradient of the ramp is the same for each fluid
You can either do one at a time – this is easier for recording time, or tip them all at the same time if you want them to get to the bottom of the table.
Let them flow for the set time you have decided, or time them until they all reach the bottom.
You can record your results in a table.
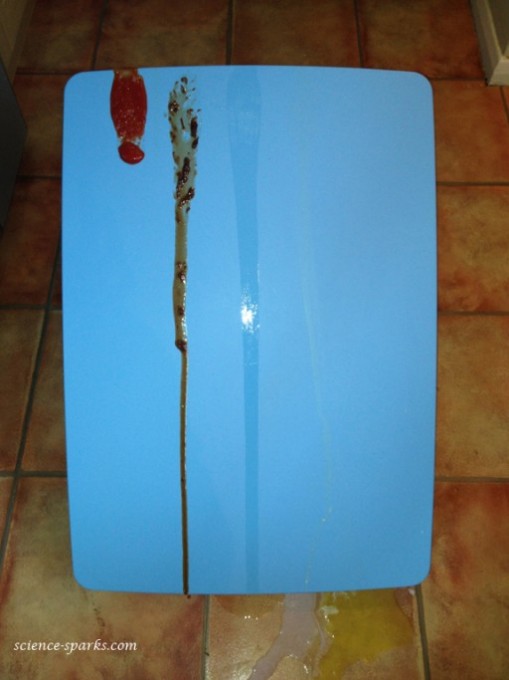
Results
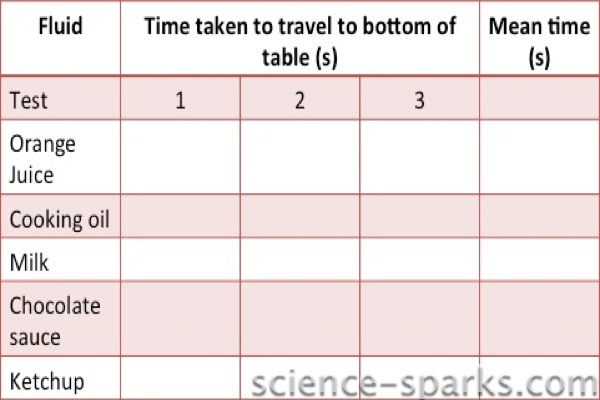
We decided to wait until they got to the bottom but had to stop the experiment as it was clear the ketchup was never going to make it! Next time we will try with measuring the distance travelled!
Last Updated on March 9, 2023 by Emma Vanstone
Safety Notice
Science Sparks ( Wild Sparks Enterprises Ltd ) are not liable for the actions of activity of any person who uses the information in this resource or in any of the suggested further resources. Science Sparks assume no liability with regard to injuries or damage to property that may occur as a result of using the information and carrying out the practical activities contained in this resource or in any of the suggested further resources.
These activities are designed to be carried out by children working with a parent, guardian or other appropriate adult. The adult involved is fully responsible for ensuring that the activities are carried out safely.
Reader Interactions
April 23, 2012 at 8:44 am
Thanks for inspiring me. I just love your blog.
April 23, 2012 at 10:30 am
I help to run an after school science club for KS2 children – this looks like a great mixture of messiness and investigation for some of our younger ones. Brilliant! Thank you!
April 23, 2012 at 5:10 pm
What a very fun experiment! Races are always fun.:)
April 24, 2012 at 9:29 am
This looks fun 🙂 Thanks for joining us at Creative Mondays 🙂
April 24, 2012 at 10:23 am
I never knew it was called that. I love how you have introduced fair testing too.
April 25, 2012 at 4:47 pm
This is such a great idea! My kids would love doing this!!! Thanks!
April 25, 2012 at 10:27 pm
What a fun idea. We’ll be saving this one for our kindergarten year. Nicely done!
April 26, 2012 at 8:07 pm
I love, LOVE the title of this post! Viscosity races – awesome. And the prediction making and conclusion making possibilities…this is great science learning and fun.
May 01, 2012 at 9:17 pm
Oooh what fun fun fun! Science really is brilliant and you guys have so many great ideas each week!
Thank you for sharing on Kids Get Crafty!
Maggy & Alissa
October 10, 2012 at 10:49 am
ohhhh how much i love science l just love it that much soo does joshua stanley from cathedral academy 🙂 <3
January 17, 2013 at 7:35 am
Neat! I think my 3-year-old would like this a lot…races and condiments all in the same science activity.
February 24, 2016 at 2:19 pm
i like peanut butter
February 13, 2017 at 11:07 pm
Good day I would like to ask if there is a specific measurements like the measure of height and length of the ramp. btw thank you for the information.
February 19, 2022 at 6:17 pm
My students will LOVE this! Thank you!
Leave a Reply Cancel reply
Your email address will not be published. Required fields are marked *
Cool Science Experiments Headquarters
Making Science Fun, Easy to Teach and Exciting to Learn!
Science Experiments
Viscosity of Liquids Science Experiment
Viscosity? If you’ve never heard this word before you might think it’s a new brand of kitchen cleaner! But of course, if it’s not a kitchen cleaner, what in the world is it?
We’ll help define viscosity in our easy to understand explanation of how it works below, but the goal of this experiment is to help kids ‘see’ viscosity in action.
Collect your materials, print out our detailed instructions, and watch our demonstration video to explore how the consistency of a liquid impacts objects.
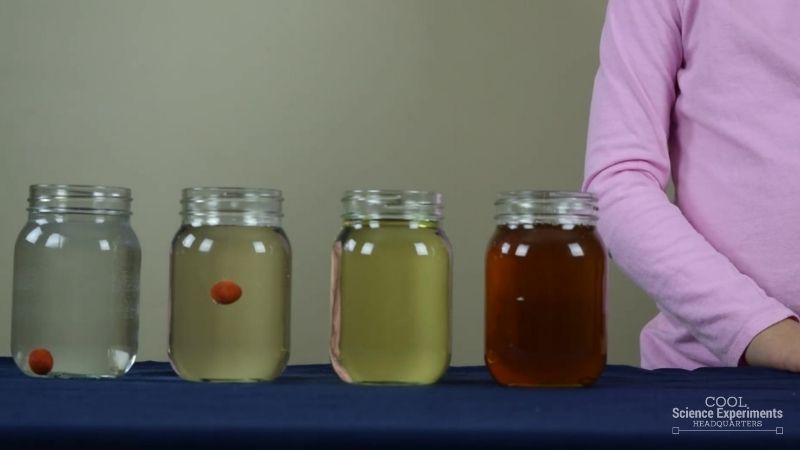
JUMP TO SECTION: Instructions | Video Tutorial | How it Works
Supplies Needed
- 4 clear glass jars of the same size (we used pint-sized mason jars)
- Water (enough to fill one jar)
- Corn Syrup (enough to fill one jar)
- Cooking Oil (enough to fill one jar) We used Vegetable Oil, but any Cooking Oil will work.
- Honey (enough to fill one jar)
Viscosity of Liquids Science Lab Kit – Only $5
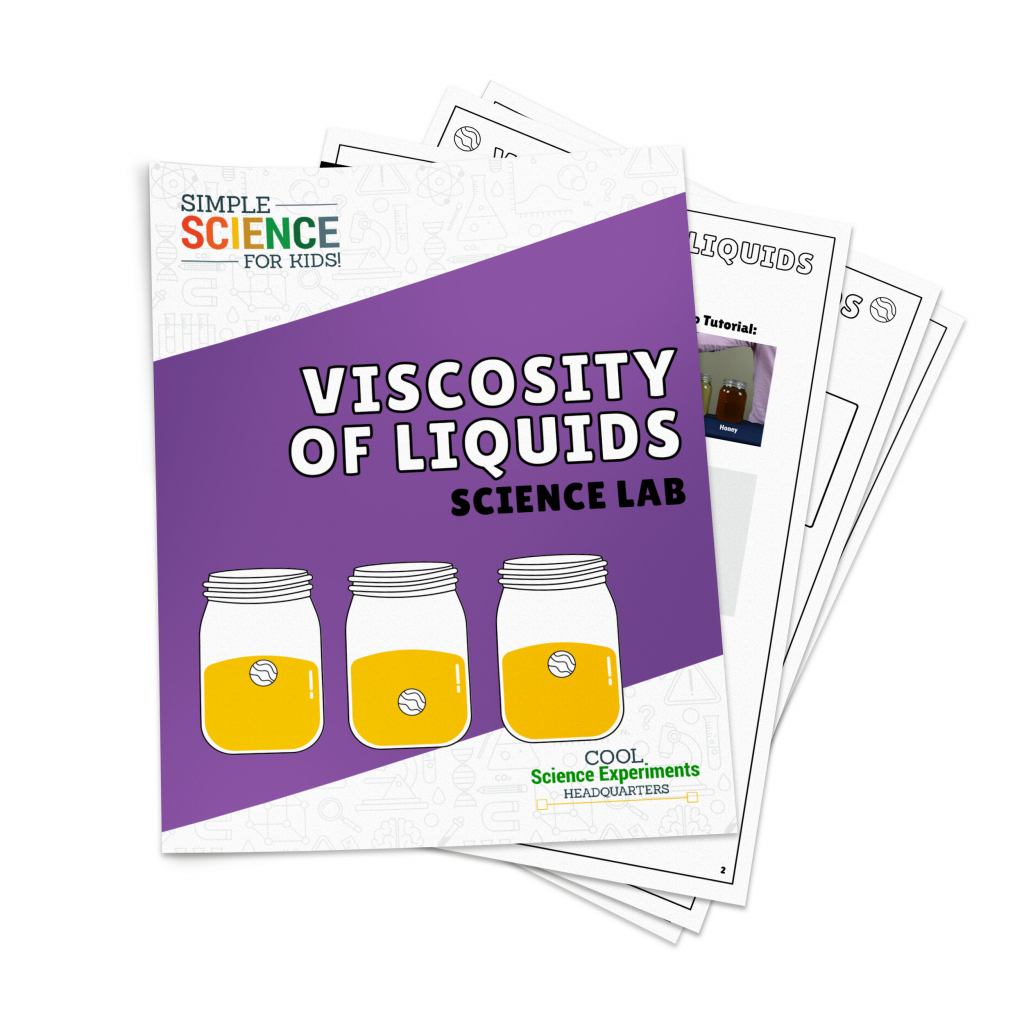
Use our easy Viscosity of Liquids Science Lab Kit to grab your students’ attention without the stress of planning!
It’s everything you need to make science easy for teachers and fun for students — using inexpensive materials you probably already have in your storage closet!
Viscosity of Liquids Science Experiment Instructions
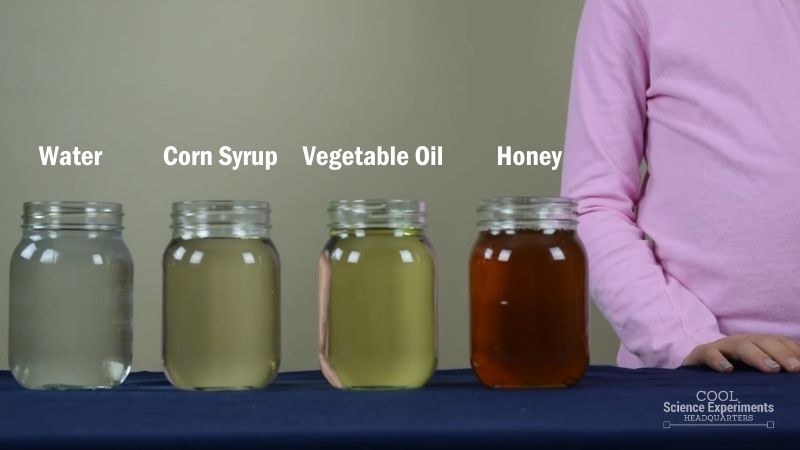
Step 1 – Gather four clear glass jars and fill one with water, one with corn syrup, one with cooking oil (we used vegetable oil, but any cooking oil will work) and one with honey.
As you are pouring the liquids, take a moment to make observations. What do you notice as you pour the water into the glass? What about the corn syrup, the cooking oil and the honey? Did you notice anything different?
Do you think the liquid will impact what happens when a marble is placed into each jar? What do you think will happen? Write down your hypothesis (prediction) and then continue the experiment to test it out and to find out if you were correct.
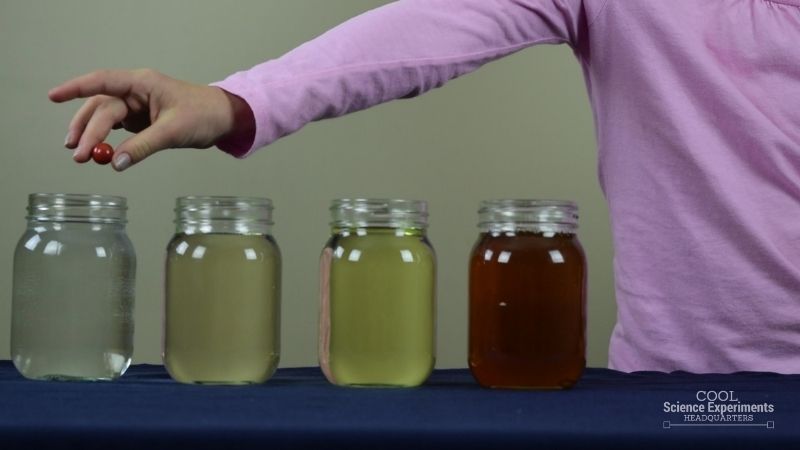
Step 2 – Carefully drop one marble into each jar. Drop one marble at a time and observe what happens to the marble when it enters the liquid. You’ll notice right away that the marble behaves differently in each jar. Was your hypothesis correct? Do you know why some marbles sink to the bottom of the jar quickly and some marbles sink to the bottle of the jar slowly?
Find out the answer in the how does this experiment work section below. It also contains ideas on how you can expand on the experiment.
Viscosity of Liquids Science Experiment Video Tutorial
How Does the Science Experiment Work?
The question answered in this experiment is: how does the consistency of a liquid impact how long it will take for a marble to sink in a jar of that liquid? A unique property of liquids is something called viscosity.
Viscosity is a liquid’s resistance to flowing.
Viscosity depends on the size and shape of the particles that make the liquid, as well as the attraction between the particles. Liquids that have a LOW viscosity flow quickly (ie. water, rubbing alcohol, and vegetable oil). Liquids that have a HIGH viscosity flow slowly (ie. honey, corn syrup, and molasses). Viscosity can also be thought of as a measure of how “thick” a liquid is. The more viscous (or thick) a liquid is, the longer it will take for an object to move through the liquid.
In our experiment, the marbles took longer to sink when dropped into the jars filled with corn syrup and honey than they did when dropped into the jars filled with water and cooking oil. Therefore, we’ve shown that corn syrup and honey have a higher viscosity (or are more viscous) than water and cooking oil.
More Science Fun
- How long will it take? Expand on the experiment, by estimating how long it will take for the marble to sink to the bottom of the jar? Then set a timer and find out how close your estimate was. Tip: Timing the marble, works best when using liquids that have a high viscosity (ie. honey, corn syrup, and molasses).
- The Pouring Test – When you are finished dropping the marbles into the jars, try pouring the liquids one at a time into another jar. You will notice that it takes longer to pour out the Corn Syrup and Honey than it does to pour out the Water and Cooking Oil. This is because the viscosity of a liquid can also be observed by how slow (or fast) it takes to pour the liquid.
- How Does the Consistency of a Liquid Impact Magnetic Attraction – This experiment shows how the viscosity of a liquid impacts how fast (or slow) the objects move toward a magnet.
I hope you enjoyed the experiment. Here are some printable instructions:
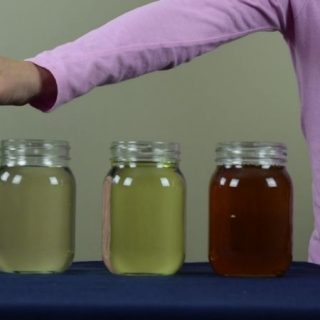
Viscosity of a Liquid Experiment Science Experiment
- 4 clear glass jars of the same size (we used pint sized mason jars)
- Cooking Oil (enough to fill one jar)
Instructions
- Gather four clear glass jars and fill one with water, one with corn syrup, one with cooking oil and one with honey.
- Carefully drop one marble into each jar. Drop one marble at a time and observe what happens to the marble when it enters the liquid. Which marbles sink to the bottom of the jar quickly and which marbles sink to the bottle of the jar slowly.

Reader Interactions
December 13, 2017 at 5:00 pm
The honey and corn syrup has a higher density than the water and oil because ther are more particals in a certain amount of space making it slower for the marball to sink to the bottom.
April 28, 2019 at 1:51 pm
Some liquids are less dense. Some liquids are more dense. The denser liquids make the marbles flow slower. The less dense liquids (water and oil) make the marbles flow faster. The more dense liquids (honey and corn syrup) make the marbles flow slower.
September 17, 2019 at 7:37 am
Viscosity is a measure of a fluid’s resistance to flow. It describes the internal friction of a moving fluid. A fluid with large viscosity resists motion because its molecular makeup gives it a lot of internal friction. A fluid with low viscosity flows easily because its molecular makeup results in very little friction when it is in motion…… So it is to do with the size and shape of the molecule rather than the density. If you heat up a liquid the density will change slightly but the viscosity will change a lot.
August 22, 2020 at 12:33 am
Honey is much thicker than oil, so the process is a little slower than the marble goes to the bottom of the honey.
Leave a Reply Cancel reply
Your email address will not be published. Required fields are marked *
Save my name, email, and website in this browser for the next time I comment.
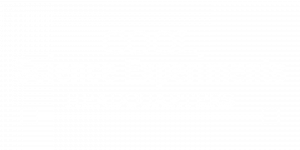
- Privacy Policy
- Disclosure Policy
Copyright © 2024 · Cool Science Experiments HQ
Volcanoes - Activity 4
Viscosityrace.
Objectives:
- determine the viscosity of some liquids; and
- describe the flow of gas in different liquids
- four pieces of cone out of a cardboard
- clear drinking glass
- drinking straw
- Before doing the activity, predict which liquid (water, syrup, honey, and cooking oil) takes the least amount of time to reach the tray. Which liquid will take the most amount of time?
- Place the cone marked A, B, C, and D on the tray.
- Pour water on the top of cone A. Record the time it takes for the water to reach the tray.
- Do it three times for each material.
NOTE: Use the same amount of material each time.
Q1. Is your prediction correct?
Q2. Which liquid is the most viscous? How do you know?
Q3. Which liquid is the least viscous?
Q4. Explain viscosity in your own words.
Q5. Compare how these liquids flow withto how you think lava flows. Why does some types of lava travel faster than others?
- Put 100 mL syrup in a clear glass.
- Using a drinking straw, blow some air from the bottom of the liquid. Observe.
- Repeat procedure 7 by blowing harder on itthe liquid. Observe.
Q6. Compare the movement of the liquid as the bubbles move on the surface.
In activity 3, you have observed that different liquids have different viscosities. If the liquid represents the magma, then its rate of flow depends on several factors. In the same way, you have seen in this activity that the amount of gas affects the viscosity of the liquid.
What type of magma do you think the Mayon Volcano ejects? Why do you say so?
Types of volcanic eruptions
Volcanoes erupt differently.They are generally classified as wet or dry depending on the magma’s water content. Volcanoes are described according to the style of eruption as follows:
- Phreatic or hydrothermal – is a stream-driven eruption as the hot rocks come in contact with water. It is short-lived, characterized by ash columns but may be an onset for of a larger eruption. Shown on the right is our Taal Volcano in Batangas.
- Phreatomagmatic – is a violent eruption due to the contact between water and magma. As a result, a large column of very fine ash and high-speed and sideway emission of pyroclastics called base surges are observed.
Fig. 7. Taal Volcano (Photo credits: USGS)
- Strombolian -- a periodic weak to violent eruption characterized by fountain lava, just like the Irazu Volcano in Costa Rica.
Fig. 8. Irazu Volcano (Photo credit: USGS)
- Vulcanian – characterized by tall eruption columns that reach up to 20 km high with pyroclastic flow and ashfall tephra like that of Paricutin Volcano in Mexico.
Fig. 9. Paricutin Volcano (Photo credits: USGS)
- Plinian – excessively explosive type of eruption of gas and pyroclastics, just like our Pinatubo Volcano in Zambales.
Fig. 10. Pinatubo Volcano (Photo credits: USGS)
Now that you have learned about the factors that affect the viscosity of magma, it’s time to find out how the type of material emissions affects the volcano’s slope.
Understanding Science
How science REALLY works...
Teaching Resources
Traveling through different liquids.
Grade Level(s):
- Janulaw, Sharon
Resource type:
- classroom activity
Discipline:
- Physical Sciences
Time: 40 minutes
Learners will observe and record what happens when they manipulate bottles containing one liquid and an object. They will compare bottles that have an object and different liquids. They will observe and record what happens when they manipulate bottles containing one liquid and more than one object.
Go to this resource »
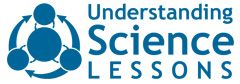
- Teaching tips
- Teaching background
- [What is science?: Grades K-2] Scientists ask and address questions. (P1, P3, NOS4)
- [What is science?: Grades K-2] Scientists base their ideas on evidence from the natural world. (P3)
- [What is science?: Grades K-2] Scientific ideas may change based on what we observe and experience. (NOS3)
- [How science works: Grades K-2] Scientists observe, explore, discover, and communicate with one another.
- [How science works: Grades K-2] Scientific observations can be made directly with our own senses or may be made indirectly through the use of tools. (P3)
- [How science works: Grades K-2] Scientists don't always agree with one another. (P7)
- [The social side of science: Grades K-2] Scientists share their ideas with each other. (P7, P8)
- [The social side of science: Grades K-2] Scientists work together.
There are no NGSS/DCI concepts currently linked to this resource.
- Exploration and discovery
Subscribe to our newsletter
- Understanding Science 101
- The science flowchart
- Science stories
- Grade-level teaching guides
- Teaching resource database
- Journaling tool
- Misconceptions
Get Your ALL ACCESS Shop Pass here →

Viscosity Experiment With Marbles
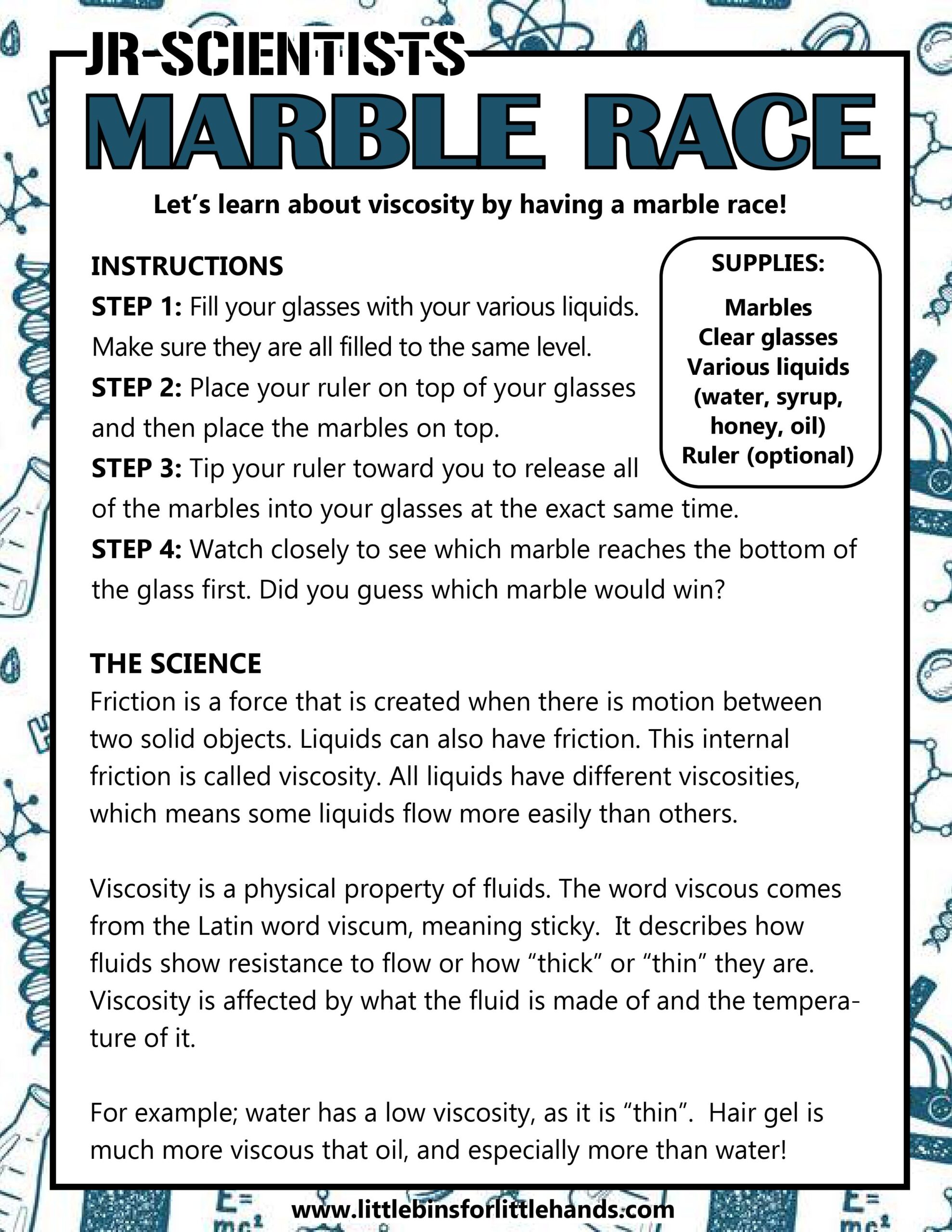
The fun thing about science experiments for kids is that you can set them up easily and quickly with what you already have! Learn about the viscosity of fluids with a simple viscosity experiment . Grab some marbles and find out which one will fall to the bottom first. We love science experiments that are fun and easy to do!
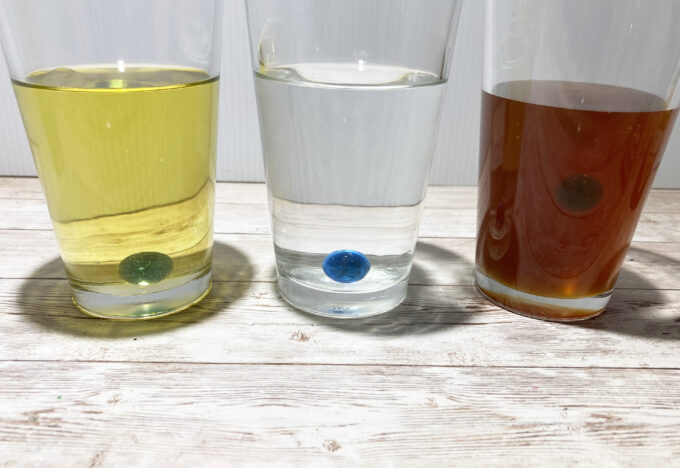
What Is Viscosity?
Friction is a force that is created when there is motion between two solid objects. Liquids can also have friction. This internal friction is called viscosity .
All liquids have different viscosities, which means some liquids flow more easily than others. Viscosity is a physical property of fluids. The word viscous comes from the Latin word viscum, meaning sticky. It describes how fluids resist flow or how “thick” or “thin” they are.
Viscosity is affected by what the fluid is made of and the temperature of it. For example, water has a low viscosity, as it is “thin.” Hair gel is much more viscous than oil and significantly more than water!
Learn about the viscosity of fluids by having a marble race. Try this fun marble drop experiment below! You could even turn it into an easy viscosity science project.
- Clear glasses
- Various liquids (water, syrup, honey, oil)
- Ruler (optional)
Instructions:
STEP 1: Fill your glasses with your various liquids. Make sure they are all filled to the same level.
Learn more about using the scientific method for kids.

STEP 2: Place your ruler on top of your glasses and then place the marbles on top.
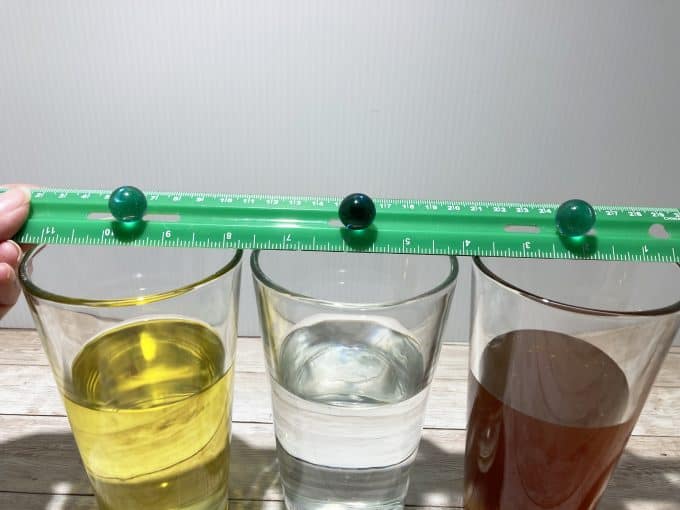
STEP 3: Tip your ruler toward you to release all of the marbles into your glasses at the exact same time.
STEP 4: Watch closely to see which marble reaches the bottom of the glass first. Did you guess which marble would win?

Using The Scientific Method
The scientific method is a process or method of research. A problem is identified, information about the problem is gathered, a hypothesis or question is formulated from the information, and the hypothesis is tested with an experiment to prove or disprove its validity.
Sounds heavy… What in the world does that mean?!? It means you don’t need to try and solve the world’s biggest science questions! The scientific method is all about studying and learning things right around you.
As children develop practices that involve creating, gathering data evaluating, analyzing, and communicating, they can apply these critical thinking skills to any situation.
LEARN MORE HERE: Using The Scientific Method with Kids
Note: The use of the best Science and Engineering Practices is also relevant to the topic of using the scientific method. Read more here and see if it fits your science planning needs.
Helpful Science Resources
Here are a few resources that will help you introduce science more effectively to your kiddos or students. Then you can feel confident yourself when presenting materials. You’ll find helpful free printables throughout.
- Best Science Practices (as it relates to the scientific method)
- Science Vocabulary
- 8 Science Books for Kids
- All About Scientists
- Science Supplies List
- Science Tools for Kids
- Join us in the Club
Get your FREE printable viscosity science project!

More Fun Viscosity Experiments To Try
Kids can use common household materials to try more viscosity experiments!
1. Cornstarch and Water: Oobleck!
Mix cornstarch with water in a bowl until you get a gooey substance. Have the kids try to stir the mixture slowly and then quickly. Discuss how the mixture behaves differently at different speeds, demonstrating its non-Newtonian properties.
2. Honey and Syrup Races
Fill two identical containers with honey and syrup. Have the kids tip the containers simultaneously, observe, and discuss which one flows faster. This demonstrates the different viscosities of honey and syrup.
3. Oil and Water Exploration
Fill a transparent container with water and drop some cooking oil into it. Observe how the oil forms droplets and floats on the water due to its lower viscosity. Discuss why the oil and water don’t mix.
Extend this viscosity experiment with alka seltzer tables. See lava lamp experiment.

4. Bubble Fun with Dish Soap
Mix dish soap with water to create a bubble solution. Use different amounts of soap to create solutions with varying viscosities. Have the kids blow bubbles and observe how the size and stability of the bubbles change with different soap concentrations.
Check out more bubble science experiments kids will love!
5. Ketchup vs. Mustard Race
Fill two squeeze bottles, one with ketchup and the other with mustard. Have the kids squeeze both bottles onto a plate and observe and discuss which condiment has a higher viscosity.
6. Molasses Pouring
Pour molasses or honey onto a plate and observe its slow flow. Discuss how molasses has a higher viscosity compared to water.
7. Dropper Races
Fill two droppers with liquids of different viscosities, such as water and honey. Challenge the kids to squeeze the droppers and observe how fast the liquids come out. Discuss the differences in flow rate.
8. Hot and Cold Syrup
Heat one container of syrup and keep another at room temperature. Compare the viscosity of the warm and cold syrup by pouring them onto a plate. Discuss how temperature can affect viscosity.
More Fun Science Experiments
- Magic Milk Experiment
- Self Inflating Balloon Experiment
- Egg in Vinegar Experiment
- Mentos and Coke Experiment
- Pop Rocks Viscosity Experiment
- Water Density Experiment
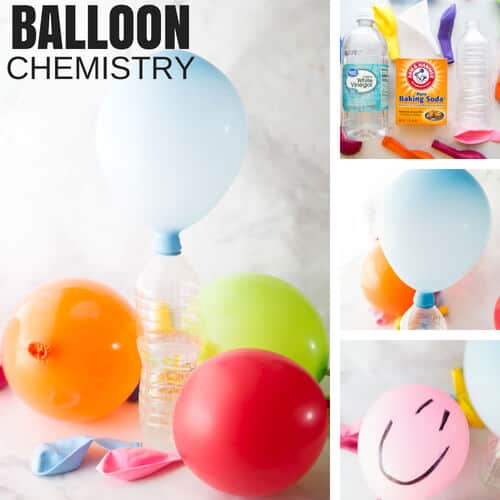
Printable Science Projects For Kids
If you’re looking to grab all of our printable science projects in one convenient place plus exclusive worksheets and bonuses like a STEAM Project pack, our Science Project Pack is what you need! Over 300+ Pages!
- 90+ classic science activities with journal pages, supply lists, set up and process, and science information. NEW! Activity-specific observation pages!
- Best science practices posters and our original science method process folders for extra alternatives!
- Be a Collector activities pack introduces kids to the world of making collections through the eyes of a scientist. What will they collect first?
- Know the Words Science vocabulary pack includes flashcards, crosswords, and word searches that illuminate keywords in the experiments!
- My science journal writing prompts explore what it means to be a scientist!!
- Bonus STEAM Project Pack: Art meets science with doable projects!
- Bonus Quick Grab Packs for Biology, Earth Science, Chemistry, and Physics

~ Projects to Try Now! ~

Module 10: Liquids and Solids
Properties of liquids, learning outcomes.
- Distinguish between adhesive and cohesive forces
- Define viscosity, surface tension, and capillary rise
- Describe the roles of intermolecular attractive forces in each of these properties/phenomena
When you pour a glass of water, or fill a car with gasoline, you observe that water and gasoline flow freely. But when you pour syrup on pancakes or add oil to a car engine, you note that syrup and motor oil do not flow as readily. The viscosity of a liquid is a measure of its resistance to flow. Water, gasoline, and other liquids that flow freely have a low viscosity. Honey, syrup, motor oil, and other liquids that do not flow freely, like those shown in Figure 1, have higher viscosities. We can measure viscosity by measuring the rate at which a metal ball falls through a liquid (the ball falls more slowly through a more viscous liquid) or by measuring the rate at which a liquid flows through a narrow tube (more viscous liquids flow more slowly).
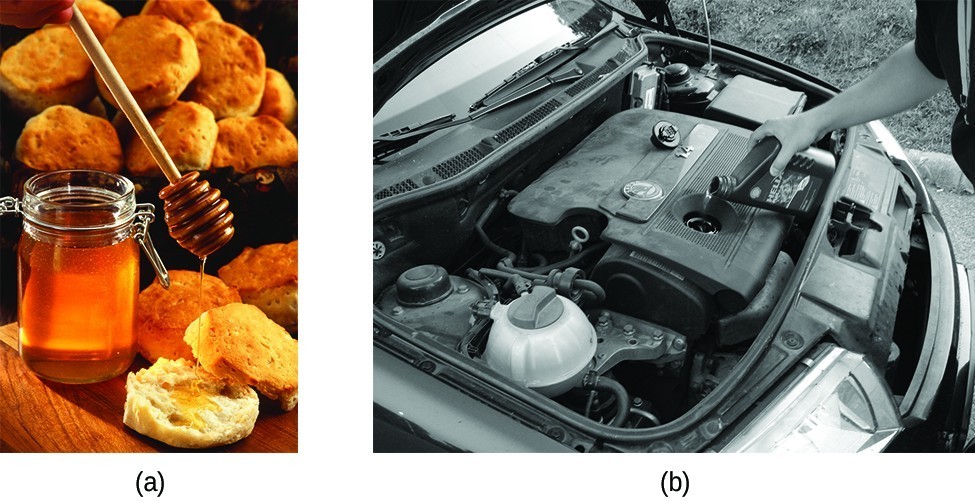
Figure 1. (a) Honey and (b) motor oil are examples of liquids with high viscosities; they flow slowly. (credit a: modification of work by Scott Bauer; credit b: modification of work by David Nagy)
You can view the transcript for “Surface tension | States of matter and intermolecular forces | Chemistry | Khan Academy” here (opens in new window) .
The IMFs between the molecules of a liquid, the size and shape of the molecules, and the temperature determine how easily a liquid flows. As Table 1 shows, the more structurally complex are the molecules in a liquid and the stronger the IMFs between them, the more difficult it is for them to move past each other and the greater is the viscosity of the liquid. As the temperature increases, the molecules move more rapidly and their kinetic energies are better able to overcome the forces that hold them together; thus, the viscosity of the liquid decreases.
The various IMFs between identical molecules of a substance are examples of cohesive forces . The molecules within a liquid are surrounded by other molecules and are attracted equally in all directions by the cohesive forces within the liquid. However, the molecules on the surface of a liquid are attracted only by about one-half as many molecules. Because of the unbalanced molecular attractions on the surface molecules, liquids contract to form a shape that minimizes the number of molecules on the surface—that is, the shape with the minimum surface area. A small drop of liquid tends to assume a spherical shape, as shown in Figure 2, because in a sphere, the ratio of surface area to volume is at a minimum. Larger drops are more greatly affected by gravity, air resistance, surface interactions, and so on, and as a result, are less spherical.
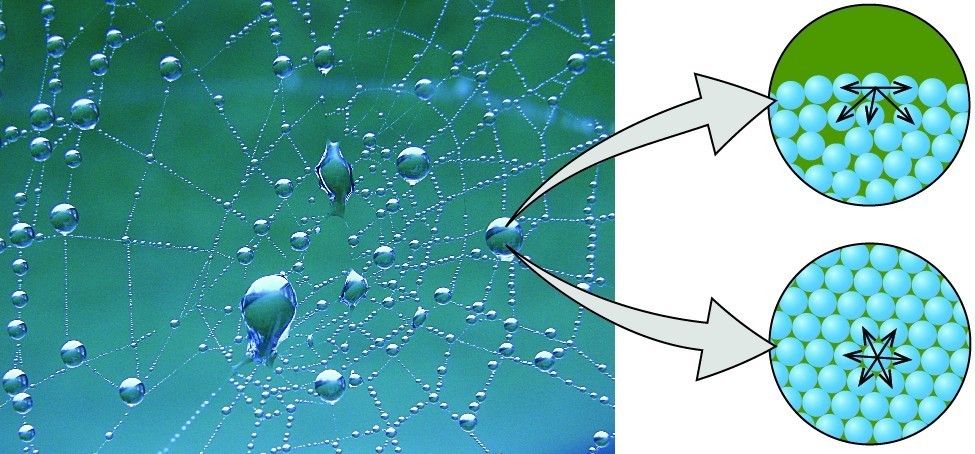
Figure 2. Attractive forces result in a spherical water drop that minimizes surface area; cohesive forces hold the sphere together; adhesive forces keep the drop attached to the web. (credit: modification of work by “OliBac”/Flickr)
Surface tension is defined as the energy required to increase the surface area of a liquid, or the force required to increase the length of a liquid surface by a given amount. This property results from the cohesive forces between molecules at the surface of a liquid, and it causes the surface of a liquid to behave like a stretched rubber membrane. Surface tensions of several liquids are presented in Table 2. Among common liquids, water exhibits a distinctly high surface tension due to strong hydrogen bonding between its molecules. As a result of this high surface tension, the surface of water represents a relatively “tough skin” that can withstand considerable force without breaking. A steel needle carefully placed on water will float. Some insects, like the one shown in Figure 3, even though they are denser than water, move on its surface because they are supported by the surface tension.
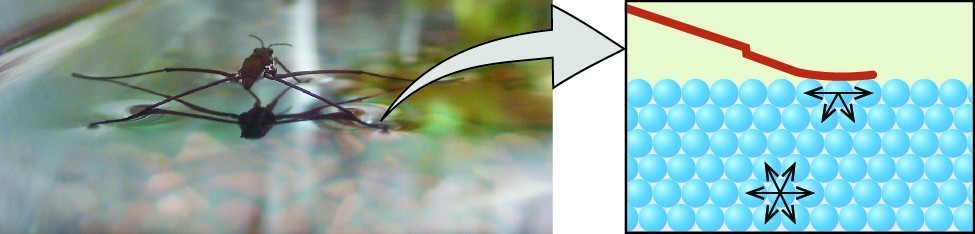
Figure 3. Surface tension (right) prevents this insect, a “water strider,” from sinking into the water (left).
The IMFs of attraction between two different molecules are called adhesive forces . Consider what happens when water comes into contact with some surface. If the adhesive forces between water molecules and the molecules of the surface are weak compared to the cohesive forces between the water molecules, the water does not “wet” the surface.
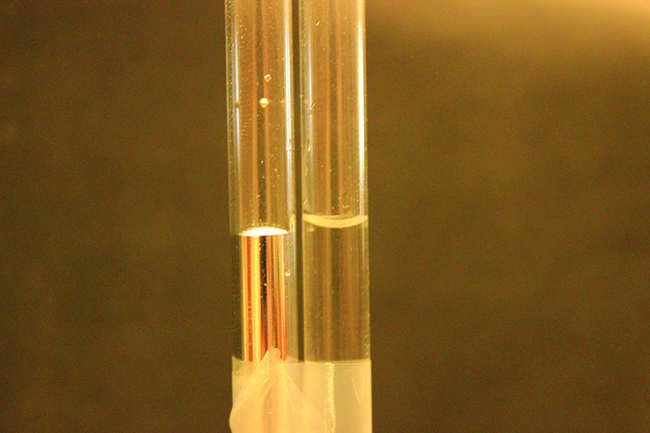
Figure 4. Differences in the relative strengths of cohesive and adhesive forces result in different meniscus shapes for mercury (left) and water (right) in glass tubes. (credit: Mark Ott)
For example, water does not wet waxed surfaces or many plastics such as polyethylene. Water forms drops on these surfaces because the cohesive forces within the drops are greater than the adhesive forces between the water and the plastic. Water spreads out on glass because the adhesive force between water and glass is greater than the cohesive forces within the water. When water is confined in a glass tube, its meniscus (surface) has a concave shape because the water wets the glass and creeps up the side of the tube. On the other hand, the cohesive forces between mercury atoms are much greater than the adhesive forces between mercury and glass. Mercury therefore does not wet glass, and it forms a convex meniscus when confined in a tube because the cohesive forces within the mercury tend to draw it into a drop (Figure 4).
If you place one end of a paper towel in spilled wine, as shown in Figure 5, the liquid wicks up the paper towel. A similar process occurs in a cloth towel when you use it to dry off after a shower. These are examples of capillary action —when a liquid flows within a porous material due to the attraction of the liquid molecules to the surface of the material and to other liquid molecules. The adhesive forces between the liquid and the porous material, combined with the cohesive forces within the liquid, may be strong enough to move the liquid upward against gravity.
Figure 5. Wine wicks up a paper towel (left) because of the strong attractions of water (and ethanol) molecules to the −OH groups on the towel’s cellulose fibers and the strong attractions of water molecules to other water (and ethanol) molecules (right). (credit photo: modification of work by Mark Blaser)
Towels soak up liquids like water because the fibers of a towel are made of molecules that are attracted to water molecules. Most cloth towels are made of cotton, and paper towels are generally made from paper pulp. Both consist of long molecules of cellulose that contain many −OH groups. Water molecules are attracted to these −OH groups and form hydrogen bonds with them, which draws the H 2 O molecules up the cellulose molecules. The water molecules are also attracted to each other, so large amounts of water are drawn up the cellulose fibers.
Capillary action can also occur when one end of a small diameter tube is immersed in a liquid, as illustrated in Figure 6. If the liquid molecules are strongly attracted to the tube molecules, the liquid creeps up the inside of the tube until the weight of the liquid and the adhesive forces are in balance. The smaller the diameter of the tube is, the higher the liquid climbs. It is partly by capillary action occurring in plant cells called xylem that water and dissolved nutrients are brought from the soil up through the roots and into a plant. Capillary action is the basis for thin layer chromatography, a laboratory technique commonly used to separate small quantities of mixtures. You depend on a constant supply of tears to keep your eyes lubricated and on capillary action to pump tear fluid away.
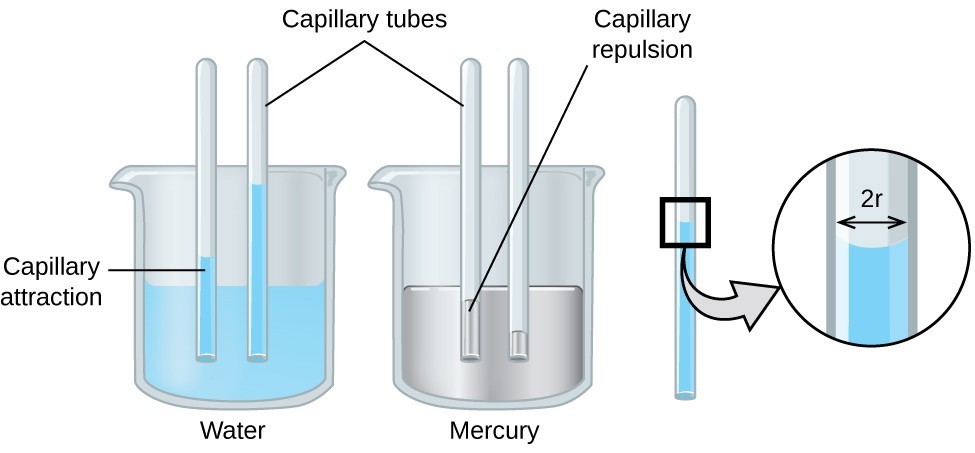
Figure 6. Depending upon the relative strengths of adhesive and cohesive forces, a liquid may rise (such as water) or fall (such as mercury) in a glass capillary tube. The extent of the rise (or fall) is directly proportional to the surface tension of the liquid and inversely proportional to the density of the liquid and the radius of the tube.
The height to which a liquid will rise in a capillary tube is determined by several factors as shown in the following equation:
[latex]\displaystyle{h=\dfrac{2T\text{cos}\theta }{r\rho g}}[/latex]
In this equation, h is the height of the liquid inside the capillary tube relative to the surface of the liquid outside the tube, T is the surface tension of the liquid, θ is the contact angle between the liquid and the tube, r is the radius of the tube, ρ is the density of the liquid, and g is the acceleration due to gravity, 9.8 m/s 2 . When the tube is made of a material to which the liquid molecules are strongly attracted, they will spread out completely on the surface, which corresponds to a contact angle of 0°. This is the situation for water rising in a glass tube.
Example 1: Capillary Rise
At 25 °C, how high will water rise in a glass capillary tube with an inner diameter of 0.25 mm?
For water, T = 71.99 mN/m and ρ = 1.0 g/cm 3 .
The liquid will rise to a height h given by: [latex]h=\dfrac{2T\text{cos}\theta }{r\rho g}[/latex]
The Newton is defined as a kg m/s 2 , and so the provided surface tension is equivalent to 0.07199 kg/s 2 . The provided density must be converted into units that will cancel appropriately: ρ = 1000 kg/m 3 . The diameter of the tube in meters is 0.00025 m, so the radius is 0.000125 m. For a glass tube immersed in water, the contact angle is θ = 0°, so cos θ = 1. Finally, acceleration due to gravity on the earth is g = 9.8 m/s 2 . Substituting these values into the equation, and cancelling units, we have:
[latex]h=\dfrac{2\left(0.07199{\text{kg/s}}^{2}\right)}{\left(0.000125\text{m}\right)\left(1000{\text{kg/m}}^{3}\right)\left(9.8{\text{m/s}}^{2}\right)}=0.12\text{m}=\text{12 cm}[/latex]
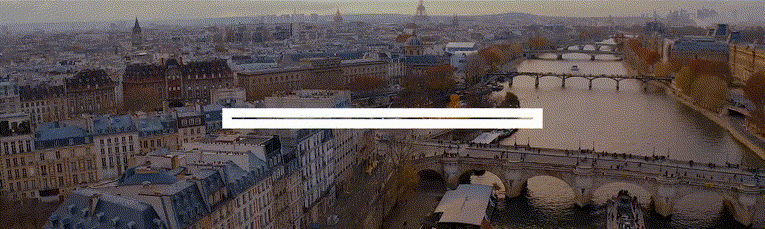
Check Your Learning
Water rises in a glass capillary tube to a height of 8.4 cm. What is the diameter of the capillary tube?
Biomedical Applications of Capillary Action
Figure 7. Blood is collected for medical analysis by capillary action, which draws blood into a small diameter glass tube. (credit: modification of work by Centers for Disease Control and Prevention)
Many medical tests require drawing a small amount of blood, for example to determine the amount of glucose in someone with diabetes or the hematocrit level in an athlete. This procedure can be easily done because of capillary action, the ability of a liquid to flow up a small tube against gravity, as shown in Figure 7. When your finger is pricked, a drop of blood forms and holds together due to surface tension—the unbalanced intermolecular attractions at the surface of the drop. Then, when the open end of a narrow-diameter glass tube touches the drop of blood, the adhesive forces between the molecules in the blood and those at the glass surface draw the blood up the tube. How far the blood goes up the tube depends on the diameter of the tube (and the type of fluid). A small tube has a relatively large surface area for a given volume of blood, which results in larger (relative) attractive forces, allowing the blood to be drawn farther up the tube. The liquid itself is held together by its own cohesive forces. When the weight of the liquid in the tube generates a downward force equal to the upward force associated with capillary action, the liquid stops rising.
You can view the transcript for “Capillary action and why we see a meniscus | Chemistry | Khan Academy” here (opens in new window) .
Key Concepts and Summary
The intermolecular forces between molecules in the liquid state vary depending upon their chemical identities and result in corresponding variations in various physical properties. Cohesive forces between like molecules are responsible for a liquid’s viscosity (resistance to flow) and surface tension (elasticity of a liquid surface). Adhesive forces between the molecules of a liquid and different molecules composing a surface in contact with the liquid are responsible for phenomena such as surface wetting and capillary rise.
Key Equations
- [latex]h=\dfrac{2T\text{cos}\theta }{r\rho g}[/latex]
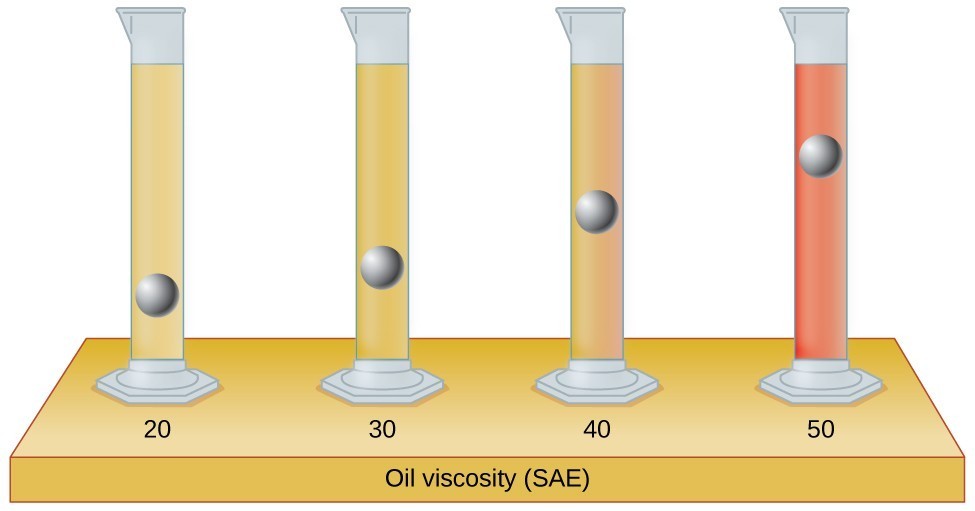
- Explain their differences in viscosity in terms of the size and shape of their molecules and their IMFs.
- Explain their differences in surface tension in terms of the size and shape of their molecules and their IMFs.
- You may have heard someone use the figure of speech “slower than molasses in winter” to describe a process that occurs slowly. Explain why this is an apt idiom, using concepts of molecular size and shape, molecular interactions, and the effect of changing temperature.
- It is often recommended that you let your car engine run idle to warm up before driving, especially on cold winter days. While the benefit of prolonged idling is dubious, it is certainly true that a warm engine is more fuel efficient than a cold one. Explain the reason for this.
- As temperature increases, what happens to the surface tension of water? Explain why this occurs, in terms of molecular interactions and the effect of changing temperature.
- As temperature increases, what happens to the viscosity of water? Explain why this occurs, in terms of molecular interactions and the effect of changing temperature.
- At 25 °C, how high will water rise in a glass capillary tube with an inner diameter of 0.63 mm? Refer to Example 10.4 for the required information.
- Water rises in a glass capillary tube to a height of 17 cm. What is the diameter of the capillary tube?
2. The water molecules have strong intermolecular forces of hydrogen bonding. The water molecules are thus attracted strongly to one another and exhibit a relatively large surface tension, forming a type of “skin” at its surface. This skin can support a bug or paper clip if gently placed on the water.
4. Temperature has an effect on intermolecular forces: the higher the temperature, the greater the kinetic energies of the molecules and the greater the extent to which their intermolecular forces are overcome, and so the more fluid (less viscous) the liquid; the lower the temperature, the lesser the intermolecular forces are overcome, and so the less viscous the liquid.
6. (a) As the water reaches higher temperatures, the increased kinetic energies of its molecules are more effective in overcoming hydrogen bonding, and so its surface tension decreases. Surface tension and intermolecular forces are directly related.
(b) The same trend in viscosity is seen as in surface tension, and for the same reason.
8. This time we will solve for r , as we are given h = 17 cm = 0.17 m.
[latex]\begin{array}{rll}0.17\text{m}&=&\frac{2\left(0.0799{\text{kg/s}}^{2}\right)}{r\left(1000{\text{kg/m}}^{2}\right)\left({\text{9.8 m/s}}^{2}\right)}\\ r&=&9.5\times {10}^{-5}\text{m}\end{array}[/latex]
adhesive force: force of attraction between molecules of different chemical identities
capillary action: flow of liquid within a porous material due to the attraction of the liquid molecules to the surface of the material and to other liquid molecules
cohesive force: force of attraction between identical molecules
surface tension: energy required to increase the area, or length, of a liquid surface by a given amount
viscosity: measure of a liquid’s resistance to flow
- Chemistry 2e. Provided by : OpenStax. Located at : https://openstax.org/ . License : CC BY: Attribution . License Terms : Access for free at https://openstax.org/books/chemistry-2e/pages/1-introduction
- Surface tension | States of matter and intermolecular forces | Chemistry | Khan Academy. Authored by : Khan Academy. Located at : https://youtu.be/_RTF0DAHBBM . License : Other . License Terms : Standard YouTube License
- Capillary action and why we see a meniscus | Chemistry | Khan Academy. Authored by : Khan Academy. Located at : https://youtu.be/eQXGpturk3A . License : Other . License Terms : Standard YouTube License

An official website of the United States government
Here’s how you know
Official websites use .gov A .gov website belongs to an official government organization in the United States.
Secure .gov websites use HTTPS A lock ( Lock A locked padlock ) or https:// means you’ve safely connected to the .gov website. Share sensitive information only on official, secure websites.
Liquids Rule
You are allowed to bring a quart-sized bag of liquids, aerosols, gels, creams and pastes in your carry-on bag and through the checkpoint. These are limited to travel-sized containers that are 3.4 ounces (100 milliliters) or less per item. Placing these items in the small bag and separating from your carry-on baggage facilitates the screening process. Pack items that are in containers larger than 3.4 ounces or 100 milliliters in checked baggage.
Any liquid, aerosol, gel, cream or paste that alarms during screening will require additional screening.
- Medications
- Infant and child nourishments
Inbound International Flights
You may carry duty free liquids in secure, tamper–evident bags, more than 3.4 oz or 100 ml in your carry-on bag if:
- The duty free liquids were purchased internationally and you are traveling to the United States with a connecting flight.
- The liquids are packed in a transparent, secure, tamper-evident bag by the retailer and do not show signs of tampering when presented to TSA for screening.
- The original receipt for the liquids is present and the purchase was made within 48 hours.
The items inside the secure, tamper-evident bags must be screened and cleared. Any item that alarms or is unable to be screened will not be permitted in your carry-on bag. We recommend packing all liquids, gels, and aerosols that are over 3.4 oz or 100 ml in your checked baggage, even if they are in a secure, tamper-evident bag.
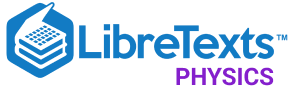
- school Campus Bookshelves
- menu_book Bookshelves
- perm_media Learning Objects
- login Login
- how_to_reg Request Instructor Account
- hub Instructor Commons
- Download Page (PDF)
- Download Full Book (PDF)
- Periodic Table
- Physics Constants
- Scientific Calculator
- Reference & Cite
- Tools expand_more
- Readability
selected template will load here
This action is not available.
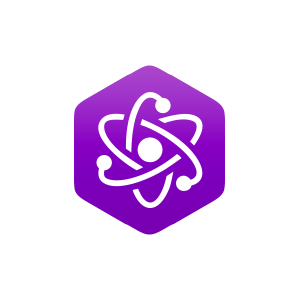
13.2: Traveling Waves
- Last updated
- Save as PDF
- Page ID 68849
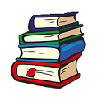
Learning Objectives
- Describe the basic characteristics of wave motion
- Define the terms wavelength, amplitude, period, frequency, and wave speed
- Explain the difference between longitudinal and transverse waves, and give examples of each type
- List the different types of waves
We saw in Oscillations that oscillatory motion is an important type of behavior that can be used to model a wide range of physical phenomena. Oscillatory motion is also important because oscillations can generate waves, which are of fundamental importance in physics. Many of the terms and equations we studied in the chapter on oscillations apply equally well to wave motion (Figure \(\PageIndex{1}\)).
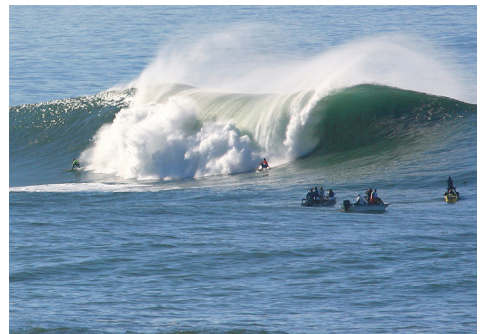
Types of Waves
A wave is a disturbance that propagates, or moves from the place it was created. There are three basic types of waves: mechanical waves, electromagnetic waves, and matter waves.
Basic mechanical waves are governed by Newton’s laws and require a medium. A medium is the substance a mechanical waves propagates through, and the medium produces an elastic restoring force when it is deformed. Mechanical waves transfer energy and momentum, without transferring mass. Some examples of mechanical waves are water waves, sound waves, and seismic waves. The medium for water waves is water; for sound waves, the medium is usually air. (Sound waves can travel in other media as well; we will look at that in more detail in Sound .) For surface water waves, the disturbance occurs on the surface of the water, perhaps created by a rock thrown into a pond or by a swimmer splashing the surface repeatedly. For sound waves, the disturbance is a change in air pressure, perhaps created by the oscillating cone inside a speaker or a vibrating tuning fork. In both cases, the disturbance is the oscillation of the molecules of the fluid. In mechanical waves, energy and momentum transfer with the motion of the wave, whereas the mass oscillates around an equilibrium point. (We discuss this in Energy and Power of a Wave .) Earthquakes generate seismic waves from several types of disturbances, including the disturbance of Earth’s surface and pressure disturbances under the surface. Seismic waves travel through the solids and liquids that form Earth. In this chapter, we focus on mechanical waves.
Electromagnetic waves are associated with oscillations in electric and magnetic fields and do not require a medium. Examples include gamma rays, X-rays, ultraviolet waves, visible light, infrared waves, microwaves, and radio waves. Electromagnetic waves can travel through a vacuum at the speed of light, v = c = 2.99792458 x 10 8 m/s. For example, light from distant stars travels through the vacuum of space and reaches Earth. Electromagnetic waves have some characteristics that are similar to mechanical waves; they are covered in more detail in Electromagnetic Waves .
Matter waves are a central part of the branch of physics known as quantum mechanics. These waves are associated with protons, electrons, neutrons, and other fundamental particles found in nature. The theory that all types of matter have wavelike properties was first proposed by Louis de Broglie in 1924. Matter waves are discussed in Photons and Matter Waves .
Mechanical Waves
Mechanical waves exhibit characteristics common to all waves, such as amplitude, wavelength, period, frequency, and energy. All wave characteristics can be described by a small set of underlying principles.
The simplest mechanical waves repeat themselves for several cycles and are associated with simple harmonic motion. These simple harmonic waves can be modeled using some combination of sine and cosine functions. For example, consider the simplified surface water wave that moves across the surface of water as illustrated in Figure \(\PageIndex{2}\). Unlike complex ocean waves, in surface water waves, the medium, in this case water, moves vertically, oscillating up and down, whereas the disturbance of the wave moves horizontally through the medium. In Figure \(\PageIndex{2}\), the waves causes a seagull to move up and down in simple harmonic motion as the wave crests and troughs (peaks and valleys) pass under the bird. The crest is the highest point of the wave, and the trough is the lowest part of the wave. The time for one complete oscillation of the up-and-down motion is the wave’s period T. The wave’s frequency is the number of waves that pass through a point per unit time and is equal to f = \(\frac{1}{T}\). The period can be expressed using any convenient unit of time but is usually measured in seconds; frequency is usually measured in hertz (Hz), where 1 Hz = 1 s −1 .
The length of the wave is called the wavelength and is represented by the Greek letter lambda (\(\lambda\)), which is measured in any convenient unit of length, such as a centimeter or meter. The wavelength can be measured between any two similar points along the medium that have the same height and the same slope. In Figure \(\PageIndex{2}\), the wavelength is shown measured between two crests. As stated above, the period of the wave is equal to the time for one oscillation, but it is also equal to the time for one wavelength to pass through a point along the wave’s path.
The amplitude of the wave (A) is a measure of the maximum displacement of the medium from its equilibrium position. In the figure, the equilibrium position is indicated by the dotted line, which is the height of the water if there were no waves moving through it. In this case, the wave is symmetrical, the crest of the wave is a distance +A above the equilibrium position, and the trough is a distance −A below the equilibrium position. The units for the amplitude can be centimeters or meters, or any convenient unit of distance.
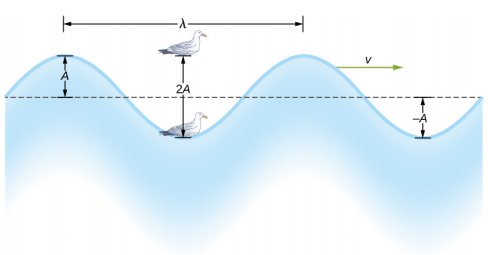
The water wave in the figure moves through the medium with a propagation velocity \(\vec{v}\). The magnitude of the wave velocity is the distance the wave travels in a given time, which is one wavelength in the time of one period, and the wave speed is the magnitude of wave velocity. In equation form, this is
\[v = \frac{\lambda}{T} = \lambda f \ldotp \label{16.1}\]
This fundamental relationship holds for all types of waves. For water waves, v is the speed of a surface wave; for sound, v is the speed of sound; and for visible light, v is the speed of light.
Transverse and Longitudinal Waves
We have seen that a simple mechanical wave consists of a periodic disturbance that propagates from one place to another through a medium. In Figure \(\PageIndex{3}\)(a), the wave propagates in the horizontal direction, whereas the medium is disturbed in the vertical direction. Such a wave is called a transverse wave . In a transverse wave, the wave may propagate in any direction, but the disturbance of the medium is perpendicular to the direction of propagation. In contrast, in a longitudinal wave or compressional wave, the disturbance is parallel to the direction of propagation. Figure \(\PageIndex{3}\)(b) shows an example of a longitudinal wave. The size of the disturbance is its amplitude A and is completely independent of the speed of propagation v.
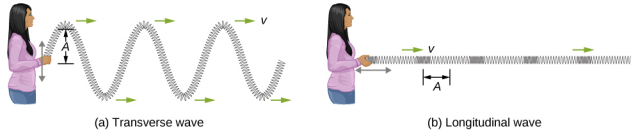
A simple graphical representation of a section of the spring shown in Figure \(\PageIndex{3}\)(b) is shown in Figure \(\PageIndex{4}\). Figure \(\PageIndex{4}\)(a) shows the equilibrium position of the spring before any waves move down it. A point on the spring is marked with a blue dot. Figure \(\PageIndex{4}\)(b) through (g) show snapshots of the spring taken one-quarter of a period apart, sometime after the end of` the spring is oscillated back and forth in the x-direction at a constant frequency. The disturbance of the wave is seen as the compressions and the expansions of the spring. Note that the blue dot oscillates around its equilibrium position a distance A, as the longitudinal wave moves in the positive x-direction with a constant speed. The distance A is the amplitude of the wave. The y-position of the dot does not change as the wave moves through the spring. The wavelength of the wave is measured in part (d). The wavelength depends on the speed of the wave and the frequency of the driving force.
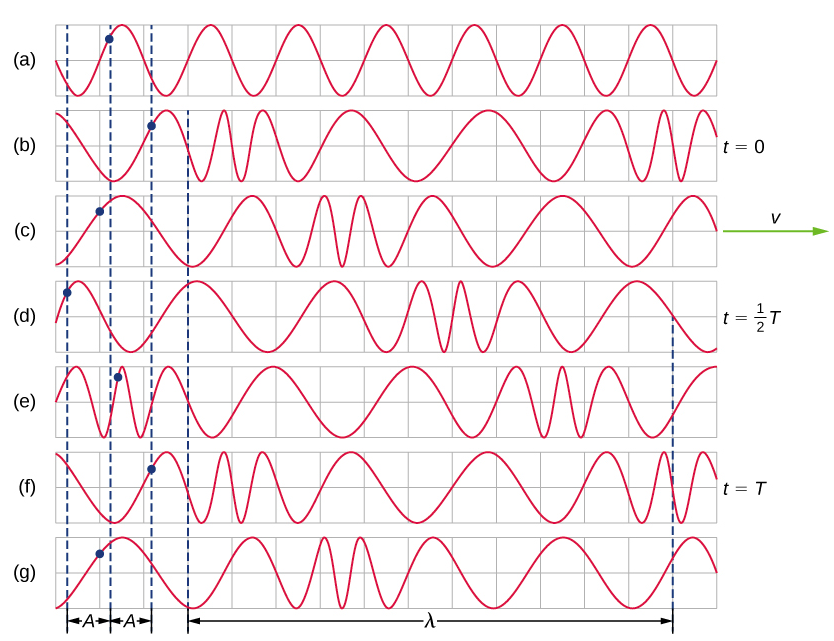
Waves may be transverse, longitudinal, or a combination of the two. Examples of transverse waves are the waves on stringed instruments or surface waves on water, such as ripples moving on a pond. Sound waves in air and water are longitudinal. With sound waves, the disturbances are periodic variations in pressure that are transmitted in fluids. Fluids do not have appreciable shear strength, and for this reason, the sound waves in them are longitudinal waves. Sound in solids can have both longitudinal and transverse components, such as those in a seismic wave. Earthquakes generate seismic waves under Earth’s surface with both longitudinal and transverse components (called compressional or P-waves and shear or S-waves, respectively). The components of seismic waves have important individual characteristics—they propagate at different speeds, for example. Earthquakes also have surface waves that are similar to surface waves on water. Ocean waves also have both transverse and longitudinal components.
Example 16.1: Wave on a String
A student takes a 30.00-m-long string and attaches one end to the wall in the physics lab. The student then holds the free end of the rope, keeping the tension constant in the rope. The student then begins to send waves down the string by moving the end of the string up and down with a frequency of 2.00 Hz. The maximum displacement of the end of the string is 20.00 cm. The first wave hits the lab wall 6.00 s after it was created. (a) What is the speed of the wave? (b) What is the period of the wave? (c) What is the wavelength of the wave?
- The speed of the wave can be derived by dividing the distance traveled by the time.
- The period of the wave is the inverse of the frequency of the driving force.
- The wavelength can be found from the speed and the period v = \(\frac{\lambda}{T}\).
- The first wave traveled 30.00 m in 6.00 s: $v = \frac{30.00\; m}{6.00\; s} = 5.00\; m/s \ldotp$
- . The period is equal to the inverse of the frequency: $T = \frac{1}{f} = \frac{1}{2.00\; s^{-1}} = 0.50\; s \ldotp$
- The wavelength is equal to the velocity times the period: $\lambda = vT = (5.00\; m/s)(0.50\; s) = 2.50\; m \ldotp$
Significance
The frequency of the wave produced by an oscillating driving force is equal to the frequency of the driving force.
Exercise 16.1
g When a guitar string is plucked, the guitar string oscillates as a result of waves moving through the string. The vibrations of the string cause the air molecules to oscillate, forming sound waves. The frequency of the sound waves is equal to the frequency of the vibrating string. Is the wavelength of the sound wave always equal to the wavelength of the waves on the string?
Example 16.2: Characteristics of a Wave
A transverse mechanical wave propagates in the positive x-direction through a spring (as shown in Figure \(\PageIndex{3}\)(a)) with a constant wave speed, and the medium oscillates between +A and −A around an equilibrium position. The graph in Figure \(\PageIndex{5}\) shows the height of the spring (y) versus the position (x), where the xaxis points in the direction of propagation. The figure shows the height of the spring versus the x-position at t = 0.00 s as a dotted line and the wave at t = 3.00 s as a solid line. (a) Determine the wavelength and amplitude of the wave. (b) Find the propagation velocity of the wave. (c) Calculate the period and frequency of the wave.
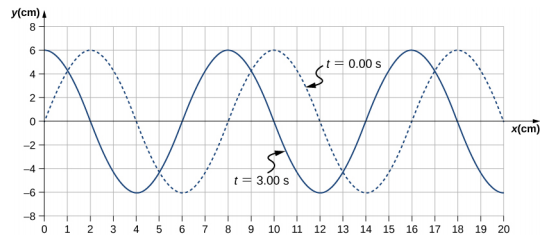
- The amplitude and wavelength can be determined from the graph.
- Since the velocity is constant, the velocity of the wave can be found by dividing the distance traveled by the wave by the time it took the wave to travel the distance.
- The period can be found from v = \(\frac{\lambda}{T}\) and the frequency from f = \(\frac{1}{T}\).
- Read the wavelength from the graph, looking at the purple arrow in Figure \(\PageIndex{6}\). Read the amplitude by looking at the green arrow. The wavelength is \(\lambda\) = 8.00 cm and the amplitude is A = 6.00 cm.
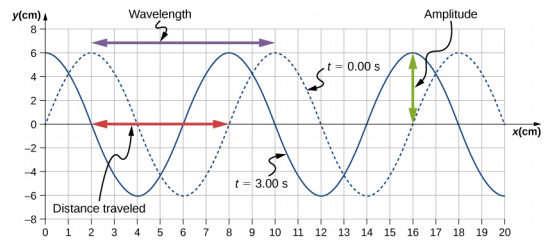
- The distance the wave traveled from time t = 0.00 s to time t = 3.00 s can be seen in the graph. Consider the red arrow, which shows the distance the crest has moved in 3 s. The distance is 8.00 cm − 2.00 cm = 6.00 cm. The velocity is $v = \frac{\Delta x}{\Delta t} = \frac{8.00\; cm - 2.00\; cm}{3.00\; s - 0.00\; s} = 2.00\; cm/s \ldotp$
- The period is T = \(\frac{\lambda}{v}\) = \(\frac{8.00\; cm}{2.00\; cm/s}\) = 4.00\; s and the frequency is f = \(\frac{1}{T}\) = \(\frac{1}{4.00\; s}\) = 0.25 Hz.
Note that the wavelength can be found using any two successive identical points that repeat, having the same height and slope. You should choose two points that are most convenient. The displacement can also be found using any convenient point.
Exercise 16.2
The propagation velocity of a transverse or longitudinal mechanical wave may be constant as the wave disturbance moves through the medium. Consider a transverse mechanical wave: Is the velocity of the medium also constant?
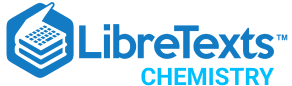
- school Campus Bookshelves
- menu_book Bookshelves
- perm_media Learning Objects
- login Login
- how_to_reg Request Instructor Account
- hub Instructor Commons
- Download Page (PDF)
- Download Full Book (PDF)
- Periodic Table
- Physics Constants
- Scientific Calculator
- Reference & Cite
- Tools expand_more
- Readability
selected template will load here
This action is not available.
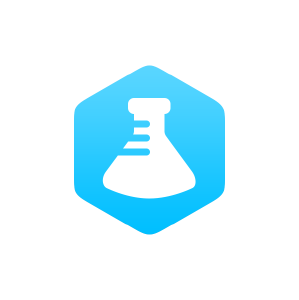
14.5: Vapor Pressure
- Last updated
- Save as PDF
- Page ID 465599
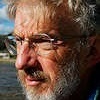
- Truro School in Cornwall
This page looks at how the equilibrium between a liquid (or a solid) and its vapor leads to the idea of a saturated vapor pressure. It also looks at how saturated vapor pressure varies with temperature, and the relationship between saturated vapor pressure and boiling point.
Evaporation: Liquid/Vapor Equilibrium
The average energy of the particles in a liquid is governed by the temperature. The higher the temperature, the higher the average energy. But within that average, some particles have energies higher than the average, and others have energies lower than the average. Some of the more energetic particles on the surface of the liquid can be moving fast enough to escape from the attractive forces holding the liquid together. They evaporate. The diagram shows a small region of a liquid near its surface.
Notice that evaporation only takes place on the surface of the liquid. That's quite different from boiling which happens when there is enough energy to disrupt the attractive forces throughout the liquid. That's why, if you look at boiling water, you see bubbles of gas being formed all the way through the liquid.
If you look at water which is just evaporating in the sun, you don't see any bubbles. Water molecules are simply breaking away from the surface layer. Eventually, the water will all evaporate in this way. The energy which is lost as the particles evaporate is replaced from the surroundings. As the molecules in the water jostle with each other, new molecules will gain enough energy to escape from the surface.
The evaporation of a liquid in a closed container
Now imagine what happens if the liquid is in a closed container. Common sense tells you that water in a sealed bottle does not seem to evaporate - or at least, it does not disappear over time. But there is constant evaporation from the surface. Particles continue to break away from the surface of the liquid - but this time they are trapped in the space above the liquid.
As the gaseous particles bounce around, some of them will hit the surface of the liquid again, and be trapped there. There will rapidly be an equilibrium set up in which the number of particles leaving the surface is exactly balanced by the number rejoining it.
In this equilibrium, there will be a fixed number of the gaseous particles in the space above the liquid. When these particles hit the walls of the container, they exert a pressure. This pressure is called the saturated vapor pressure (also known as saturation vapor pressure ) of the liquid.
Measuring the saturated vapor pressure
It is not difficult to show the existence of this saturated vapor pressure (and to measure it) using a simple piece of apparatus. If you have a mercury barometer tube in a trough of mercury, at 1 atmosphere pressure the column will be 760 mm tall. 1 atmosphere is sometimes quoted as 760 mmHg ("millimetres of mercury").
If you squirt a few drops of liquid into the tube, it will rise to form a thin layer floating on top of the mercury. Some of the liquid will evaporate and you will get the equilibrium we've just been talking about - provided there is still some liquid on top of the mercury. It is only an equilibrium if both liquid and vapor are present.
The saturated vapor pressure of the liquid will force the mercury level down a bit. You can measure the drop - and this gives a value for the saturated vapor pressure of the liquid at this temperature. In this case, the mercury has been forced down by a distance of 760 - 630 mm. The saturated vapor pressure of this liquid at the temperature of the experiment is 130 mmHg. You could convert this into proper SI units (pascals) if you wanted to. 760 mmHg is equivalent to 101,325 Pa.
A value of 130 mmHg is quite a high vapor pressure if we are talking about room temperature. Water's saturated vapor pressure is about 20 mmHg at this temperature. A high vapor pressure means that the liquid must be volatile - molecules escape from its surface relatively easily, and aren't very good at sticking back on again either.
That will result in larger numbers of them in the gas state once equilibrium is reached. The liquid in the example must have significantly weaker intermolecular forces than water.
The variation of saturated vapor pressure with temperature
The effect of temperature liquid/ vapor equilibrium.
You can look at this in two ways. (1) There is a common sense way. If you increase the temperature, you are increasing the average energy of the particles present. That means that more of them are likely to have enough energy to escape from the surface of the liquid. That will tend to increase the saturated vapor pressure. (2) Or you can look at it in terms of Le Chatelier's Principle - which works just as well in this kind of physical situation as it does in the more familiar chemical examples.
When the space above the liquid is saturated with vapor particles, you have this equilibrium occurring on the surface of the liquid:
The forward change (liquid to vapor) is endothermic. It needs heat to convert the liquid into the vapor. According to Le Chatelier, increasing the temperature of a system in a dynamic equilibrium favors the endothermic change. That means that increasing the temperature increases the amount of vapor present, and so increases the saturated vapor pressure.
The effect of temperature on the saturated vapor pressure of water
The graph shows how the saturated vapor pressure (svp) of water varies from 0°C to 100 °C. The pressure scale (the vertical one) is measured in kilopascals (kPa). 1 atmosphere pressure is 101.325 kPa.
Saturated vapor pressure and boiling point
A liquid boils when its saturated vapor pressure becomes equal to the external pressure on the liquid. When that happens, it enables bubbles of vapor to form throughout the liquid - those are the bubbles you see when a liquid boils.
If the external pressure is higher than the saturated vapor pressure, these bubbles are prevented from forming, and you just get evaporation at the surface of the liquid. If the liquid is in an open container and exposed to normal atmospheric pressure, the liquid boils when its saturated vapor pressure becomes equal to 1 atmosphere (or 101325 Pa or 101.325 kPa or 760 mmHg). This happens with water when the temperature reaches 100°C.
But at different pressures, water will boil at different temperatures. For example, at the top of Mount Everest the pressure is so low that water will boil at about 70°C. Whenever we just talk about "the boiling point" of a liquid, we always assume that it is being measured at exactly 1 atmosphere pressure. In practice, of course, that is rarely exactly true.
Sublimation: solid/vapor Equilibrium
Solids can also lose particles from their surface to form a vapor, except that in this case we call the effect sublimation rather than evaporation. Sublimation is the direct change from solid to vapor (or vice versa) without going through the liquid stage.
In most cases, at ordinary temperatures, the saturated vapor pressures of solids range from low to very, very, very low. The forces of attraction in many solids are too high to allow much loss of particles from the surface. However, there are some which do easily form vapors. For example, naphthalene (used in old-fashioned "moth balls" to deter clothes moths) has quite a strong smell. Molecules must be breaking away from the surface as a vapor, because otherwise you would not be able to smell it. Another fairly common example (discussed in detail on another page) is solid carbon dioxide - "dry ice". This never forms a liquid at atmospheric pressure and always converts directly from solid to vapor. That's why it is known as dry ice.
Contributors and Attributions
Jim Clark ( Chemguide.co.uk )
FREE K-12 standards-aligned STEM
curriculum for educators everywhere!
Find more at TeachEngineering.org .
- TeachEngineering
- Traveling Sound
Hands-on Activity Traveling Sound
Grade Level: 4 (3-5)
Time Required: 30 minutes
Expendable Cost/Group: US $2.00
Group Size: 2
Activity Dependency: None
Subject Areas: Physical Science, Reasoning and Proof, Science and Technology
NGSS Performance Expectations:
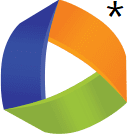
Curriculum in this Unit Units serve as guides to a particular content or subject area. Nested under units are lessons (in purple) and hands-on activities (in blue). Note that not all lessons and activities will exist under a unit, and instead may exist as "standalone" curriculum.
- Seeing and Feeling Sound Vibrations
- Pitch and Frequency
- Sound Visualization Stations
TE Newsletter
Engineering connection, learning objectives, materials list, worksheets and attachments, more curriculum like this, pre-req knowledge, introduction/motivation, vocabulary/definitions, troubleshooting tips, activity extensions, activity scaling, user comments & tips.
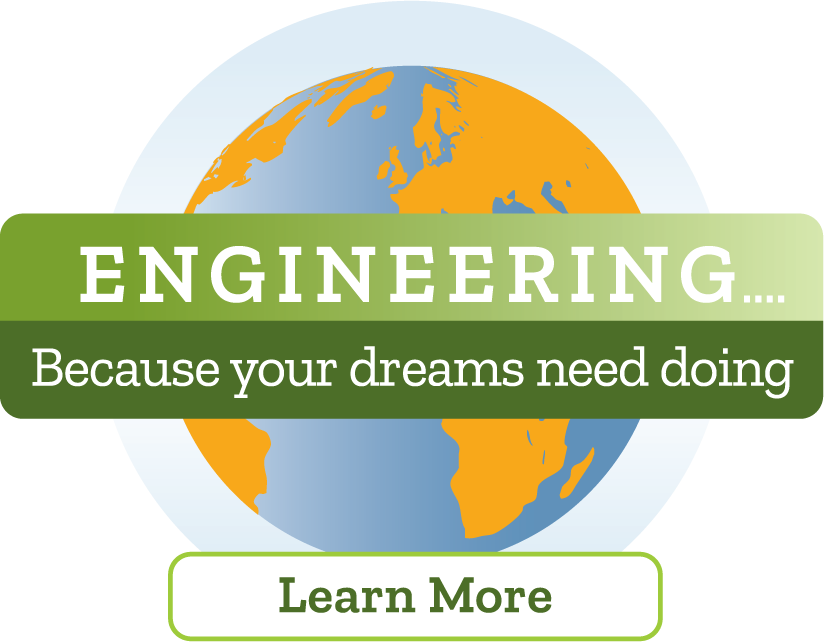
Sound and acoustic engineers know that the shape of a room and its materials greatly impact how sound waves travel. Recording studios are designed in soundproof booths so that the recorded music does not contain any unwanted external noise. Libraries are designed to reduce any introduced noises, to assure a quiet, non-distracting learning environment. Concert halls are designed so that sound generated on the stage travels to the back of the space without being distorted.
After this activity, students should be able to:
- Explain that sound can move through solids, liquids and gases.
- Describe how sound needs molecules to move and that changing the medium that it travels through changes the sound.
- Describe how engineers use sound energy when designing spaces, such as movie theaters.
Educational Standards Each TeachEngineering lesson or activity is correlated to one or more K-12 science, technology, engineering or math (STEM) educational standards. All 100,000+ K-12 STEM standards covered in TeachEngineering are collected, maintained and packaged by the Achievement Standards Network (ASN) , a project of D2L (www.achievementstandards.org). In the ASN, standards are hierarchically structured: first by source; e.g. , by state; within source by type; e.g. , science or mathematics; within type by subtype, then by grade, etc .
Ngss: next generation science standards - science, international technology and engineering educators association - technology.
View aligned curriculum
Do you agree with this alignment? Thanks for your feedback!
State Standards
Colorado - science.
Each group needs:
- large bowl (metal works best)
- 2 metal objects, such as spoons, to knock together
- Traveling Sound Worksheet , one per student
A basic understanding of the phases of matter: liquids, solids and gases.
Sound engineers are especially interested in the way sound travels. Can you hear as well when you sit in the back of the class as when you sit in the front? What about in the assembly hall or gymnasium? On the playground? Can you think of other times when you cannot hear as well as someone else? What happened? How about in a movie theater? What do engineers do so that the sound quality is good for everyone in a movie theater? (Possible answers: Add speakers around the room, curtains, carpet the walls, cone-shaped theaters act like a megaphone and help to direct sound waves further.)
Which is louder—walking on carpet or on tile? It is quieter on carpet because the carpet absorbs the sound energy . Sound energy, light energy and other types of energy, need molecules to travel through and vibrate , but sometimes sound energy is absorbed by an object or material. Engineers use this idea when designing rooms that are meant to be quiet. Have you ever noticed how the walls of a movie theater are covered with carpet or fabric? This is to prevent echoing of the sound system. Sometimes when you are in an empty room, your voice echoes or sounds hollow. This is because an empty room has no materials in it that might absorb the sound energy, so the sound bounces off the hard walls, back at you. This makes it hard to hear clearly.
Do you think sound energy can travel through air? Of course it can! That is how sound energy travels when you talk to a friend. How about water? Can you hear sound travel under water? How about a solid? Can sound move through a solid object? Engineers want to know if sound can travel through solids, liquids and gases so they can develop ways to send messages to people all over the world. Can you imagine how great sound would be if it could travel anywhere?
Understanding the properties of sound and how sound waves travel helps engineers determine the best room shape and construction materials when designing libraries, classrooms, sound recording studios, concert halls and theatres. Room shape and materials can impact how sound waves travel since sound waves bounce off different object in different ways. In this activity, we are going to study how sound waves travel through liquids, solids and gases, and think about how engineers might use this information.
Before the Activity
- Gather materials and make copies of the Traveling Sound Worksheet .
- Divide the class into teams of two students each.
With the Students
- Ask the students to predict if sound can move through solids, liquids and gases.
- Have the students complete the worksheet, which leads them through traveling sound wave activities.
- Can sound energy travel through solids? Students place their ears on a desk or table as they tap or scratch on the top. They compare that to the same sound made when their ear is not pressed to the table.
- Can sound energy traveling through liquids? Fill a large bowl or bucket (metal works best) with water. One student taps two spoons together under the water. Two other students observe and compare the tapping sound they hear, as heard through the air and as heard by placing an ear against the bowl.
- Can sound energy traveling through gases (air)? The students feel their throats gently during each of these tasks:
- Hum with your mouth and nose open.
- Hum with your mouth open and nose closed.
- Hum with your mouth closed and nose open.
- Hum with your mouth and nose closed.
- Discuss with the students what happened. Were their predictions correct? Can sound travel through air, water and solids? (Answer: Yes!) Sound needs molecules to move. Solids, liquids and gases are all made of molecules. The characteristics of the molecules (for example, the space between the molecules) determine whether the sound becomes muffled or changes in some way.
- How might engineers use the knowledge that sound travels through solids, liquids and gases? (Possible answers: Engineers create devices that send sound anywhere — through water to a submarine in the ocean, through wires to your TV, and through the air in surround sound movie theaters or emergency broadcast signals.)
echo: Repetition of a sound by reflection of sound waves from a surface.
frequency: The rate of vibrations in different pitches.
pitch: The highness or lowness of a sound.
sound energy: Audible energy that is released when you talk, play musical instruments or slam a door.
sound wave: A longitudinal pressure wave of audible or inaudible sound.
vibration: When something moves back and forth, it is said to vibrate. Sound is made by vibrations that are usually too fast to see.
volume: When sound becomes louder or softer.
wave: A disturbance that travels through a medium, such as air or water.
Pre-Activity Assessment
Prediction: Ask students if they think sound can move through solid, liquid, and gas. If so what are some examples? (Possible examples: Students may recall talking under water or using tin can and string telephones.)
Activity Embedded Assessment
Worksheet: Have students use the Traveling Sounds Worksheet to guide them in the activity and as a place to record their observations. Review their answers to gauge their mastery of the subject.
Post-Activity Assessment
Toss-a-Question: Ask students to independently think of an answer to the question below and write it on a half sheet of paper. Have students wad up and toss the paper to another team member who then adds their answer idea. After all students have written down ideas, have them toss the paper wad to another team, who reads the answers aloud to the class. Discuss answers with the class.
- What is an example of something through which sound can travel?
Neighbor Check: Have the students compare their activity observations with a neighbor. Are they the same or different? Have each team report some of their similar and dissimilar observations to the rest of the class.
Engineering Design: The supply of air on Earth is running out! Several futuristic cities for human habitation are being designed either underwater or deep inside mountains. Have each student group become a city planning engineering team and draw a communication system for sending emergency messages between the new cities. Make sure to illustrate and describe how the sound energy (message) will move through air, water or solid rock.
This activity can be very loud. Ask students to not disturb others while they learn and have fun.
To bring some humor to the activity, ask each student to hum a small part of their favorite song while feeling their throat. Have each student alternate between having their nose and mouth open or closed while humming non-stop. Why does the sound change depending on whether you close your nose or mouth? What happens if you block your ears? What does this activity teach us about sound? (Answer: Sound vibrations must travel through air for us to hear them. Like a musical instrument [perhaps a recorder or flute], if you change the holes where sound escapes, it changes the pitch, but not the frequency/vibrations of the sound.)
If a metal bowl is used during the activity, the vibrations from the objects colliding underwater vibrate the bowl, creating the illusion that the bowl is being struck. Have students draw the vibrations in the bowl on a piece of paper. Do the vibrations change if the objects are tapped together increasing softly?
Have students think about different forms of communications. Does sound travel most often through solids, liquids or gases? Have students poll their friends, family and neighbors to solicit their ideas.
For lower grades, conduct the activities as a class instead of in teams. Younger students could also draw pictures of their observations instead of writing in sentence form.

Students are introduced to the sound environment as an important aspect of a room or building. Several examples of acoustical engineering design for varied environments are presented.

Students learn how different materials reflect and absorb sound.

Students learn that sound is energy and has the ability to do work. Students discover that sound is produced by a vibration and they observe soundwaves and how they travel through mediums. They understand that sound can be absorbed, reflected or transmitted.
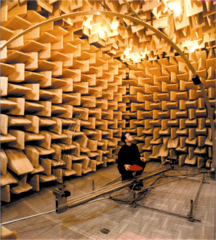
Students use the engineering design process to design and create soundproof rooms that use only one type of material. They learn and explore about how these different materials react to sound by absorbing or reflecting sound and then test their theories using a box as a proxy for a soundproof room. ...
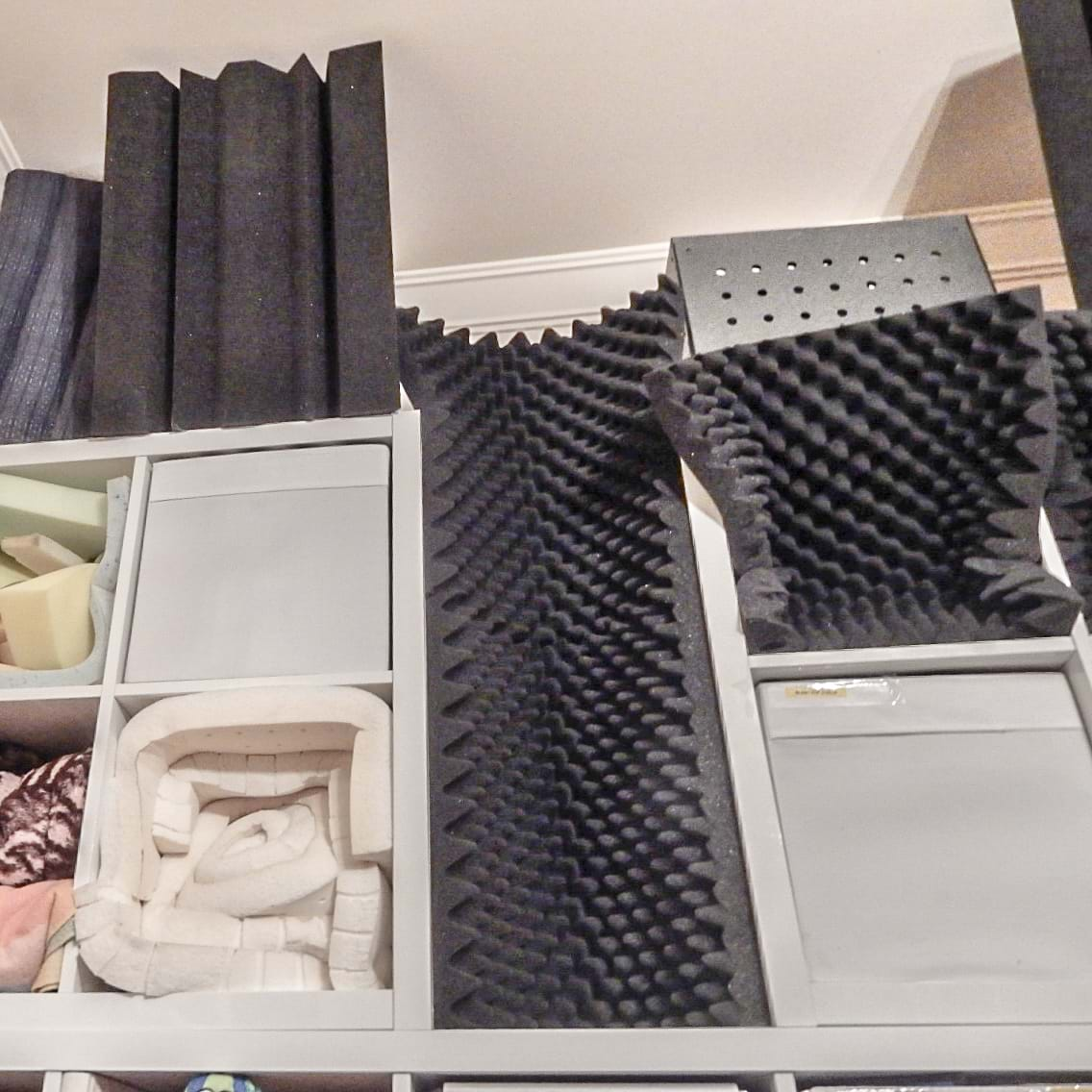
Dictionary.com. Lexico Publishing Group, LLC. Accessed December 19, 2005. (Source of some vocabulary definitions, with some adaptation.) http://www.dictionary.com
Contributors
Supporting program, acknowledgements.
The contents of this digital library curriculum were developed under grants from the Fund for the Improvement of Postsecondary Education (FIPSE), U.S. Department of Education and National Science Foundation (GK-12 grant no. 0338326). However, these contents do not necessarily represent the policies of the Department of Education or National Science Foundation, and you should not assume endorsement by the federal government.
Last modified: March 17, 2021
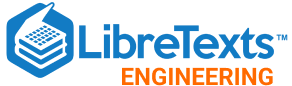
- school Campus Bookshelves
- menu_book Bookshelves
- perm_media Learning Objects
- login Login
- how_to_reg Request Instructor Account
- hub Instructor Commons
- Download Page (PDF)
- Download Full Book (PDF)
- Periodic Table
- Physics Constants
- Scientific Calculator
- Reference & Cite
- Tools expand_more
- Readability
selected template will load here
This action is not available.
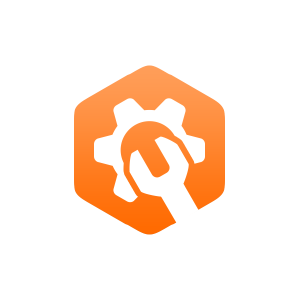
2.5: Thermodynamic tables
- Last updated
- Save as PDF
- Page ID 88830
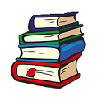
- Claire Yu Yan
- University of British Columbia, Okanagan via BC Campus
Thermodynamic tables are commonly used to determine the properties of a substance at a given state. This book includes t he tables for four pure substances : water, ammonia, R134a, and carbon dioxide. The data in these tables are obtained from NIST Chemistry WebBook , SRD 69 , which consists of the thermophysical properties of various common fluids.
Appendix A : Thermodynamic Properties of Water
- Table A1 : Saturated water
- Table A2 : Superheated vapour, water
- Table A3 : Compressed liquid water
Appendix B : Thermodynamic Properties of Ammonia
- Table B1 : Saturated ammonia
- Table B2 : Superheated ammonia
Appendix C : Thermodynamic Properties of R134a
- Table C1 : Saturated R134a
- Table C2 : Superheated R134a
Appendix D : Thermodynamic Properties of Carbon Dioxide
- Table D1 : Saturated CO 2
- Table D2 : Superheated CO 2
Tables A1, B1, C1, and D1 are the tables for the saturated fluids. They are used to find the properties of the corresponding fluid s in saturated liquid, saturated vapour, and two-phase regions. Tables A2, B2, C2, and D2 are the superheated vapour tables for find ing the properties of the fluid s in the superheated vapour region. Table A3 is the compressed liquid table for water.

The tables in Appendices A-D are presented with a small temperature increment . L inear interpolations, see Figure 2.4.4 , are often used if the given temperature or other properties cannot be found directly from these tables.
\[y=y_0+\left( x-x_0 \right) \dfrac{y_1-y_0}{x_1-x_0}\]

The following examples demonstrate how to use these tables to find the properties of a compressed liquid, superheated vapour, and liquid-vapour mixture.
Example \(\PageIndex{1}\)
Determine the properties of water at T = 1 5 0 o C and P = 100 kPa.
- Both temperature and pressure are given for water. Use the flow chart for case 1, Figure 2.4.1 .
- From Table A1 : at T = 150 o C , P sa t = 0.47617 MPa = 476.17 kPa.
- Because P =100 kPa < 476.17 kPa , or P < P sat , water at this state is a superheated vapour .
- From Table A2 : at T = 150 o C and P =100 kPa ,
v = 1.93665 m 3 /kg, u = 2582.94 kJ/kg
h = 2776.60 kJ/kg, s = 7.6148 kJ/kgK
Example \(\PageIndex{2}\)
Determine the properties of a mmonia at T = 0 o C and v = 0.2 m 3 /kg .
- Both T and v are given for ammonia . Use the flow chart for case 3, Figure 2.4.3 .
- From Table B1 : at T = 0 o C , v f = 0.001566 m 3 /kg and v g = 0.289297 m 3 /kg.
- Because v f < v < v g , ammonia at this state is a liquid- vapour two – phase mixture. Its pressure and quality are
\[P=P_{sat}=0.42939 \ \rm{MPa} =429.39 \ \rm{kPa}\]
\[x=\dfrac{v-v_f}{v_g-v_f} =\dfrac{0.2-0.001566}{0.289297-0.001566}=0.68965\]
From Table B1 : at T = 0 o C ,
u f = 342.48 kJ/kg and u g = 1481.17 kJ/kg
h f = 343.16 kJ/kg and h g = 1605.39 kJ/kg
s f = 1.4716 kJ/kgK and s g = 6.0926 kJ/kgK
T herefore, the specific internal energy, specific enthalpy, and specific entropy of this two-phase mixture are
Example \(\PageIndex{3}\)
Refrigerant R134a has a specific enthalpy h = 420 kJ/kg at T = 2 0 o C . Determine the pressure P and specific volume v of R134a at this state.
- Refer to case 4 as both T and h are given for R134a . B ecause the p rocedures for cases 3 and 4 are the same, the flow chart for case 3, Figure 2.4.3 , is used by replacing v with h .
- From Table C1 : a t T = 2 0 o C , h g = 409.75 kJ/kg. Because h = 420 kJ/kg > h g , R134a at this state is a superheated vapour .
- From Table C2 :
A t T = 2 0 o C and P 1 = 1 0 0 kPa: h 1 = 420.31 kJ/kg, v 1 = 0.233731 m 3 /kg
A t T = 20 o C and P 2 = 150 kPa: h 2 = 419.33 kJ/kg, v 2 = 0.154053 m 3 /kg
Because 419.33 kJ/kg < 420 kJ/kg < 420.31 kJ/kg, t he pressure of R134a at the given state must be between 100 kPa and 150 kPa. Use linear interpolation to calculate the pressure and specific volume at the given state .
\[\because\dfrac{P-P_1}{P_2-P_1} =\dfrac{h-h_1}{h_2-h_1}\]
\[\therefore\dfrac{P-100}{150-100} =\dfrac{420-420.31}{419.33-420.31}\]
\[\therefore P = 115.82 \ \rm{kPa}\]
Specific volume
\[\because\dfrac{v-v_1}{v_2-v_1} =\dfrac{h-h_1}{h_2-h_1}\]
\[\therefore\dfrac{v-0.233731}{0.154053-0.233731} =\dfrac{420-420.31}{419.33-420.31}\]

Query \(\PageIndex{1}\)
Media Attributions
- Linear interpolation © ElectroKid is licensed under a Public Domain license
Freezing Points of Various Liquids
Most recent answer: 10/22/2007
(published on 10/22/2007)
Follow-Up #1: detective work on freezing a slurpee
Those BK Icee's have a lot of sugar, but it's still less than 100gm/liter. That liter incudes lots of ice too though, so I don't know how high the concentration is in the syrup. As you say, we don't know whether what was left was pure syrup or had some melted ice in it. Also, I don't know if the sugar is mostly sucrose or fructose, which has about half the molecular weight and thus does a better job per gram of lowering the freezing point. High fructose corn syrup is very highly concentrated (only about 1/4 water) and must have a very low freezing point, but I haven't been able to find data on that. So I'm not sure if lowering the freezing point to 18°F with sucrose and fructose is even possible. However, there can be other complications. How cold did it actually get inside the car? Could the slurpee have stayed a little supercooled? Could enough water have evaporated while the car was still warm to increase the sugar concentration significantly?
So maybe an experiment would be the best way to answer your question. You and your granddaughter can take some of the same type of slurpee and get some liquid from it, making sure that its red color density matches the suspected drink. (The red dye and the sugar concentrations should track each other, since neither evaporates or goes into ice.) Leave it out in the same car under the same conditions and see what happens. In case it does freeze, you should try several repeats with approximately similar conditions, since in this country we have the "innocent until proven guilty" principle. (In some other countries, adolescents are legally allowed to drink.)
Regardless of the truth or falsity of the particular accusation you're making, maybe the exercise of doing a real experiment together will be valuable.
(published on 01/02/2016)
Follow-up on this answer
Related Questions
- freezing vs. melting points
- melting times
- melting of ice with salt
- imploded water bottles
- high-temperature freezing
- tears melting snow
- salt and freezing point
- salt water freezing temperature
- types of salt melting ice
- desalination by freezing
Still Curious?
Expore Q&As in related categories
- Melting & Freezing
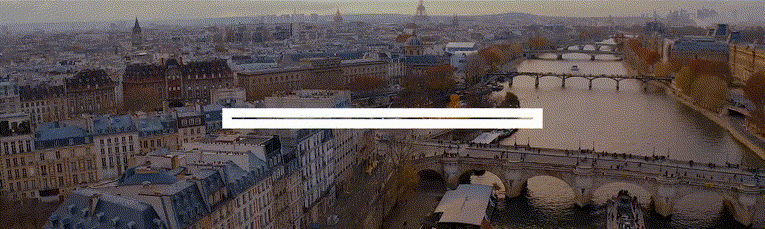
IMAGES
VIDEO
COMMENTS
Before doing the activity, predict which liquid (water, syrup, honey, and cooking oil) takes the least amount of time to reach the tray. Which liquid will take the most amount of time? 2. Place the cone marked A, B, C, and D on the tray. 3. Pour water on the top of cone A. Record the time it takes for the water to reach the tray. 4.
The gradient of the ramp is the same for each fluid. Method. You can either do one at a time - this is easier for recording time, or tip them all at the same time if you want them to get to the bottom of the table. Let them flow for the set time you have decided, or time them until they all reach the bottom. You can record your results in a ...
Pour water on the top of cone A. Record the time it takes for the water to reach the tray. Do it three times for each material. NOTE: Use the same amount of material each time. Table 2. Travel time of some liquids Liquid Travel time (seconds) 1st 2nd 3rd Average Water Cooking oil Syrup Honey. Q1. Is your prediction correct?_____
A unique property of liquids is something called viscosity. Viscosity is a liquid's resistance to flowing. Viscosity depends on the size and shape of the particles that make the liquid, as well as the attraction between the particles. Liquids that have a LOW viscosity flow quickly (ie. water, rubbing alcohol, and vegetable oil).
Hold one marble in each hand, just above the surface of the liquid in each glass. Watch the glasses closely. Be prepared to watch the bottom to see which marble hits first. If you have a volunteer, have them look at the glasses too. Let the marbles go <i>at the same time</i>. Observe which marble hits the bottom of the glass first.
Put 100 mL syrup in a clear glass. Using a drinking straw, blow some air from the bottom of the liquid. Observe. Repeat procedure 7 by blowing harder on itthe liquid. Observe. Q6. Compare the movement of the liquid as the bubbles move on the surface. In activity 3, you have observed that different liquids have different viscosities.
Knowing the time it took to travel through the column of liquid, the height of the column, the density of the sphere, and the density of the liquid, you can then calculate the viscosity of the liquid with the viscosity equation: Equation 1: μ = 2(Δρ)ga2 9v μ = 2 ( Δ ρ) g a 2 9 v. μ (the lowercase Greek letter mu, pronounced "mew") is the ...
4. Transfer spoonful of liquid onto the top of the run. Time how long it takes for the liquid to travel between the start and finish lines on the ramp. The timer should be started when the liquid first passes the start line and stopped when it first reaches the finish line). 5. Repeat with a variety of different liquids.
4 identical paper clips. 1 small bowl with water. 1 small bowl with Karo syrup. Advance preparation: Prepare the bottles for each group by filling six bottles with equal amounts of water (about two cups) and six bottles with equal amounts of Karo syrup (about two cups). Place the objects (screw, nail and paper clip) in bottles so that there is ...
Overview. Learners will observe and record what happens when they manipulate bottles containing one liquid and an object. They will compare bottles that have an object and different liquids. They will observe and record what happens when they manipulate bottles containing one liquid and more than one object. Go to this resource ». Concepts.
Learn more about using the scientific method for kids. STEP 2: Place your ruler on top of your glasses and then place the marbles on top. STEP 3: Tip your ruler toward you to release all of the marbles into your glasses at the exact same time. STEP 4: Watch closely to see which marble reaches the bottom of the glass first.
report flag outlined. Viscosity is a parameter wherein it determines the energy and time acquired to make a liquid flow. Just like the case of friction that occurs between two moving solid objects, Viscosity is the friction or an interaction between molecules between liquid and it is defined as the resistance of liquid to flow. Advertisement.
Surface tensions of several liquids are presented in Table 2. Among common liquids, water exhibits a distinctly high surface tension due to strong hydrogen bonding between its molecules. As a result of this high surface tension, the surface of water represents a relatively "tough skin" that can withstand considerable force without breaking.
There are some properties that all liquids, including water, have. All liquids have a certain portion of particles with enough energy to enter the gas phase, and if these particles are at the surface of the liquid, they do so (Figure 10.4.1 10.4. 1 ). The formation of a gas from a liquid at temperatures below the boiling point is called ...
Liquids Rule. You are allowed to bring a quart-sized bag of liquids, aerosols, gels, creams and pastes in your carry-on bag and through the checkpoint. These are limited to travel-sized containers that are 3.4 ounces (100 milliliters) or less per item. Placing these items in the small bag and separating from your carry-on baggage facilitates ...
23 people found it helpful. mmceebi. report flag outlined. The travel time of some liquids may differ from its flow. So for example, slime that has a very thick substance it means it is very slow to flow and water that has a thin substance that is very quick to flow. To identify the resistance of liquid, we use the term Viscosity. Advertisement.
Electromagnetic waves can travel through a vacuum at the speed of light, v = c = 2.99792458 x 10 8 m/s. For example, light from distant stars travels through the vacuum of space and reaches Earth. Electromagnetic waves have some characteristics that are similar to mechanical waves; they are covered in more detail in Electromagnetic Waves.
The viscosity of a liquid is a measure of its resistance to flow. Water, gasoline, and other liquids that flow freely have a low viscosity. Honey, syrup, motor oil, and other liquids that do not flow freely, like those shown in Figure 10.2.1 10.2. 1, have higher viscosities. We can measure viscosity by measuring the rate at which a metal ball ...
Project in science
14.5: Vapor Pressure. Page ID. Jim Clark. Truro School in Cornwall. This page looks at how the equilibrium between a liquid (or a solid) and its vapor leads to the idea of a saturated vapor pressure. It also looks at how saturated vapor pressure varies with temperature, and the relationship between saturated vapor pressure and boiling point.
Students explore how sound waves move through liquids, solids and gases in a series of simple sound energy experiments. Understanding the properties of sound and how sound waves travel helps engineers determine the best room shape and construction materials when designing sound recording studios, classrooms, libraries, concert halls and theatres.
Tables A2, B2, C2, and D2 are the superheated vapour tables for find ing the properties of the fluid s in the superheated vapour region. Table A3 is the compressed liquid table for water. In these tables, the specific volume, specific internal energy, specific enthalpy, and specific entropy are tabulated as functions of the pressure and ...
Butane liquifies at -0.5 °C and freezes at -138 °C (it liquifies at higher temperatures when it's under pressure). Most substances have solid, liquid, and gas phases under different temperature and pressure conditions. Some, like oil and glass, don't have clear demarcations between solid and liquid phases.